Abstract
Objectives
To determine the shear bond strength to bovine dentin of dual-cured resin cements cured in different circumstances, the contraction stress and volumetric shrinkage in both polymerization modes, and to review the failure stress distribution at the cement–tooth interface with finite element analysis.
Methods
The volumetric shrinkage of RelyX Unicem, Panavia F 2.0 and DC Core Automix was determined by mercury dilatometry. Polymerization contraction stress was determined using a constraint tensilometer set-up. For the shear bond strength test, cement discs on bovine root dentin (self-cured and dual-cured), composite discs cemented to dentin (self-cured and dual-cured), and dentin cemented to dentin (self-cured) specimens were fabricated. Specimens were stored in water for 24 h (37 °C, 100% humidity) and tested (crosshead speed 1 mm min −1 ). FE modeling of the specimens was carried out in order to calculate the maximum shear stresses in the cement–dentin interface. Differences between groups were determined using two-way ANOVA with Tukey post hoc tests, and paired samples t -tests ( α < 0.05).
Results
Panavia F2.0 showed significantly lower volumetric shrinkage than the other cements. Dual-curing lead to higher contraction stresses for all tested cements compared to self-curing. RelyX Unicem showed higher volumetric shrinkage when dual-cured. Shear bond strength and maximum shear stress was positively influenced by dual-curing. DC Core Automix performed best and Panavia F2.0 worst in terms of shear bond strength and maximum shear stress.
Significance
Curing mode may play an important role in the final bond strength to dentin of indirect restorations, depending on the material used.
1
Introduction
The retention and clinical success of indirect restorations relies heavily on the bond strength the cement will achieve and maintain to the substrates it is bonded to . The cements that are most used today are dual-cured resin cements. Its bond quality may depend on many variables, like the mode of polymerization . An important advantage of dual-cured cements is their ability to be light-cured to shorten treatment time, whilst a proper cure is secured in places where the light is not likely to reach the cement, for instance beneath an inlay, opaque crown materials like zirconium-dioxide reinforced ceramic crowns, or in deeper aspects of a root canal or post space. Nevertheless, previous research shows that sufficient curing in these places is not always obvious. Contradictory results are published. On one hand, light-curing is observed to be less effective in deep aspects of post spaces, resulting in a lower degree of conversion which might be compensated for by the dual-cure mode as is reported by Pereira who did not find differences in degree of conversion for a dual-cured resin cement in different root canal regions . On the other hand, lower cohesive strength , and depending on the cement, a lower diametral tensile strength within apical parts of the post space, even for dual-cured cements were reported. The light-transmitting ability of the post or restorative material plays a role together with the distance from the light source .
An important problem with resin cements is their volumetric shrinkage. This is caused by the cross-linking of monomers to polymer chains which results in volume loss of the cement. In an unconstraint situation this volume loss is compensated for by viscous flow of the cement . In a constraint condition however, when the cement is adhesively bonded to one or more walls, the flow of the cement is hindered, and the volume loss of the cement results in internal stresses known as contraction stress . The amount of stress can be predicted by calculating the Configuration factor or C-factor which has been described by Feilzer et al. as the ratio between bonded and unbonded surface of a cavity . With a high C-factor, the flow of the resin cement to compensate for volume loss as a result of polymerization shrinkage of the cement is very limited. Therefore, high stresses are generated. This can negatively influence the bond strength between cement and dentin, and even disrupt this bond. The higher the C-factor, the greater this problem will be , although this effect can be counteracted by the compliance of the cavity walls . Clinically, these effects are encountered with the cementation of resin bonded (indirect) restorations such as (prefabricated fiber) posts, (partial) crowns, inlays and onlays. However, the contraction stresses involved in the cementation of an indirect restoration are not solely a result of the C-factor. The mode of polymerization also plays an important role. Light curing a resin cement leads to a fast polymerization process, limiting the time for viscous flow of the cement, and thereby increasing the contraction stresses . Self-curing of a dual-cure cement, however, often leads to lower degrees of cure and therefore lower mechanical properties . Also, it has been shown that thin resin layers have higher tensile strengths compared to thicker resin layers . This is likely to be of influence.
