Fig. 15.1
Difference between revascularization and pulp regeneration in immature teeth. (a) The revitalization/revascularization procedures of filling of the canal space with blood clot. (b) The pulp regeneration procedures of filling of the canal space with stem cells with homing/migration factor
15.3 Ectopic Approach for Pulp Regeneration
The concept of pulp regeneration is distinct from revitalization/revascularization. The tissue engineering triad of stem/progenitor cells, morphogenetic signaling molecules, and extracellular scaffold is utilized for pulp regenerative therapy [4, 17] (Fig. 15.1a, b). The first evidence for de novo pulp regeneration has been demonstrated in ectopic tooth transplantation approach in which human tooth slices or root fragments were injected with stem cells/scaffolds into the root canal and were subsequently transplanted into the subcutaneous space of immunocompromised mice [18, 19] (Fig. 15.2a). The ectopic transplantation approach has advantages to neglect a direct blood supply essential for regeneration. It is a useful model to examine regeneration of pulp tissue and dentin by dental pulp/progenitor stem cells and its mechanisms (Fig. 15.3a, b). It may also allow for the study of molecular and cellular events involved in the fate of stem cell therapy [19]. Pulp-like tissue is induced in a tooth slice model (1-mm-thick tooth slice (cross sections)) by filling of pulp stem cells and dentin matrix protein 1 (DMP1) together [20] or stem cells from exfoliated deciduous teeth (SHED) only [21]. SHED can differentiate into functional odontoblast-like cells that generate tubular dentin along the dentinal wall and angiogenic endothelial cells in the tooth slice model [22]. Similarly pulp-like tissue is regenerated in subcutaneously transplanted tooth root fragment (6–7–mm long) with an enlarged root canal (2–3 mm in diameter) with one end sealed with mineral trioxide aggregate (MTA) cement. In this root fragment model, it is unlikely to occur shortly after transplantation that the blood vessel growth into the end of the canal space provides nutrients for the stem cells. Thus, the cells not only survive well but also regenerate tissue by the nutrients which are able to diffuse into the canal space [23]. PLG poly(DL-lactide-co-glycolide) scaffolds seeded with stem cells from apical papilla (SCAP) or DPSCs are injected into the root canal. Three to four months after transplantation, the canal space is filled with vascularized pulp-like tissue, and a newly generated