Introduction
Orthodontic movement triggers a sequence of cellular and molecular events that may be affected by different systemic conditions. This study evaluated the effect of obesity on rat periodontal tissue remodeling induced by mechanical orthodontic force.
Methods
Thirty-two Holtzman rats were distributed into 4 groups: control, obesity induction (O), orthodontic movement (M), and obesity induction and orthodontic movement (OM). Obesity was induced by a high-fat diet for 90 days. After 15 days of orthodontic movement, the animals were killed. Obesity induction was confirmed by animal body weight, adipose tissue weight, and serologic analysis. Periodontal tissue remodeling was evaluated using microcomputed tomography and histologic analysis. The gene expression of adipokines and cytokines in gingival tissues was evaluated.
Results
An increase in body and adipose tissue weight was observed in the obesity induction groups. The O group presented an increase in lipids and blood glucose. The OM group showed a decrease in bone volume fraction and bone mineral density compared with all other groups and a tendency for more rapid tooth movement than the M group. The OM group showed a higher quantity of inflammatory cells and higher Mmp1 expression than the O group. The O and OM groups showed higher Nampt expression than the control group and lower Nampt expression than the M group.
Conclusions
Obesity modulates periodontal tissue remodeling during orthodontic movement and results in more inflammation and bone loss than in nonobese animals.
Highlights
- •
Obesity associated with orthodontic movement increases inflammation.
- •
Obesity associated with orthodontic movement induces alveolar bone loss.
- •
Orthodontic movement and obesity affect the gene expression of adipokines and cytokines.
Obesity is a metabolic disorder characterized by excessive fat accumulation that has a negative impact on health. It occurs because of increased caloric intake and/or a sedentary lifestyle, genetic factors, and hormonal dysfunctions. In adults, obesity is defined as a body mass index greater than or equal to 30 kg/m 2 . According to the World Health Organization, the worldwide prevalence of obesity almost tripled between 1975 and 2016, and overweight and obesity are linked with more deaths worldwide than underweight. Furthermore, obesity is associated with many pathologies, such as cardiovascular disease, rheumatoid arthritis, type 2 diabetes mellitus, and certain types of cancer. The trigger that initiates the inflammatory process in obesity has not been fully elucidated. However, one hypothesis is that an excess of nutrients present in metabolic cells, such as adipocytes, promotes the activation of an inflammatory cascade, resulting in increased cytokine and adipokine release. In addition, an increase in adipocyte volume leads to the secretion of inflammatory cytokines such as tumor necrosis factor-alpha (TNFa), interleukin-6 (IL-6), and interleukin-1beta (IL-1b) and hypoxia. As a consequence, local and systemic inflammation is increased.
Adipokines produced by the adipose tissue can be proinflammatory, such as leptin, visfatin, and resistin, or anti-inflammatory, such as adiponectin. Adipokines can regulate insulin resistance, energy output, and immuno-inflammatory and healing processes. Interestingly, patients with inflammatory disorders have high serum levels of adipokines like obese subjects. Moreover, high levels of visfatin were detected in the crevicular gingival fluid and serum of patients with periodontal disease, suggesting that visfatin is produced in the periodontium and is regulated by the inflammatory process. ,
In a previous study, our research group demonstrated that visfatin is produced by periodontal ligament (PDL) cells and that its production is increased in the presence of Fusobacterium nucleatum and decreased by biomechanical force. Leptin, another proinflammatory adipokine, is known to modulate appetite and satiety and influence energy expenditure and body weight. In obesity, the hypothalamus presents insulin and leptin resistance, which results in appetite and satiety dysregulation, causing an increase in fatty tissue and body weight. Resistin does not have a clear role in humans; however, it has been associated with insulin resistance, type 2 diabetes mellitus, arteriosclerosis, and cardiovascular diseases. Receptors for the anti-inflammatory adipokine, adiponectin, are also present in gingival fibroblasts and human PDL cells. This adipokine is responsible for modulating metabolic and immune processes by downregulation of proinflammatory cytokines and upregulation of anti-inflammatory cytokines. The levels of adiponectin in inflammatory disorders such as obesity, insulin resistance, and diabetes are lower than normal.