The aim of the study was to gain a better understanding of the variables curing mode, volumetric shrinkage, polymerization contraction and C-factor on the shear bond strength to bovine dentin and the maximum shear stresses in the cement–dentin interface as calculated with Finite Element Analysis (FEA).
2
Materials and methods
In this study, the shear bond strength of RelyX Unicem, Panavia F 2.0, and DC Core Automix ( Table 1 ) to bovine dentin was determined under different circumstances. The cements were either self-cured (SC) or dual-cured (DC) and placed in bulk on a bovine dentin disc (DC specimens), as a cement layer between a bovine dentin disc and a composite disc (DCC specimens), or in between two bovine root dentin layers (DCD specimens) ( Table 2 and Fig. 1 ). The specimens were prepared by removing the crowns of bovine teeth leaving the roots which were ground flat and embedded in resin (PMMA Self Curing Clear, Vertex, NL) to create dentin discs. The discs were polished wet with 600 grit sanding paper (silicon grinding paper, Carbimet, Buehler, USA) to obtain a standardized smear layer, and stored at room temperature at 100% humidity. All cements were applied according to the manufacturers’ instructions, and in the case of the dual-cured groups, light-cured with a Elipar Freelight (3 M Espe, Seefeld, Germany). Each cement was tested with each test set-up ( n = 10). In the DC–SC and DC–DC groups, the cements were applied in a rubber mold with a diameter of 5 mm and a height of 1 mm. Before curing of the cement, the cement was covered with a plastic matrix strip. In the DCC–SC and DCC–DC groups, a small amount of cement was applied and a composite (Filtek Supreme XT, 3M-ESPE, Seefeld, Germany) disc with a diameter of 5 mm and a height of 1 mm was cemented to the dentin using finger pressure (ca. 50 N). In the DCD–SC groups, two dentin discs were cemented together. In order to obtain a standardized bonding surface, a perforated (5 mm diameter) transparent matrix band was applied to the surface of one of the dentin discs as they were cemented together.
Product | Manufacturer | Batch number | Composition |
---|---|---|---|
Clearfil DC Core Automix Paste | Kuraray | 0123AA | Catalyst paste: Bis-GMA, TEGDMA, silanated colloidal silica, barium glass, d,l -camphorquinone, benzoyl peroxide Universal paste: Bis-GMA, TEGDMA, silanated colloidal silica, barium glass, N,N-diethanol p-toluidine |
Clearfil SA Primer | Kuraray | 0066CA | Salicylic acid monomer |
Clearfil Photo Bond Bonding Agent Universal | Kuraray | 0536AA | N,N-di-ethanol p-toluidine, sodium benzene sulfinate, ethanol |
Clearfil Photo Bond Bonding Agent Catalyst | Kuraray | 0439AA | MDP, Bis-GMA, HEMA, hydrophobic alifatic dimethacrylate, camphorquinone, benzoyl peroxide |
RelyX Unicem Aplicap | 3M ESPE | 384961 | Powder: glass fillers, silica, calcium hydroxide, self-cure initiators, pigments, light-cure initiators. Liquid: methacrylated phosphoric esters, dimethacrylates, acetate, stabilizers, self-cure initiators |
Panavia F 2.0 Paste A | Kuraray | 0431AB | Hydrophobic aromatic dimethacrylate, hydrophobic aliphatic dimethacrylate, hydrophilic dimethacrylate, sodium aromatic sulfinate (TPBSS), N,N-diethanol-p-toluidine, surface treated (functionalized) sodium fluoride, silanated barium glass |
Panavia F 2.0 Paste B | Kuraray | 0220AB | MDP, hydrophobic aromatic dimethacrylate, hydrophobic aliphatic dimethacrylate, hydrophilic dimethacrylate, silanated silica, photoinitiator, dibenzoylperoxide |
Panavia F 2.0 ED Primer II Liquid A | Kuraray | 0280CA | HEMA, MDP, 5-NMSA, water, accelerator |
Panavia F 2.0 ED Primer II Liquid B | Kuraray | 0156AA | 5-NMSA, accelerator, water, sodium benzene sulfinate |
Panavia F 2.0 Oxyguard II | Kuraray | 0618BA | Polyethyleneglycol, glycerine, sodium-benzenesulfinate, N,N-diethanol p-toluidine |
Filtek Supreme XT restorative composite | 3M ESPE | Multiple | Non-agglomerated/non-aggregated 75 nm silica nanofiller, non-agglomerated/non-aggregated 15–20 nm zirconia nanofiller, loosely bound agglomerated zirconia/silica nanocluster consisting of 5–20 nm primary zirconia/silica particles, Bis-GMA, TEGDMA, Bis-EMA |