dentin-like layer is deposited onto the existing dentinal walls and the MTA cement surface [23]. The regenerated dentin-like tissue has less continuity and thickness in DPSCs transplantation with PLG compared with that in SCAP transplantation with PLG [23]. More recently, full-length human roots injected with scaffolds (PuraMatrix™ (3-D Matrix Medical Technology, Waltham, MA) or recombinant collagen) containing SHED are transplanted subcutaneously and resulted in pulp-like tissue regeneration with odontoblasts capable of generating new tubular dentin throughout the root canals [24].
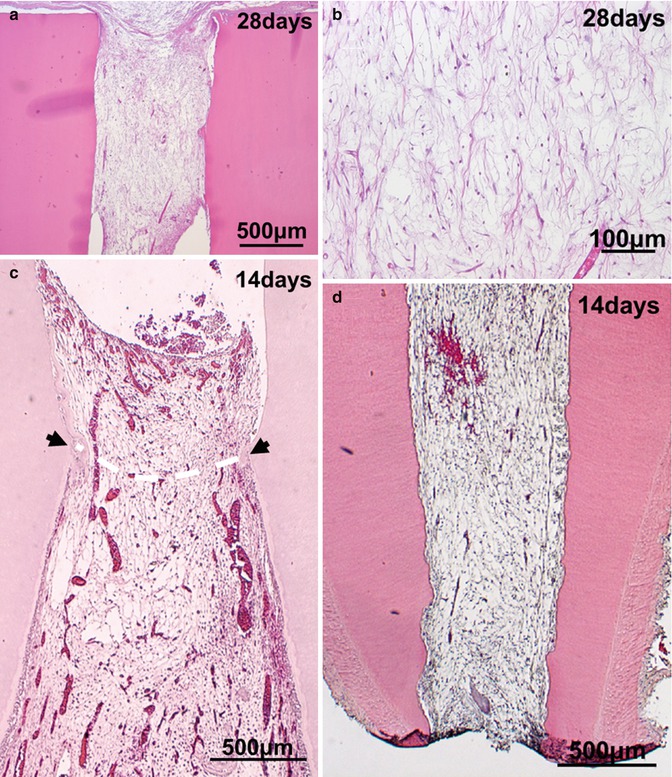
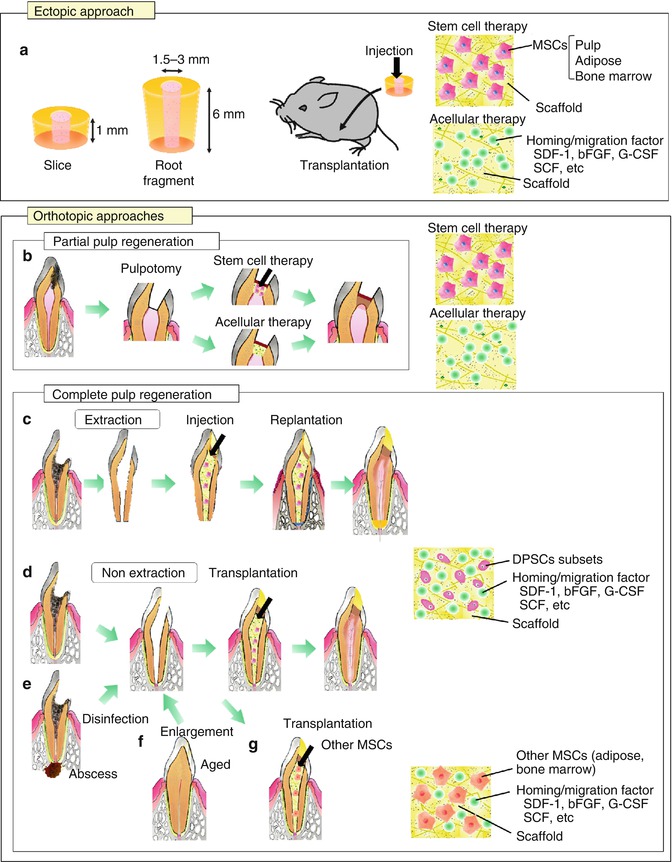
Fig. 15.2
Experimental in vivo approaches for pulp regeneration. (a) Ectopic approach. (b–g) Orthotopic approaches. (b) Partial pulp regeneration model. (c–g) Complete pulp regeneration model. c Tooth extraction model in case of pulpectomy. (d–g) Tooth non-extraction method in case of pulpectomy. (e) Complete pulp regeneration with periapical disease. (f) Complete pulp regeneration in the aged. (g) Complete pulp regeneration harnessing MSCs from other tissue sources
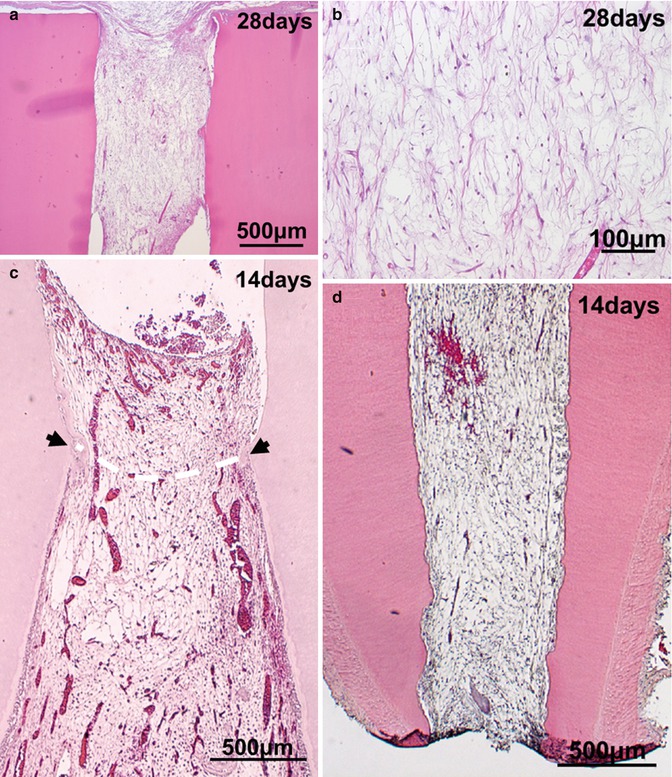
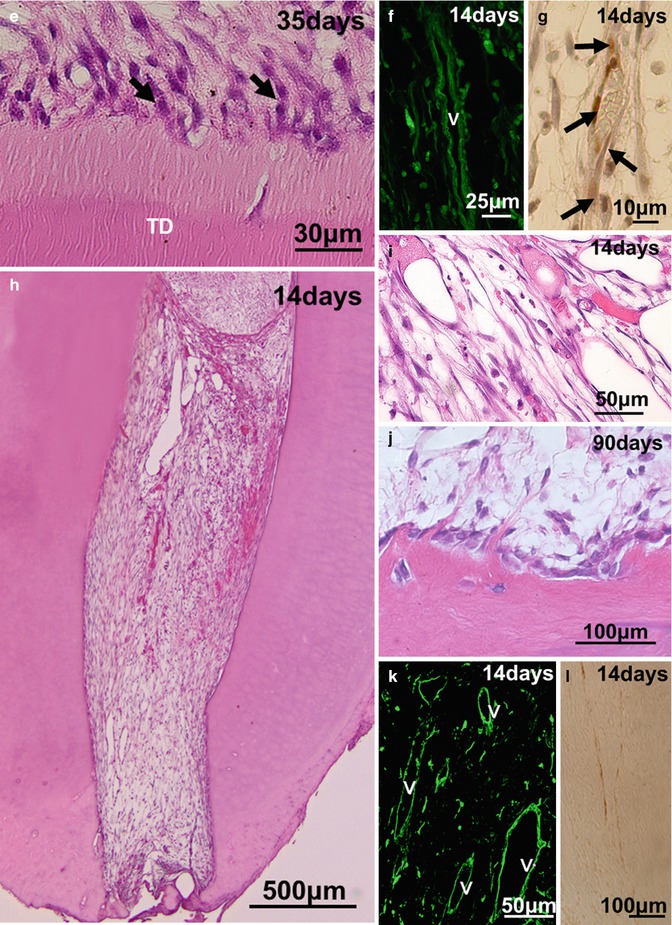
Fig. 15.3
Experimental in vivo approaches of pulp regeneration. (a, b) Ectopic pulp regeneration after transplantation of CD31−/CD146− SP cells with tooth root into subcutaneous sites of mouse. (c) Partial pulp regeneration in the cavity of the amputated pulp of dogs 14 days after autologous transplantation of CD31−/CD146− SP cells of a three-dimensional pellet with type I and type III collagen. (d–g) Complete regeneration of pulp-like tissue after autologous cell transplantation in the extraction model of dogs. (d) Fourteen days after CD31−CD146− SP cell transplantation with 10 ng/μl SDF-1. (e) Thirty-five days after CD105+ cell transplantation. Odontoblastic cells (arrows) lining to newly formed dentin. (f) Immunostaining with BS-1 lectin on day 14. v: Newly formed capillaries. (g) Immunostaining with PGP 9.5 on day 14. Neuronal process (arrows). (h–l) Complete regeneration of pulp-like tissue after autologous CD105+ cell transplantation with 10 ng/μl SDF-1 in the non-extraction model of dogs. Fourteen days after transplantation. (j) Ninety days after transplantation. Odontoblastic cells lining to newly formed dentin. (k) Immunostaining with BS-1 lectin on day 14. v: Newly formed capillaries. (l) Immunostaining with PGP 9.5 on day 14
15.4 Orthotopic Model for Pulp Regeneration in Mature Teeth