Obesity can cause an increase in the density and size of the vertebral bone and, consequently, an increase in skeletal growth. This growth leads to early jaw maturation, causing malocclusions. Therefore, orthodontic treatment is commonly required for obese subjects. Biomechanical forces provoked by orthodontic appliances act on periodontal tissues and cause aseptic inflammation, resulting in coordinated bone remodeling, consisting of bone resorption predominantly on the pressure side and bone apposition predominantly on the tension side. This bone remodeling occurs in the presence of fibroblasts, osteoclasts, osteoblasts, lymphocytes, and other immune cells. During this process, several proinflammatory cytokines are released, such as IL-1, IL-6, and TNFa. Regarding the adipokines, levels of leptin in the gingival crevicular fluid of nonobese patients, are reduced by orthodontic movement. In contrast, the average concentration of leptin in the saliva of obese patients during orthodontic treatment was 3 times higher when compared with the saliva of nonobese subjects, showing a strong correlation between leptin and dental movement in these patients.
The presence of specific biomarkers such as leptin and resistin in the gingival crevicular fluid of obese subjects shows a proinflammatory state in the periodontium of these patients. Serum levels of leptin, resistin, and receptor activator of NFkB ligand differ between obese and nonobese patients. Although not significantly different, orthodontic treatment in obese subjects seems to require a shorter time. However, the association of orthodontic movement with a pathologic inflammatory disease, such as periodontitis, enhances inflammatory processes and osteoclastogenesis, resulting in greater destruction of periodontal tissues. Given that obesity is increasing worldwide, studies to evaluate the effect of this systemic condition on periodontal tissues during orthodontic movement are necessary.
Therefore, this study aimed to evaluate the effect of obesity on rat periodontal tissue remodeling caused by orthodontic force.
Material and methods
This study was approved by the Ethics Committee on Animal Experimentation of the Sao Paulo State University, School of Dentistry at Araraquara, Brazil (protocol no. 16/2015). The sample size was calculated by analysis of variance on the basis of microcomputed tomography (microCT) analysis performed in a previous study by our group. Thirty-two male adult Holtzman rats, with an average weight of 300 g, were used. These animals were maintained in the animal facilities of the University in plastic cages, under controlled temperature (22°C-25°C) with a 12-hour light/dark cycle, and received standard laboratory diet (Labina/Purina, Ribeirão Preto, Brazil) or high-fat diet and water ad libitum. The animals were randomly assigned into 4 experimental groups: (1) control/without any intervention (C), (2) obesity induction (O), (3) orthodontic movement (M), and (4) obesity induction and orthodontic movement (OM). The animals’ body mass was recorded weekly.
The animals in the O and OM groups received a high-fat diet to induce obesity. This diet contained around 3.82 kcal/g and was composed of standard rat chow plus peanuts, milk chocolate, and sweet biscuits in a 3:2:2:1 ratio, and contained 20 g of protein, 20 g of total fat, 48 g of carbohydrate, and 4 g of fiber per 100 g of diet. In contrast, the standard diet contained around 2.25 kcal/g and 22 g of protein, 48 g of carbohydrate, 4 g of total fat, 8 g of fiber, and 200 mg of sodium per 100 g of diet. The high-fat diet was provided daily and had started 75 days before baseline when orthodontic movement was initiated.
At baseline, general anesthesia was performed with intramuscular injections of ketamine chlorhydrate 10% (0.08 mL/100 g body weight) and xylazine chlorhydrate 2% (0.04 mL/100 g body weight) in the animals in the M and OM groups. Then, a closed coil nickel-titanium Sentalloy spring (GAC, Central Islip, NY), which provided a relatively constant 25 g of force during the experiment, was connected between the maxillary first molars and the maxillary central incisors with the aid of a steel wire (0.20 mm, 55.01.208; Morelli, Sorocaba, Brazil). For spring placement around the maxillary central incisors, grooves were prepared on their mesial, distal, and vestibular surfaces, and a thin layer of composite resin was placed to cover the steel wire. For the maxillary first molars, the spring was positioned on the occlusal surface and composite resin was placed to cover it. To avoid occlusal interference, we extracted the mandibular first molar. The animals received paracetamol 200 mg/kg by oral administration immediately after surgery.