Group | Specimen | Curing mode |
---|---|---|
DC–SC | Dentin – Cement | Self-cure |
DC–DC | Dentin – Cement | Dual-cure |
DCC–SC | Dentin – Cement – Composite | Self-cure |
DCC–DC | Dentin – Cement – Composite | Dual-cure |
DCD–SC | Dentin – Cement – Dentin | Self-cure |
All specimens were stored at 37 °C for 24 h in the dark before testing. After this, the rubber molds were removed from the specimens, and the specimens were placed in the testing device for shear testing until failure. The testing was performed with the Instron 6022 Tensilometer (Instron Ltd., Wycombe, UK) at a crosshead speed of 1 mm min −1 . The load at failure was recorded, and the shear strength was calculated. The mode of failure, adhesive, cohesive or mixed, was determined by visual inspection.
In addition to the shear bond strength measurements, the volumetric shrinkage and polymerization contraction stress were determined for the same cements. Volumetric shrinkage measurements were performed with the use of a mercury dilatometer at 23 ± 0.1 °C as reported by de Gee et al. . The specimens ( n = 6) were either dual-cured using an Elipar Trilight (3 M ESPE, Seefeld, Germany) for 40 s in a standard mode, or left to self-cure. The measuring continued for 30 min.
Polymerization contraction stress measurements were carried out using a constraint tensilometer set-up as described by Kleverlaan and Feilzer . The specimens ( n = 6) were either light-cured (3M ESPE Elipar Trilight) for 40 s or self-cured. During a period of 30 min, the contraction stress development was measured, while the distance between the glass and steel bolt head was kept constant through counteracting feedback displacement of the cross-head. This simulated a restoration in a fully rigid situation, where the cavity walls do not yield to the contraction forces.
2.1
Finite element analysis
Three-dimensional FE models of the three different test specimens DC, DCC, and DCD were created. The finite element modeling was carried out using FEMAP software (FEMAP 10.1.1; Siemens PLM Software, Plano, TX, USA), while the analysis was done with NX Nastran software (NX Nastran; Siemens PLM Software, Plano, TX, USA). The models were designed according to the dimensions of the test specimens of the shear bond strength test. The cement layer was designed with a thickness of 50 μm. Since the models were symmetrical in geometry they were split in half specimens, with the nodes in the centric plane allowing for sliding in the surface only. The models were composed of 22,508–39,491 parabolic tetrahedron solid elements. For the resin cements an elastic modulus of 7.5 GPa was used. For the resin composite material and the dentin, values of 10.5 GPa and 18.3 GPa were used, respectively. The Poisson’s ratio was 0.3 in all cases. The anisotropy of the dentin was not taken into account.
The experimentally determined loads from the shear bond strength tests were applied on the nodes at the outside central area of the dentin disc. In order to simulate the placement and the support in the test set-up of the specimen, the nodes in the area, where the force was applied and the nodes at the outside plane of the loaded dentin disc were fixed with no movement in the direction perpendicular to the force and the nodes of the elements at the opposite outside central area of the other disc were fixed with no movement in any direction ( Fig. 2 ).
The analysis was carried out with the load as primary input followed by a second calculation taking into account the shrinkage of the cement layer.
The obtained values of the volumetric shrinkage and the polymerization contraction stresses ( Table 3 ) were used to calculate the constraint shrinkage. In an FE model simulating the used tensilometer set-up, the contraction stresses ( σ FEA ) were calculated using the values of the shrinkage determined by the dilatometer. As described by Ausiello et al. , the contraction stresses found in the test set-up ( σ TEST ) were considered to be caused by the constraint portion of the shrinkage, the remaining shrinkage being free shrinkage. The constraint shrinkage was calculated as the σ TEST/ σ FEA portion of the total shrinkage.
