Introduction
This study aimed to determine the identifiability and measurement accuracy of defined bony defects of the temporomandibular joint (TMJ) in cone-beam computed tomographies, depending on different milliampere-second (mAs)-reduced protocols.
Methods
Defined artificial defects were prepared on 30 condyles from 15 intact pig heads, with a maximum of surrounding soft tissue preserved. Three-dimensional imaging was performed using ProMax 3D Mid (Planmeca, Helsinki, Finland). The scan protocol with maximum resolution and without mAs-reduction was defined as control. Twenty-six mAs-reduced imaging protocols were analyzed by 1 examiner regarding the protocol-specific measurement accuracy. Defect depth (DD) was divided into 3 categories: I, <2 mm; II, 2-3 mm; and III, >3 mm. The protocol-specific sensitivity and specificity were evaluated in relation to localization and defect size as determined from the results of 3 examiners.
Results
There was a significant difference from the control protocol in DD measurement in 8 mAs-reduced protocols, P <0.001-0.027. In most protocols, there was no significant difference in measurement accuracy concerning defect size and localization. The mean sensitivity reached values between 93.3% and 98.6% and differed significantly among protocols ( P = 0.002). The mean specificity amounted to 97.0%-98.1% and did not differ among protocols ( P = 0.462). The specificity of DD III (99.1%) was higher than DD I (97.7%) and DD II (97.1%). There was a significant difference in specificity and sensitivity concerning defect localization ( P <0.001).
Conclusions
This study showed that mAs-reduced cone-beam computed tomographies protocols are suitable for the analysis of defined osseous TMJ defects. When 3-dimensional TMJ imaging is indicated because of potential erosive defects, validated mAs-reduced scan protocols should be applied instead of high-definition protocols.
Highlights
- •
Mean sensitivities and specificities ranged from 93% to 98% and 97% to 98%, respectively.
- •
There were significant differences in sensitivity between protocols.
- •
There was slightly higher specificity in larger defects (>3 mm).
- •
There was excellent measurement accuracy of defect depth independent of the protocol.
- •
There was decreased measurement accuracy for larger defects.
Cone-beam computed tomography (CBCT) replaced conventional tomography (CT) in a high proportion of 3-dimensional dentomaxillofacial imaging and is accepted for a variety of diagnostic purposes in dentistry. Advantages of CBCT include lower radiation dose, lower cost, and higher resolution due to smaller field of views (FOVs), and justify an indication as an alternative to medical CT.
Since its introduction, CBCT became an established imaging modality for anomalies of the temporomandibular joint (TMJ). It provides crucial information for diagnosis and management of temporomandibular dysfunctions (TMD) and enables the diagnosis of initial changes in mandibular condyles of patients with juvenile idiopathic arthritis, such as small erosions. , CBCT is also accepted to evaluate the disease severity and progression in TMJ osteoarthritis. However, the diagnostic value is limited to hard tissues. Reliable information on the morphology of the condylar head, the continuity of cortical articular surfaces, and subcortical changes are available. Erosions are localized areas of decreased density with interruptions of the continuity of the condylar surface.
Many authors verified the good reliability of CBCT imaging for the assessment of osseous changes of the TMJ compared with physical macroscopic observation; thus, indicating the former as the gold standard. In contrast with CT or other conventional imaging modalities, a superior or at least equivalent detection accuracy of osseous defects was determined with CBCT. , , , The studies agree on a high specificity and significantly lower sensitivity. Ma et al reported in a 2016 meta-analysis a pooled specificity and sensitivity of 0.87 and 0.67, respectively. Some reasons for this discrepancy may be differing imaging modalities, study material, defect size, and morphology. Marques et al and Patel et al determined that the defect size significantly influenced sensitivity. The fairly high overall sensitivity (73%-88%) for the detection of simulated condylar defects decreased when evaluating defects <2 mm. Moreover, the imaging protocol affected the diagnostic accuracy of erosive lesions on the condyle. , Zhang et al described an inconsistency in the literature concerning the influence of spatial resolution on the accuracy of the evaluation of structures imaged with CBCT. Although some authors reported an increased sensitivity for detecting small condylar defects with increased scanning resolution, others concluded that when physical acquisition parameters within one CBCT imaging system are constant, different spatial resolutions do not necessarily produce different detection accuracies.