After 15 days from baseline, all animals were killed. The maxillary jaws were collected for microCT and histologic analyses, aiming to evaluate, respectively, mineralized and nonmineralized periodontal tissues. Gingival tissue around the maxillary first molars was excised for analysis of cytokine and adipokine gene expression by reverse transcription-quantitative polymerase chain reaction. For serum analysis, the animals fasted for 12 hours before sacrifice. Blood was collected through cardiac puncture, and after centrifugation (3000 rpm at 23°C for 10 minutes), the plasma was analyzed for triglycerides, total cholesterol, high-density lipoprotein (HDL), low-density lipoprotein (LDL), and insulin. Epididymal, retroperitoneal, and mesenteric adipose tissues were removed and weighed on a precision scale.
The hemimaxillae of all animals were fixed in 4% paraformaldehyde for 48 hours and stored in 70% ethanol before microCT scanning (μCT Skyscan 1176; Bruker, Kontich, Belgium). Scanning was performed using an x-ray generator operating at 50 kVp, beam current at 500 μA, with a 0.5 mm aluminum filter and image resolution of 18 μm. The images were reconstructed using software (NRecon, version 1.6.1.5; Bruker) in all 3 dimensions. Data Viewer (version 1.4.3.1, Skyscan; Bruker) and CTan (CT-Analyser software, version 1.10.1.0, Skyscan; Bruker) were used for image reorientation and volumetric analysis. A region of interest (ROI) was delimited from the root apices of the first and second maxillary molars to the alveolar crest in the apical-coronal direction. In the mesial-distal direction, the limits were the mesial face of the second molars, and therefore all the alveolar bone around the first molars was included in the ROI. Tooth roots and PDL were excluded from the ROI. The microarchitecture parameters evaluated were bone volume (BV), tissue volume, bone volume fraction (BV/tissue volume [%]), and bone mineral density (BMD). The BMD measurement was performed with phantom rods at densities previously established by the manufacturer (Bruker). To calculate tooth movement, we measured the horizontal distance between the highest convexity on the distal surface of the first molars and the highest convexity on the mesial surface of the second molars using the Data Viewer software (Bruker).
Eight hemimaxillae per group were used to evaluate morphologic changes. Serial parasagittal sections (4 μm) were mounted on slides and stained with hematoxylin and eosin (HE). Two sections of each tooth were selected for descriptive histologic, histometric, and stereometric analyses. These analyses were performed by a blinded and calibrated examiner using Leica DMLS microscopy (Leica DMLS; Leica/Reichert Diastar Products & Jung, Wetzlar, Germany) at a magnification of 50× for histometric analysis and 200× for stereometric analysis. The areas of interest were selected and photographed using a Leica DFC 300 FX digital camera, and the captured images were archived in tagged image file format. The histometric analysis consisted of measuring the alveolar linear bone loss in the interproximal region, using an image-analysis system (Image J 1.37b; National Institutes of Health, Bethesda, Md). The distance between the cementoenamel junction to the alveolar bone crest was determined on the distal surface of the maxillary first molars. The stereometric analysis quantified the presence of inflammatory cells, fibroblasts, blood vessels, and other structures using a point-counting technique. A 60 mm × 135 mm (8100 mm 2 ) grid with 50 points was constructed using image editing software (Adobe Photoshop CS6; Adobe System Incorporated, San Jose, Calif) and overlaid on the digital images obtained from the histologic sections. The grid was positioned over the bone crest and under the epithelial tissue using the image editing software. The structure observed at each intersecting point of the grid was recorded. The identification of cell type (inflammatory or fibroblast) was on the basis of their morphologic features. In general, inflammatory cells have a rounded shape, whereas fibroblasts are elongated/fusiform. Vascular and other structures (unidentified) were quantified as previously described. , , To guarantee that the structures were correctly identified, the analysis was performed under a light microscope to confirm at higher magnification any doubt that the examiner might have. If any doubt persisted, the structure was recorded as other structure . The presence of each structure was expressed as a percentage of the total points analyzed in the total area.