RelyX Unicem | Panavia F 2.0 | DC Core Automix | |
---|---|---|---|
A | |||
DC–SC | 5.7 (3.4) AB | 6.4 (2.6) | 7.9 (1.9) ABC |
DC–DC | 10.8 (3.7) A | 7.1 (1.5) a | 13.0 (3.1) Aa |
DCC–SC | 7.6 (1.7) C | 9.3 (3.0) | 10.8 (2.4) |
DCC–DC | 15.4 (8.2) BCDa | 9.3 (3.8) ab | 13.4 (6.1) Bb |
DCD–SC | 9.9 (4.2) Da | 7.2 (1.7) b | 14.4 (3.5) Cab |
Overall cement | 9.8 (5.6) a | 7.9 (2.8) a | 11.9 (4.3) a |
B | |||
DC–SC | 90 | 100 | 100 |
DC–DC | 60 | 90 | 80 |
DCC–SC | 90 | 100 | 80 |
DCC–DC | 70 | 100 | 90 |
DCD–SC | 100 | 70 | 100 |
Overall cement | 82 | 92 | 90 |
C | |||
DC–SC | 14.5 (8.6) AB | 16.5 (5.8) | 20.0 (4.9) |
DC–DC | 28.0 (9.5) ACDa | 19.0 (4.1) ab | 28.0 (6.7) b |
DCC–SC | 16.0 (3.5) CE | 17.5 (5.7) | 20.0 (4.5) |
DCC–DC | 28.5 (15.2) BEFa | 18.5 (7.6) a | 26.0 (11.9) |
DCD–SC | 15.0 (6.3) DF | 11.5 (2.8) a | 22.0 (5.4) a |
Overall cement | 20.4 (11.2) a | 16.6 (5.9) ab | 23.2 (7.6) b |
This calculated constraint shrinkage was used as shrinkage to calculate the stresses due to the shrinkage of the cement layer in the models of the test specimens. Hereafter, the combined stresses of the stresses due to the loads and the shrinkage of the cement layer were calculated using the linear combination facility of FEMAP. The maximum shear stresses at the cement–dentin interface in the horizontal plane were reported.
2.2
Statistical analysis
Two-way ANOVA and Tukey’s post hoc tests (SPSS 15.0, SPSS Inc., Chicago, MN, USA) were used to analyze the 30 min results of the volumetric shrinkage and contraction stress tests, as well as the differences between groups of the shear bond strength test, and the maximum shear stresses as obtained with FEA. The volumetric shrinkage and contraction stress data measured at 5, 15, and 30 min, respectively, were analyzed with paired t -tests. A P -value < 0.05 was considered statistically significant.
2
Materials and methods
In this study, the shear bond strength of RelyX Unicem, Panavia F 2.0, and DC Core Automix ( Table 1 ) to bovine dentin was determined under different circumstances. The cements were either self-cured (SC) or dual-cured (DC) and placed in bulk on a bovine dentin disc (DC specimens), as a cement layer between a bovine dentin disc and a composite disc (DCC specimens), or in between two bovine root dentin layers (DCD specimens) ( Table 2 and Fig. 1 ). The specimens were prepared by removing the crowns of bovine teeth leaving the roots which were ground flat and embedded in resin (PMMA Self Curing Clear, Vertex, NL) to create dentin discs. The discs were polished wet with 600 grit sanding paper (silicon grinding paper, Carbimet, Buehler, USA) to obtain a standardized smear layer, and stored at room temperature at 100% humidity. All cements were applied according to the manufacturers’ instructions, and in the case of the dual-cured groups, light-cured with a Elipar Freelight (3 M Espe, Seefeld, Germany). Each cement was tested with each test set-up ( n = 10). In the DC–SC and DC–DC groups, the cements were applied in a rubber mold with a diameter of 5 mm and a height of 1 mm. Before curing of the cement, the cement was covered with a plastic matrix strip. In the DCC–SC and DCC–DC groups, a small amount of cement was applied and a composite (Filtek Supreme XT, 3M-ESPE, Seefeld, Germany) disc with a diameter of 5 mm and a height of 1 mm was cemented to the dentin using finger pressure (ca. 50 N). In the DCD–SC groups, two dentin discs were cemented together. In order to obtain a standardized bonding surface, a perforated (5 mm diameter) transparent matrix band was applied to the surface of one of the dentin discs as they were cemented together.
