Highlights
- •
Addition of Al 2 O 3 result in decrease in sintering shrinkage and relative density.
- •
Different forms of Al 2 O 3 in Al 2 O 3 –ZrO 2 composite result in different mechanical properties.
- •
Addition of microfibre form Al 2 O 3 result in enhanced mechanical properties of Al 2 O 3 –ZrO 2 .
- •
Addition of microfibre form Al 2 O 3 result in lowest Weibull modulus.
- •
Addition of greater than 20 vol% of microfibre form Al 2 O 3 result in agglomeration.
Abstract
Objectives
This study evaluates the difference in physical and mechanical properties of ZrO 2 ceramics, commonly used in dental applications, altered by three different forms of Al 2 O 3 content; microparticles (m), nanoparticles (n), and microfiber (f).
Methods
Three different types of ZrO 2 –Al 2 O 3 composites were formed using microparticle (m), nanoparticle (n), or microfibre (f) forms of Al 2 O 3 . The physical and mechanical properties such as sintering shrinkage, relative density, Vickers hardness, fracture toughness, and biaxial strength were evaluated. A Weibull analysis was performed to assess the strength reliability of the specimens. All data were calculated using the t-test and ANOVA.
Results
The sintering shrinkage and relative density of all ceramic composite groups were decreased with the addition of Al 2 O 3 . The mechanical properties of ZrO 2 –Al 2 O 3 (f) composite were higher than that of ZrO 2 –Al 2 O 3 (m) composite and ZrO 2 –Al 2 O 3 (n) composite. The maximum hardness, fracture toughness, and biaxial flexural strength were observed for 10 vol% of Al 2 O 3 fibre. When the content of Al 2 O 3 fibre in the matrix was increased above 20 vol%, agglomeration occurred and resulted in a decrease of hardness and toughness. The Weibull modulus value of the ZrO 2 –Al 2 O 3 (f) composite was the lowest compared to that of other groups. However, characteristic strength (σ 0 ) of ZrO 2 –Al 2 O 3 (f) the highest value.
Significance
The current study demonstrated that the addition of right amount of Al 2 O 3 microfibre into the ZrO 2 matrix enhanced the mechanical properties of ZrO 2 -Al 2 O 3 (f) composite, which would be favourable for dental applications.
1
Introduction
Zirconia (ZrO 2 ) has been increasingly used as the dental ceramicdue to its high strength and fracture toughness as well as its colour, which is similar to that of a natural tooth [ ]. Especially, the development of high-toughness ceramics has led to an increased use of ZrO 2 stabilized with Y 2 O 3 (Y-TZP) in clinical applications [ , ]. Although the addition of Y 2 O 3 retained the tetragonal phase of the ZrO 2 at room temperatures, when a crack initiates on the surface of Y-TZP, the stress concentration at the top of the crack causes the tetragonal crystal to transform into a monoclinic crystal, with associated volumetric expansion [ ]. In addition, the fracture toughness of monolithic ceramics is generally very poor and limits their applications. Hence, to minimize undesired events of surface cracking or change in the surface microstructure during clinical usage, the hardness and fracture toughness of tetragonal ZrO 2 still needs to be improved [ ].
To increase the mechanical properties of ZrO 2 , Al 2 O 3 is recommended as a reinforcement agent for a ZrO 2 composite [ ]. Reinforcement materials for ceramic composites can be chosen in forms of particle-, fibre- or whisker-type. A recent trend in the research for ceramic composites is to incorporate strong ceramic fibre into ceramic matrices to form a fibre-reinforced ceramic matrix composite. These composites are being extensively studied to enhance their mechanical properties, leading to the interest of researchers in the development of fibre-reinforced ceramic composite systems [ ]. Such studies were concentrated on the effect of adding Al 2 O 3 particles to ZrO 2 –Al 2 O 3 composites. There has been a limited number of research on how different forms of Al 2 O 3 such as microparticles, nanoparticles, and microfibres would influence the ZrO 2 –Al 2 O 3 composites.