The quality of the CBCT image as well as the effective radiation dose are influenced by a variety of factors such as scanning unit, tube voltage, anode current, detector type, FOV, spatial resolution, scan time, and examined object. , In most CBCT systems, acquisition parameters are fixed, and only the FOV and the resolution can be selected according to the specific clinical task. Increased spatial resolution is usually associated with smaller FOVs and higher radiation doses.
The increased use of CBCT heated the debate on radiation hygiene. However, comparability is difficult because CBCT systems are an inhomogeneous device class concerning applied radiation dose and the image quality of anatomic structures. , The industry reacted with the introduction of so-called “ultra-low dose” CBCT machines. Ludlow and Walker reported a substantial effective dose reduction of 87% when using reduced exposure parameters compared with standard protocols. Pauwels et al found an association between reduced milliampere-second (mAs) values and a gradual reduction in clinical image quality. Because of the variety in exposure options, it is possible to address different diagnostic tasks with adapted radiation doses, but there is still a lack of well-defined CBCT protocols for specific diagnostic purposes in dentistry.
This study aimed to determine the identifiability and measurement accuracy of defined osseous defects of the TMJ in CBCTs depending on different mAs-reduced protocols. The null hypothesis was that decreased voxel sizes and increased mAs values correlate with increased detection and measurement accuracy.
Material and methods
Fifteen intact pig heads were acquired for this study. To investigate the articulating surfaces of the 15 right and left condyles, we mobilized the mandible by partial elevation and dissection of the musculi massetericus, temporalis, pterygoideus medialis, and lateralis. The joint capsule was exposed from the dorsal side and incised vertically. The capsule was elevated and dissected from the collum mandibulae on all sides except the anterior part. The ligamentum laterale and the stratum inferius were dissected. All surrounding soft tissues were left intact. The mandible was then luxated in a dorsocaudal direction, exposing the condylar surface. The soft tissues were only elevated and not removed to reposition them in the correct area later. All preparations were carried out standardized and systematically by 1 person with experience in the preparation of human cadaver heads for anatomic courses. Four gutta-percha markings were attached just beyond the study area to partition the articular surface into 4 quadrants (posterior-medial, anterior-medial, posterior-lateral, anterior-lateral) per condyle. Predefined defects were placed with a round bur (Ø: 1 mm, 2 mm, or 3 mm) and water cooling. The specific defect depth (DD) was ensured by a periodontal probe. Defects were classified into 1 of 3 defect groups: DG I, <2 mm; DG II, 2-3 mm; and DG III, >3 mm). They were randomly distributed with a maximum of 4 defects per quadrant. An example of a prepared condyle and the visual representation in the CBCT image can be seen in Figures 1 and 2 . The total number of defects was 170. Seventeen quadrants were left without defect as control. Finally, the condyle, as well as all soft tissues, were repositioned and fixated.


Three-dimensional imaging was performed using a ProMax 3D Mid (Planmeca, Helsinki, Finland). Two FOVs were used (20 × 17 cm and 20 × 10 cm). The scan protocol with maximum resolution and without mAs-reduction was defined as the control protocol. The control resolution was 200 μm voxel size with a mAs-product of 360 mAs (20 × 17 cm) and 180 mAs (20 × 10 cm). Twenty-six mAs-reduced imaging protocols were used that differed in anode current, exposure time, resolution, and FOV. The specific parameters are listed in Tables I and II . According to the resolution, 3 groups can be distinguished (low-definition≙600 μm, normal-definition≙400 μm, and high-definition≙200 μm). A series of images of the same condyle and defect but different representative protocols can be seen in Supplementary Figures 1-6 . Image analyses were done by 4 blinded examiners who had experience with CBCT evaluation. They were calibrated by a senior employee of the radiological institute, MESANTIS 3D DENTAL-RADIOLOGICUM, Berlin, Germany.