15.4.1 Pulp Stem/Progenitor Cells
Dental pulp tissue is rich in vasculature and innervation, and their intimate association is involved in pulp homeostasis including regulation of inflammation and enhancing pulp defense mechanisms [25]. Angiogenesis and reinnervation are also prerequisites for pulp regeneration to stimulate migration/homing, proliferation of stem cells, and stromal matrix formation in the root canal [4]. Thus, for optimal stem cell therapy, pulp stem/progenitor cells need to not only have high proliferative and multi-differentiation potential in vitro as typical stem cell properties but also to enhance angiogenesis/vasculogenesis and neurogenesis in vivo [5]. Subfractions of dental pulp stem cells (DPSCs) with high angiogenic/vasculogenic and neurogenic potential, pulp CD31− side population (SP) cells, and CD105/endoglin+ cells can be isolated from human, porcine, and canine adult teeth [5, 26–31]. Pulp SP cells are enriched in the “true” or “mother” adult stem cells, which exhibit lower level of the DNA-binding fluorescent dye, Hoechst 33342, than the rest of pulp cells [32]. CD105/endoglin is a component of the transforming growth factor-beta receptor complex which is widely expressed on mesenchymal stem cells (MSCs) [33] and has been used for isolation of MSCs [34, 35]. Pulp CD31− SP cells and CD105+ cells are positive for CD29, CD44, CD73, and Thy-1/CD90 and negative for CD31 and CD45 as unfractionated colony-derived DPSCs and other MSCs [36]. Their positive rates of CD105, CXCR4, and G-CSF receptor (G-CSFR), however, are higher compared with colony-derived DPSCs [29]. Pulp CD31− SP cells and CD105+ cells stimulate the blood flow recovery and angiogenesis/vasculogenesis after transplantation into mouse hindlimb ischemic models [5, 28, 30]. Those cells also enhance angiogenesis and neurogenesis in rat cerebral ischemic models in peri-infarct area by promoting migration and differentiation of the endogenous neuronal stem/progenitor cells, which resulted in the functional recovery [5, 30, 37]. The conditioned medium of DPSC subfractions contain high concentration of angiogenic/neurotrophic factors such as brain-derived neurotrophic factor (BDNF), nerve growth factor (NGF), vascular endothelial growth factor (VEGF-A), glial cell-line-derived neurotrophic factor (GDNF), granulocyte-macrophage colony-stimulating factor (GM-CSF), granulocyte colony-stimulating factor (G-CSF), and matrix metalloproteinase 3 (MMP3). The stimulatory role of these trophic factors on