Product | Manufacturer | Batch number | Composition |
---|---|---|---|
Clearfil DC Core Automix Paste | Kuraray | 0123AA | Catalyst paste: Bis-GMA, TEGDMA, silanated colloidal silica, barium glass, d,l -camphorquinone, benzoyl peroxide Universal paste: Bis-GMA, TEGDMA, silanated colloidal silica, barium glass, N,N-diethanol p-toluidine |
Clearfil SA Primer | Kuraray | 0066CA | Salicylic acid monomer |
Clearfil Photo Bond Bonding Agent Universal | Kuraray | 0536AA | N,N-di-ethanol p-toluidine, sodium benzene sulfinate, ethanol |
Clearfil Photo Bond Bonding Agent Catalyst | Kuraray | 0439AA | MDP, Bis-GMA, HEMA, hydrophobic alifatic dimethacrylate, camphorquinone, benzoyl peroxide |
RelyX Unicem Aplicap | 3M ESPE | 384961 | Powder: glass fillers, silica, calcium hydroxide, self-cure initiators, pigments, light-cure initiators. Liquid: methacrylated phosphoric esters, dimethacrylates, acetate, stabilizers, self-cure initiators |
Panavia F 2.0 Paste A | Kuraray | 0431AB | Hydrophobic aromatic dimethacrylate, hydrophobic aliphatic dimethacrylate, hydrophilic dimethacrylate, sodium aromatic sulfinate (TPBSS), N,N-diethanol-p-toluidine, surface treated (functionalized) sodium fluoride, silanated barium glass |