Protocol | Resolution category | Voxel size, μm | Anode current, mA | Exposure time, s | mAs-product, mAs |
---|---|---|---|---|---|
1 | LD | 600 | 5 | 9 | 45 |
2 | LD | 600 | 4 | 9 | 36 |
3 | LD | 600 | 3.2 | 9 | 27 |
4 | LD | 600 | 2.5 | 9 | 22.5 |
5 | LD | 600 | 2 | 9 | 18 |
6 | ND | 400 | 7.1 | 9 | 63.9 |
7 | ND | 400 | 6.3 | 9 | 54 |
8 | ND | 400 | 5 | 9 | 45 |
9 | ND | 400 | 4 | 9 | 36 |
10 | HD | 200 | 7.1 | 12 | 85.2 |
11 | HD | 200 | 6.3 | 12 | 72 |
12 | HD | 200 | 5 | 12 | 60 |
13 | HD | 200 | 4 | 12 | 48 |
14 | HD | 200 | 3.2 | 12 | 38.4 |
Protocol | Resolution category | Voxel size, μm | Anode current, mA | Exposure time, s | mAs-product, mAs |
---|---|---|---|---|---|
15 | LD | 600 | 6.3 | 4.5 | 27 |
16 | LD | 600 | 5 | 4.5 | 22.5 |
17 | LD | 600 | 4 | 4.5 | 18 |
18 | LD | 600 | 2.5 | 4.5 | 11.25 |
19 | ND | 400 | 7.1 | 4.5 | 32 |
20 | ND | 400 | 6.3 | 4.5 | 27 |
21 | ND | 400 | 5 | 4.5 | 22.5 |
22 | ND | 400 | 4 | 4.5 | 18 |
23 | HD | 200 | 7.1 | 6 | 42.6 |
24 | HD | 200 | 6.3 | 6 | 36 |
25 | HD | 200 | 5 | 6 | 30 |
26 | HD | 200 | 4 | 6 | 24 |
Image evaluation consisted of 2 parts. One examiner measured the DD perpendicular to the articular surface on the maximum DD for the calculation of measurement accuracy. He was also responsible for the standardized adjustment and orientation of the images for all other examiners. The images were oriented according to the gutta-percha markings to simplify the classification of defect localization. All 2-dimensional planes of the multiplanar reconstruction were used for the evaluation. Three examiners who were not allowed to use the measuring tool were instructed to identify the defects, determine the defect localization, and guess the DD according to the DG to calculate sensitivity, specificity, and accuracy. Except for image orientation, no further assistance was provided. The 3 investigators had to scroll through the CBCT volume by themselves to identify and categorize the defects. Four condyles were evaluated in the control protocols repeatedly to measure intra- and interindividual reliability.
Statistical analysis
A power analysis concerning the null hypothesis was conducted to determine the needed sample size. We considered a difference in DD measurement of 0.5 mm between the investigated and control protocol as clinically relevant and defined a power of 80% and a significance level of 0.05 to detect this difference. A similar study with artificial defects on pig condyles suggests a normal distribution of the data with a standard deviation for interrater reliability of 0.4 mm. A sample size of at least 11 defects for each DD category was calculated to answer the research question. Descriptive and explorative data analysis was done using SPSS software (version 20; SPSS, Chicago, Ill). The significance level was defined as 0.05. Intraindividual reproducibility of DD measurement was evaluated with the Houston reliability coefficient and intraclass correlation (ICC). Intra- and interindividual reproducibility for defect identification and classification was calculated with Cohen’s kappa coefficient and agreement in percent.