proliferation, migration, and anti-apoptosis has also been reported in vitro in endothelial cells, neuroprogenitor cells, and fibroblasts [28, 30, 37]. The transplanted DPSC subfractions are successfully engrafted in proximity to the newly formed vasculature and neuron, releasing angiogenic/neurotrophic factors without direct incorporation into the vessels or nerves, suggesting their trophic effect [28, 30, 37, 38] as reported in other MSCs [39–41]. These findings demonstrate cell sources of the DPSC subfractions, pulp CD31– SP cells, and CD105+ cells for stem cell therapy in pulp regeneration by promoting cell survival, angiogenesis, neurogenesis, and neuroprotection and by activating endogenous stem cells in a paracrine fashion.
15.4.2 Partial Pulp Regeneration
With the recent advances in tissue engineering and regenerative medicine, partial pulp regeneration therapy in case of pulp exposure or partial pulpitis has been developed (Fig. 15.2b). The two potential methods in partial pulp regeneration are (1) transplantation of engineered pulp/dentin tissue or DPSC subfractions with scaffold and (2) application of homing/migration factors with scaffolds.
It has been reported to engineer pulp-like tissue in vitro by harnessing pulp fibroblasts adhered to polyglycolic acid (PGA) scaffolds [7, 42, 43]. The pulp cells seeded in PGA for 24 h survive and produce extracellular matrix after subcutaneous transplantation in immunocompromised mice [42], although their engraftment and resultant partial pulp regeneration have not been demonstrated in any orthotopic models until recently. After that, the successful induction of the partial pulp regeneration as indicated in the first method has been demonstrated after autologous transplantation of three-dimensional culture of pulp CD31− SP cells with collagen scaffold in the cavity on the amputated pulp (Fig. 15.3c). The regenerated pulp tissue contains well-developed vasculature and innervation, and the tubular dentin formed along the dentinal wall [27]. As seen in the cerebral and hindlimb ischemic models, the transplanted CD31− SP cells localize closely to the newly formed capillaries with expression of angiogenic/neurotrophic factors, implicating their trophic effects on neovascularization also in partial pulp regeneration. In contrast with CD31− SP transplantation, CD31+ SP cell transplantation induces regenerated tissue in less volume with fewer capillaries [27], suggesting distinct regenerative potential for pulp tissue among subfractions of DPSCs.