Panavia F 2.0 Paste B | Kuraray | 0220AB | MDP, hydrophobic aromatic dimethacrylate, hydrophobic aliphatic dimethacrylate, hydrophilic dimethacrylate, silanated silica, photoinitiator, dibenzoylperoxide |
Panavia F 2.0 ED Primer II Liquid A | Kuraray | 0280CA | HEMA, MDP, 5-NMSA, water, accelerator |
Panavia F 2.0 ED Primer II Liquid B | Kuraray | 0156AA | 5-NMSA, accelerator, water, sodium benzene sulfinate |
Panavia F 2.0 Oxyguard II | Kuraray | 0618BA | Polyethyleneglycol, glycerine, sodium-benzenesulfinate, N,N-diethanol p-toluidine |
Filtek Supreme XT restorative composite | 3M ESPE | Multiple | Non-agglomerated/non-aggregated 75 nm silica nanofiller, non-agglomerated/non-aggregated 15–20 nm zirconia nanofiller, loosely bound agglomerated zirconia/silica nanocluster consisting of 5–20 nm primary zirconia/silica particles, Bis-GMA, TEGDMA, Bis-EMA |
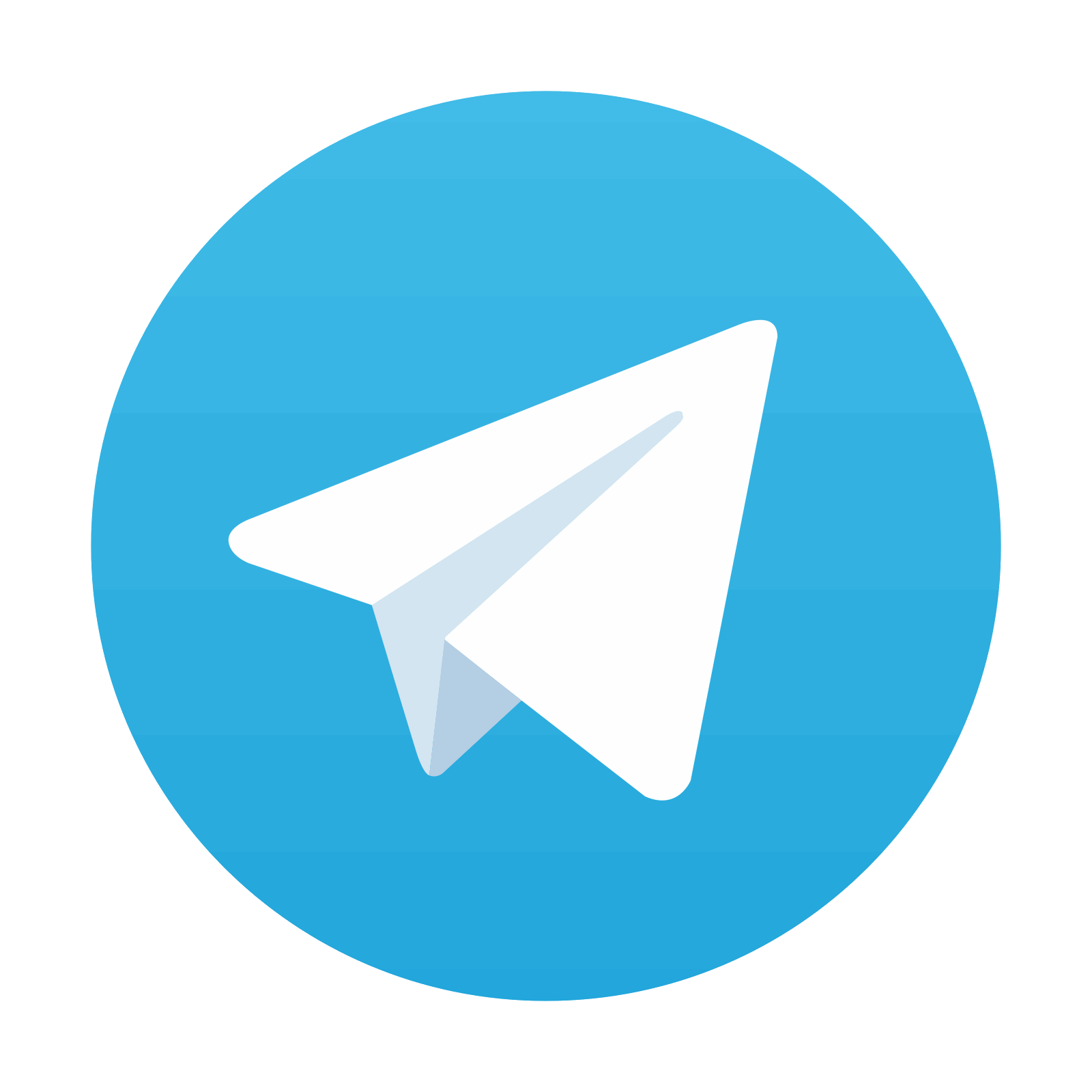
Stay updated, free dental videos. Join our Telegram channel

VIDEdental - Online dental courses
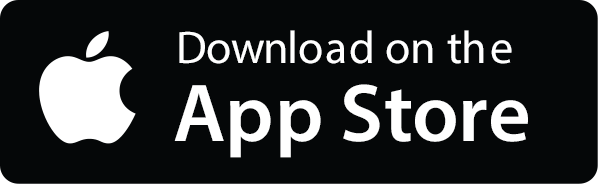
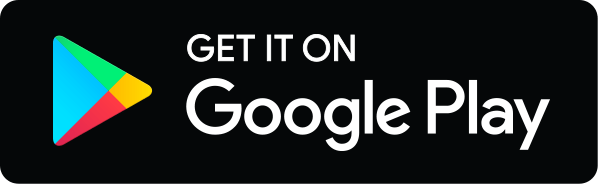
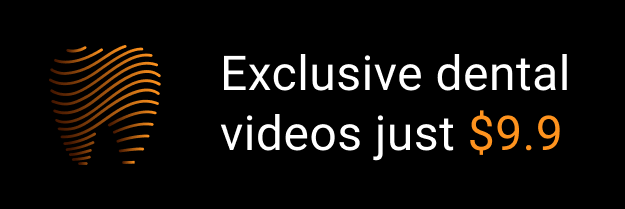