Because of inconsistencies in the distribution pattern of the protocol-specific differences from the control protocol, a mix of parametric and nonparametric tests were performed, depending on sample size for the specific comparisons. Protocol-specific measurement accuracy compared with the control protocol was tested with ICC and paired t test. In addition, a graphical evaluation in the form of Bland-Altman plots with limits of agreement (LOA; ± 1.96 [standard deviation]) was conducted. A comparison of measurement accuracy between protocols was made with a 1-way analysis of variance. The Kruskal-Wallis test for independent samples was used to evaluate differences in measurement accuracy between DD and localization categories because this dataset had a small sample size and was not normally distributed. Interaction of DD and localization concerning measurement accuracy was tested with a 2-way analysis of variance. The datasets from each examiner were combined for qualitative analysis. In addition to the protocol-specific sensitivity and specificity, a chi-square test was used to evaluate the reliability of defect identification and categorization and to compare DD and localization groups. Differences between the mAs-reduced protocols were analyzed with Cochran’s Q. McNemar’s test was used for pairwise comparison when Cochran’s Q showed significant results. These comparisons were based on dichotomous data, not interval-scaled sensitivity or specificity.
Results
The intrarater reliability of DD measurement was good to excellent with an ICC of 0.799-0.997 (95% confidence interval, 0.679-0.997) and a Houston reliability coefficient of 96.6%-97.6%. The mean standard error was 0.03-0.05 mm with a variance of 0.01-0.03 mm 2 . The intrarater and interrater reliability of defect identification and categorization were substantial to almost perfect. The intrarater reproducibility for the 3 examiners was 90%-95% (κ = 0.754-0.918). The interrater reliability was lower, with 78% (κ = 0.795).