The second method is to recruit endogenous progenitor cells by some homing/migration factor, subsequently participating in partial pulp regeneration. It is advantageous that the second method lacks excessive costs including cell isolation, handling, storage and shipping, ex vivo manipulation, and immune rejection (for allogeneic cells) and is also free from liabilities of potential contamination, pathogen transmission, and tumorigenesis that may be associated with cell transplantation [44]. Prominent migration/homing factors that have recently emerged as aids in regenerative medicine are fibroblast growth factor-2 (FGF-2) [45] and stromal cell-derived factor-1α (SDF-1, CXCL12/pre-B-cell growth-stimulating factor) [46]. SDF-1 is known as a chemokine for CXCR4-positive stem cells. When DPSCs seeded on the surface of three-dimensional collagen gel cylinders are incubated in chemically defined media supplemented with FGF-2 or SDF-1, more cells migrate into collagen gel than in the absence of these cytokines [47]. Re-cellularization and revascularization have been demonstrated in endodontically treated human teeth with FGF-2 followed by ectopic transplantation [47]. A noncontrolled release of free FGF-2 only accelerates reparative dentin formation in the residual dental pulp, whereas a controlled release of FGF-2 from gelatin hydrogels induces formation of osteodentin in the pulp proliferating in the dentin defects. The controlled release of an appropriate dosage of FGF-2 from gelatin hydrogels induced osteodentin formation on the surface of the regenerated pulp [48–50]. Another migration/homing factor related to the second method is matrix metalloproteinase-3 (MMP-3). MMP-3 has high proliferation, migration, and anti-apoptotic effects on endothelial cells in vitro and promotes angiogenesis and pulp wound healing when applied on the amputated pulp of rat incisors [51]. The pulp tissue is regenerated by anti-inflammatory effects of MMP-3 even in partial irreversible pulpitis in a canine experimental model [52]. Furthermore, the migration of DPSCs is also promoted by the extracellular matrix proteins (EMPs), particularly laminin, and chemoattractants, such as sphingosine-1-phosphate (S1P) and TGF-β1, suggesting the highly regulated migration by the interplay between the EMPs and chemoattractants [53]. More recently, stem cell factor (SCF) which binds to the c-Kit receptor CD117 is applied in pulp regeneration, resulting in induced cell homing and promoting angiogenesis, remodeling of the implanted collagen scaffold, and new collagen matrix synthesis [54]. Thus, these findings demonstrate that selective homing/migration factors, such as bFGF, SDF-1, MMP3, S1P, TGF-β, and SCF, are potentially useful to recruit endogenous dental pulp cells including stem/progenitor cells from the remaining pulp tissue in partial pulp regeneration.
15.4.3 Complete Pulp Regeneration with DPSC Subfractions and Homing/Migration Factors
The discovery of a new population of mesenchymal stem cells residing in the apical papilla (SCAPs) of the developing teeth with incomplete apical closure [55–57] has raised the possibility of whole pulp regeneration after pulpectomy in immature teeth (Fig. 15.1b). Furthermore, the existence of mesenchymal stem cells in the surrounding tissue of the teeth, bone marrow, and periodontal ligament (BMSCs and PDLSCs) [18] makes considerable optimism for whole pulp regeneration even in case of mature teeth with complete apical closure (Fig. 15.2c, d). The transplanted cells need nutrition and oxygen in the empty root canals and some DPSC subfractions with high migration potential including CD105+ cells and CD31– SP cells have potential utility. They induce angiogenesis and reinnervation and are not incorporated into the vasculature or nerve [5, 28]. Thus, homing/migration factors are potentially useful for whole pulp regeneration to recruit endogenous stem/progenitor cells into the pulpectomized root canal [5]. SDF-1 is one of the prominent homing/migration factors known to be involved in hematopoietic stem cells (HSC) homing to the bone marrow niche [58, 59] and in subventricular zone (SVZ) cells homing to areas of ischemic injury in central nervous system (CNS) [60–62]. MSCs highly express CXCR-4 on their surface, and CXCR-4-SDF-1 axis is used by MSCs in homing and engraftment at injured or inflammation sites [63]. The DPSC subfractions, pulp CD31– SP cells, and CD105+ cells are also CXCR4-positive with high migratory and proliferative activity with SDF-1 [5, 28]. A recent report on SDF-1 has demonstrated that GFP-labeled bone marrow-derived cells engraft more in the dental pulp than other tissues/organs after transplantation into irradiated wild-type mice under normal conditions, since dental pulp expresses SDF-1 significantly higher than other tissues/organs [64]. These results shed new light on the possibility of complete pulp regeneration by application of SDF-1 together with DPSC subfractions in the pulpectomized root canal. Combinatorial delivery of bFGF, VEGF, or PDGF with NGF and BMP7 has shown to promote cell migration into pulpectomized root canals of human teeth to induce revascularization and pulp regeneration after ectopic transplantation [44]. Furthermore, most recently, stem cell factor (SCF) which binds to the c-Kit receptor CD117 has shown to increase in the cell number and capillaries in subcutaneously implanted collagen sponge, suggesting the suitability of SCF as a potent aid in pulp regeneration [54]. Thus, other possible homing/migration factors might be bFGF, VEGF, PDGF, and SCF.