Root resorption was also evaluated in the first molars. Serial parasagittal sections (4 μm) were mounted on slides and stained with HE. Using a camera (DP71; Olympus, Tokyo, Japan) attached to a light microscope (BX51; Olympus), an image of the first molar was captured at ×65 magnification. The root surface of the first molars was measured using an image-analysis system (Image-Pro Express 6.0; Olympus). Subsequently, the linear surface of the excavated areas on the root surface was measured and the resorbed surface per total length of the root surface was calculated.
RNA was extracted from the gingival tissues collected from 6 hemimaxillae per group using an RNeasy Mini kit (Qiagen, Valencia, Calif) according to the manufacturer’s protocol. RNA concentration was determined using a NanoVue UV/Visible Spectrophotometer (GE Healthcare, Piscataway, NJ), and 500 ng of total RNA was reverse transcribed using a High Capacity cDNA Reverse Transcription Kit (Applied Biosystems, Foster City, Calif) according to the manufacturer’s instructions. The gene expression of Il1b , Tnfa , Il6 , Nampt , Adipoq , Mmp1, and Gapdh was analyzed by reverse transcription-polymerase chain reaction in an iCycler iQ Real-Time PCR Detection System (Bio-Rad, Richmon, Calif), using SYBR Green Master Mix (Qiagen) and specific primers (QuantiTect Primer Assay; Qiagen). Amplification was carried out under the following conditions: initial denaturation at 95°C for 5 minutes followed by 40 cycles of denaturation at 95°C for 10 seconds and combined annealing/extension at 60°C for 30 seconds. Data were analyzed using the comparative cycle threshold method.
To evaluate whether obesity interferes in collagen degradation and, consequently, in orthodontic movement, the collagen content on the pressure and tension sides of the PDL was estimated . The sections were stained with 0.1% picrosirius red solution and analyzed under a polarized light microscope (BX51; Olympus). For each specimen, 2 nonserial sections of the entire distal root taken at intervals of at least 60 μm were used to measure the birefringent collagen content on the pressure and tension sides of the PDL from the distal root of the first molars. In each section, 3 standardized areas (cervical, middle, and apical portions) of each side (pressure and tension) of the PDL were captured at magnification of 400×.
The birefringent collagen content was determined using image-analysis software (Image J 1.37b) according to the methodology described by Manni et al. Data for each hue (red, orange, yellow, and green) were determined as a percentage of the total number of collagen pixels, which in turn was expressed as a percentage of the total number of pixels in the image.
Statistical analysis
Analyses were performed using GraphPad Prism software (GraphPad Software, San Diego, Calif). The Shapiro-Wilk test was used to confirm the normal distribution of all data ( P >0.05). One-way analysis of variance was followed by post-hoc Tukey test for multiple comparisons among groups ( P ≤0.05). The Kruskal-Wallis test followed by the Dunn test was used for stereometrics analysis ( P ≤0.05), and the Student t test was used for comparisons between 2 groups ( P ≤0.05).
Results
Obesity was confirmed by measuring body and adipose tissue weight and by plasma lipid profile analysis. Animals in the O and OM groups presented a significantly higher body weight than nonobese animals (C and M) ( P <0.05) after the fourth week of high-fat diet intake. However, after the eleventh week, only animals from the O group showed a significant difference ( P <0.05) in comparison with the other groups ( Fig 1 , A ). At sacrifice, animals from both obese groups (O and OM) had significantly heavier epididymal, retroperitoneal, and mesenteric adipose tissues than the nonobese groups (C and M) ( P <0.05) ( Fig 1 , B ).
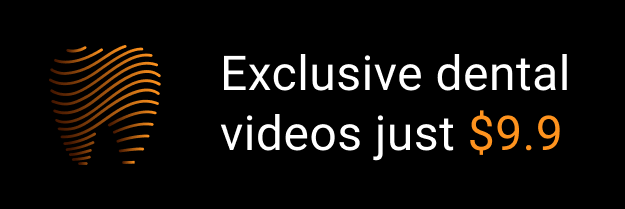