The mean sensitivity for identification and classification of the defects was 93.3%-98.2%, with a significant difference between mAs-reduced protocols ( P = 0.002). The mean specificity ranged from 97.0% to 98.1% and did not differ significantly between protocols ( P = 0.462). The DD-specific sensitivity was 97.6%-98.2% and did not differ significantly among DD groups ( P = 0.378). The mean specificity of defects >3 mm (99.1%) was larger than of DG I and II (97.7% and 97.1%, respectively). Moreover, there was a significant difference in sensitivity and specificity regarding defect localization ( P <0.001). The highest mean sensitivity was determined for the posterior-lateral quadrant with 100%. The sensitivity, specificity, and accuracy for the different DD and localization groups are presented in Table III .
Defect category | Specificity, % | Sensitivity, % | Accuracy, % |
---|---|---|---|
DD | |||
<2 mm | 97.7 ± 1.7 | 97.6 ± 3.5 | 97.7 ± 1.7 |
2-3 mm | 97.1 ± 1.8 | 97.6 ± 3.6 | 97.4 ± 1.8 |
>3 mm | 99.1 ± 1.1 | 98.2 ± 2.4 | 98.7 ± 1.2 |
Defect localization | |||
Posterior-medial | 97.2 ± 1.6 | 97.6 ± 2.7 | 97.4 ± 1.4 |
Posterior-lateral | 97.6 ± 1.8 | 100 ± 0.0 | 98.6 ± 1.0 |
Anterior-medial | 99.3 ± 0.8 | 97.3 ± 3.9 | 98.4 ± 1.8 |
Anterior-lateral | 97.8 ± 1.8 | 96.3 ± 3.4 | 97.2 ± 1.8 |
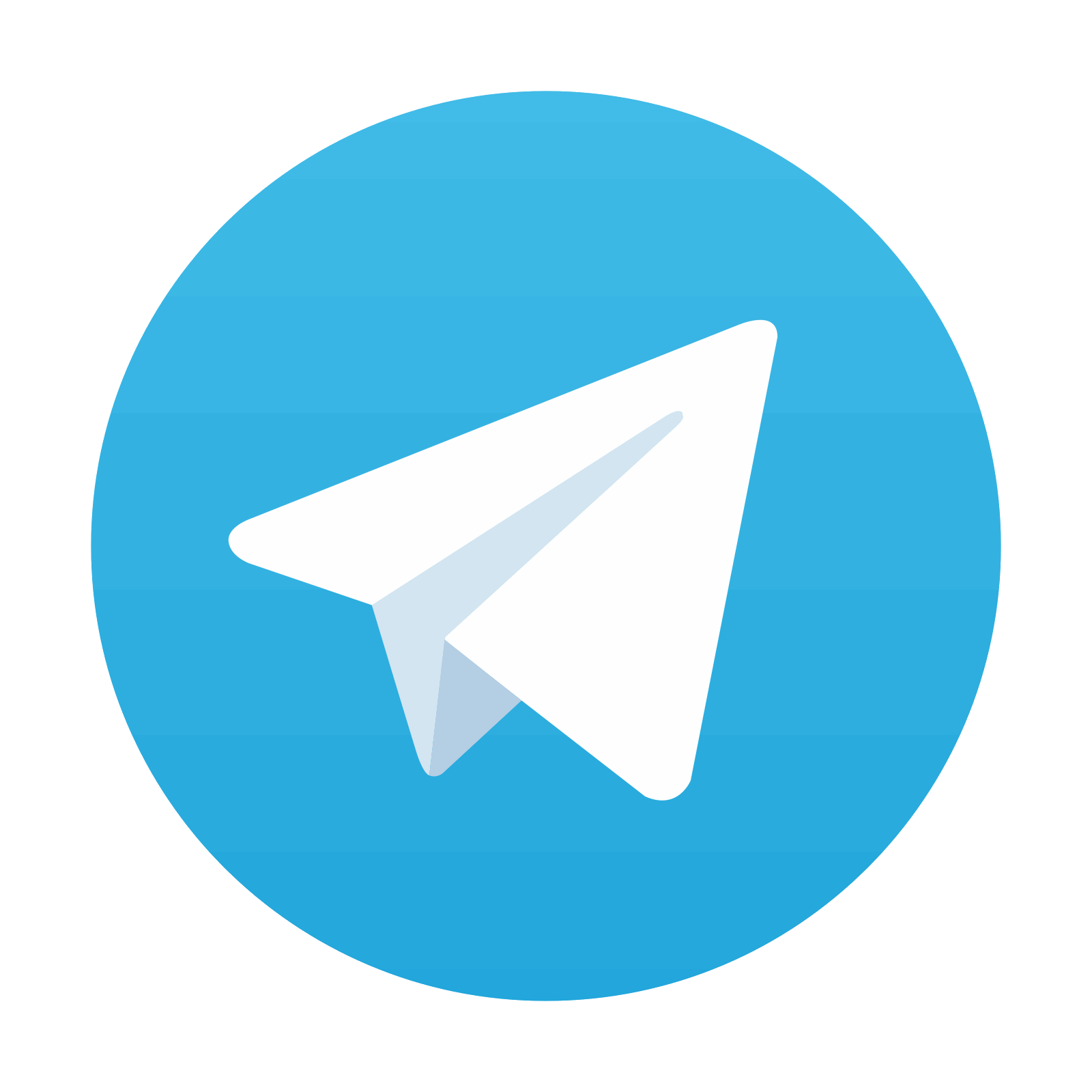
Stay updated, free dental videos. Join our Telegram channel

VIDEdental - Online dental courses
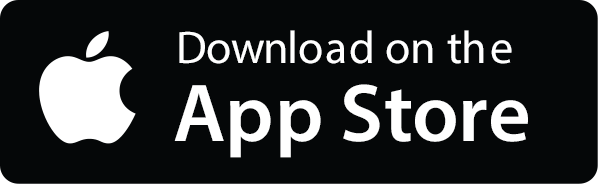
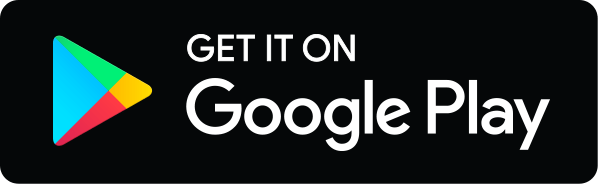
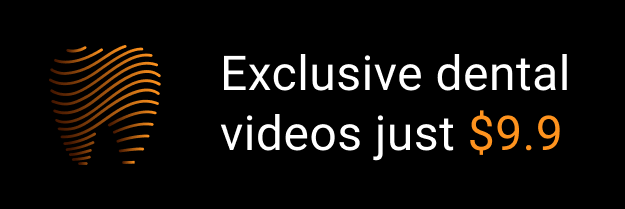