An optimal scaffold with the following properties has been developed for efficient and safe complete pulp regeneration: (1) optimal flow for injection to be devoid of bubble formation and little contraction after hardening in the root canal; (2) biocompatibility with high bioactivity to be impregnated with trophic factors secreted by transplanted stem cells for homing, engraftment, anti-apoptosis and proliferation of endogenous stem/progenitor cells; (3) biodegradability to release trophic factors and replace the damaged tissue by the regenerated tissue [65–67]; (4) close resemblance of the cell’s physiological environment, natural extracellular matrix (ECM), providing the chemical signals to modulate cellular behavior and reinforce a particular phenotype [65]; and (5) no stimulation of differentiation of odontoblasts/osteodentinoblasts and mineral deposition inside the root canal except along the dentinal wall and in the dentin defect [5]. The two categories of materials are natural polymers such as collagen, gelatin, dextran and fibronectin, and synthetic polymers such as poly(lactic) acid (PLA) and poly(glycolic) acid (PGA). These materials exhibit satisfactory results in terms of biocompatibility and degradation. Collagen is biocompatible and degradable by enzymes, but its processing and customizing for specific application are often difficult. It is also affiliated with the risk of transmitting animal-associated pathogens or provoking an immunoresponse [65]. In contrast, PLA and PGA and their copolymer are nontoxic and biocompatible and degrade by hydrolysis. They lack the morphogenetic cues that are physiologically present in the extracellular matrix [65]. Ectopic transplantation of SHED seeded onto PLA into tooth slice forms vascularized soft connective, pulp-like tissue [21, 22]. SCAP and DPSCs seeded onto PLGA into root canals also form pulp-like tissue [23]. A recent promising class of biomaterials, self-assembling peptide hydrogels, generate extracellular matrix-like materials with cell adhesion motifs, controlled release of bioactive molecules, enhanced cell migration, and enzyme-cleavable sites for cell-mediated degradation [68]. The combination of inductive scaffold with stem cells might optimize the approaches for pulp regeneration. Thus, based on the concepts described previously, complete pulp regeneration has been developed using the tissue engineering triad, stem/progenitor cells, homing/migration factors, and optimal scaffolds. To mimic the endodontic treatment, transplantation has been performed in mature teeth with complete apical closure into the alveolar bone in dogs. The first experimental model for complete pulp regeneration is a tooth extraction model in case of pulpectomy (Fig. 15.2c). The tooth is extracted, and its apical portion of the root (1 mm in length) is cut out. The apical foramen of the root canal is enlarged to 0.8 mm in width after whole pulp removal. Ex vivo injection of autologous DPSC subfractions, CD31– SP cells, or CD105+ cells, 1 × 106 cells, is performed with collagen types I and III (1:1) scaffold (collagen TE, Nitta Gelatin) in the lower part of the root canals. The upper part is further filled with SDF-1 at the final concentration of 10 ng/mL with collagen TE. And finally, the tooth is re-transplanted into the alveolar bone. Pulp-like tissue with well vasculature and nerves is formed 14 days after transplantation (Fig. 15.3d, f, g). The odontoblast-like cells extending their process into the dentin tubules and producing tubular dentin along the dentinal wall are seen on day 35 (Fig. 15.3e). The regenerated pulp-like tissue does not mineralize inside the root canal except along the dentinal wall and in dentin defect (Fig. 15.3d), unlike transplantation of unfractionated DPSCs which yields mineralized tissue in the entire regenerated tissue [69].
The second experimental model is tooth non-extraction method in case of pulpectomy. Pulpectomy with enlarged apical portion, 0.6~0.7 mm in width, is also performed without tooth extraction in mature teeth with complete apical closure in an experimental model in dogs (Fig. 15.2d). Autologous transplantation of DPSC subfractions, 1 × 106 cells with collagen TE in the lower part of the root canal and SDF-1 (15 ng/mL) with collagen TE in the upper part, is performed [26]. Furthermore, the modified non-extraction method in which DPSC subfractions and SDF-1 are injected together without separation in both parts of the root canal has been developed [31]. Although non-extraction methods yield identical pulp regeneration on day 14 as in the extraction method (Fig. 15.3h, i, k, l), it is noteworthy that in the non-extraction method neither internal nor external root resorption is observed in the tooth. Induced vascularization in the regenerated tissue is similar in density and orientation to those in the normal pulp analyzed by the three-dimensional image of two-photon microscopy. Numerous transplanted cells are in the vicinity of newly formed capillaries and express angiogenic/neurotrophic factors, implicating a trophic role in neovascularization [26, 31]. DiI labelling on the regenerated pulp in the lower incisor has demonstrated the neuronal process from the regenerated pulp connecting to the inferior alveolar nerve [26]. Additional dentin formation along the dentinal wall and in the enlarged apical portion is detected on day 90 (Fig. 15.3j). It is noteworthy that transplantation of DPSC subfractions alone, or SDF-1 alone, yields significantly less pulp tissue. In addition, after transplantation of unfractionated DPSCs, the regenerated tissue is significantly less and undergoes mineralization [26]. The regenerated tissue by transplantation of the DPSC subfractions and SDF-1 has been demonstrated to be identical to normal functional pulp tissue by similar expression of the pulp tissue markers, Syndecan and TRH-DE mRNA. Furthermore, two-dimensional electrophoretic analyses and microarray analyses have shown that the qualitative and quantitative protein and mRNA expression patterns of the regenerated tissue are virtually identical to normal pulp [26, 38].
The possible mechanisms of pulp regeneration based on DPSC subfractions and SDF-1 are as follows: the CXCR4/SDF-1 axis may induce CXCR4-positive endogenous stem/progenitor cells to migrate into the root canal and proliferate and differentiate into the endothelial cells, pulp cells, and odontoblasts. Angiogenesis and reinnervation are stimulated by angiogenic/neurotrophic factors secreted by the transplanted stem cells. However, the precise mechanisms of recruitment and crucial molecules for cell migration are still unclear and need additional experimental scrutiny.
15.4.4 Preclinical Trial of Complete Pulp Regeneration
With the successful results on complete pulp regeneration described previously, a preclinical trial by harnessing pulp stem/progenitor cells with high angiogenic/neurogenic potential and homing/migration factors with proper scaffold has assessed its efficacy and safety as a preparation for the impending clinical trials. First, for clinical translation, it is essential to manufacture clinical-grade DPSC subsets according to good manufacturing practice (GMP) conditions without using conventional flow cytometry. To isolate DPSC subsets, a new cost-effective method has been devised by employing an optimized granulocyte colony-stimulating factor (G-CSF)-induced mobilization [29
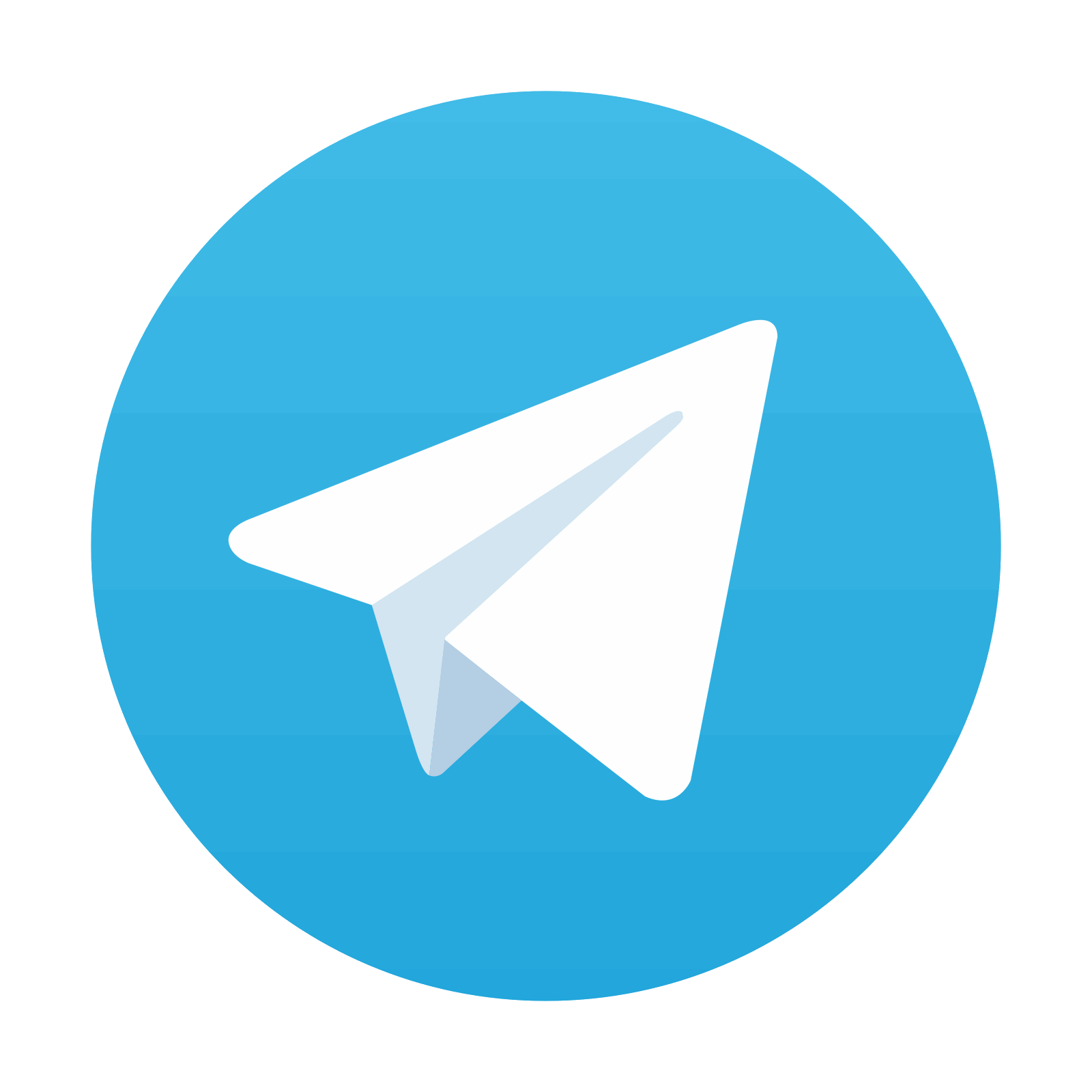
Stay updated, free dental videos. Join our Telegram channel

VIDEdental - Online dental courses
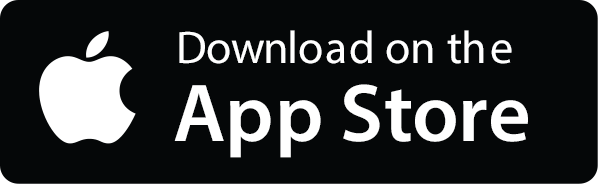
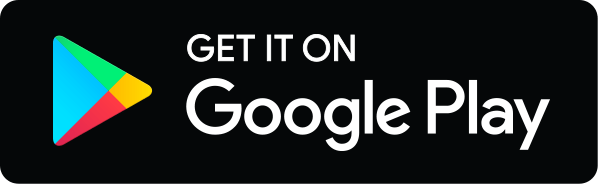