Hence, in this study, the alteration of physical and mechanical properties of ZrO 2 ceramics according to the addition of different forms of Al 2 O 3 were investigated in association with the possible use of the ZrO 2 –Al 2 O 3 composites in dentistry. Three different forms of Al 2 O 3 were considered, which included microparticle (Al 2 O 3 (m)), nanoparticle (Al 2 O 3 (n)) and microfibre (Al 2 O 3 (f)).
2
Materials and methods
2.1
Materials
For all test groups, 3 mol % yttria-stabilized tetragonal zirconia powder (KZ-3YF, Kyoritsu, Japan) was used. In terms of microparticle, nanoparticle and microfibre forms of Al 2 O 3 , micro sized Al 2 O 3 particles (ALM-43, Sumitomo. Chemical Co., Japan), nanosized Al 2 O 3 particles (Aeroxide Alu C, Degussa Evonik, Germany), and Al 2 O 3 fibre (B97N3, Denka Alsen, Denka Co., Japan) were used, respectively.
2.2
Preparation of ZrO 2 –Al 2 O 3 composite
The ZrO 2 and ZrO 2 –Al 2 O 3 composite (90:10 vol %) was prepared using yttria-stabilized tetragonal zirconia powder and their different types of alumina: Al 2 O 3 (m), Al 2 O 3 (n) and Al 2 O 3 (f). Additionally, ZrO 2 -based ceramic composites with varying Al 2 O 3 fibre content (5, 15 and 20 vol%) were prepared.
The mixed powders were ball-milled for 24 h in ethanol. The slurries were dried at 80 °C for 24 h and sieved through a 212 μm mesh. Disk shape samples (13.00 ± 0.05 mm in diameter, 1.75 ± 0.05 mm in thickness) were prepared from the powders obtained under uniaxial pressure of 1 ton in a steel mould for 2 min. The pressed specimens were sintered in an electric furnace (Super Kanthal Furnace, Lindberg. Blue M, USA) at 1450 °C for 2 h at a heating and cooling rate of 10 °C/min. After sintering, the specimens were cooled to room temperature.
2.3
Shrinkage and relative density
To investigate shrinkage behaviour of each specimen, sintering shrinkage of all specimens was calculated by measuring diameter and thickness before and after sintering. Eighteen specimens were made for each group.
The bulk density of each of the sintered samples (n = 5 per group) was measured in water using the Archimedes method. The theoretical density was determined using the rule of mixtures. The relative density was calculated as the ratio of the bulk density of the sintered specimens to the theoretical density.
2.4
Vickers hardness and fracture toughness
The Vickers hardness and fracture toughness values of the ZrO 2 and ZrO 2 –Al 2 O 3 composite specimens (n = 10 per group) were measured using the indentation method. A maximum load of 9.8 N (1 kg) was applied to the specimen surface with a dwell time of 15 s (Microhardness tester, Matsuzawa, Inc., MXD-CX3E, Japan). Three readings were taken for each ten specimens from each group, and the mean Vickers hardness value was recorded. Hardness and fracture toughness of the ZrO 2 and ZrO 2 -Al 2 O 3 composite were calculated from the following Eqs. (1) and (2) [ ];
Hv=1.854×Pd2
where p is the indentation load (N), d is an average length of the two diagonals of the indentation (mm), and H v is the Vickers hardness (GPa) [ ];
KIC=0.0726×Pc32
where P is the applied load for indentation (N), c is the 1/2 of crack length (m) and K IC is the fracture toughness (MPa m 1/2 )
2.5
Biaxial flexural strength and Weibull analysis
The biaxial flexural strength test of eleven specimens per test group was measured by using the piston-on-three ball technique in a universal testing machine at a 1.0 mm/min crosshead speed. The fracture load for each specimen was measured, and the biaxial flexural strength was calculated for measured value using the Eq. (3) [ ];
S=P-0.2387(X-Y)d2
where s is the maximum centre tensile stress (MPa), P is the load at fracture (N) and d is the thickness at the specimen disk centre (mm).
X and Y were calculated using following two Eqs. (4) and (5) ;
X=1+νlnγ2γ32+1-ν2γ2γ32
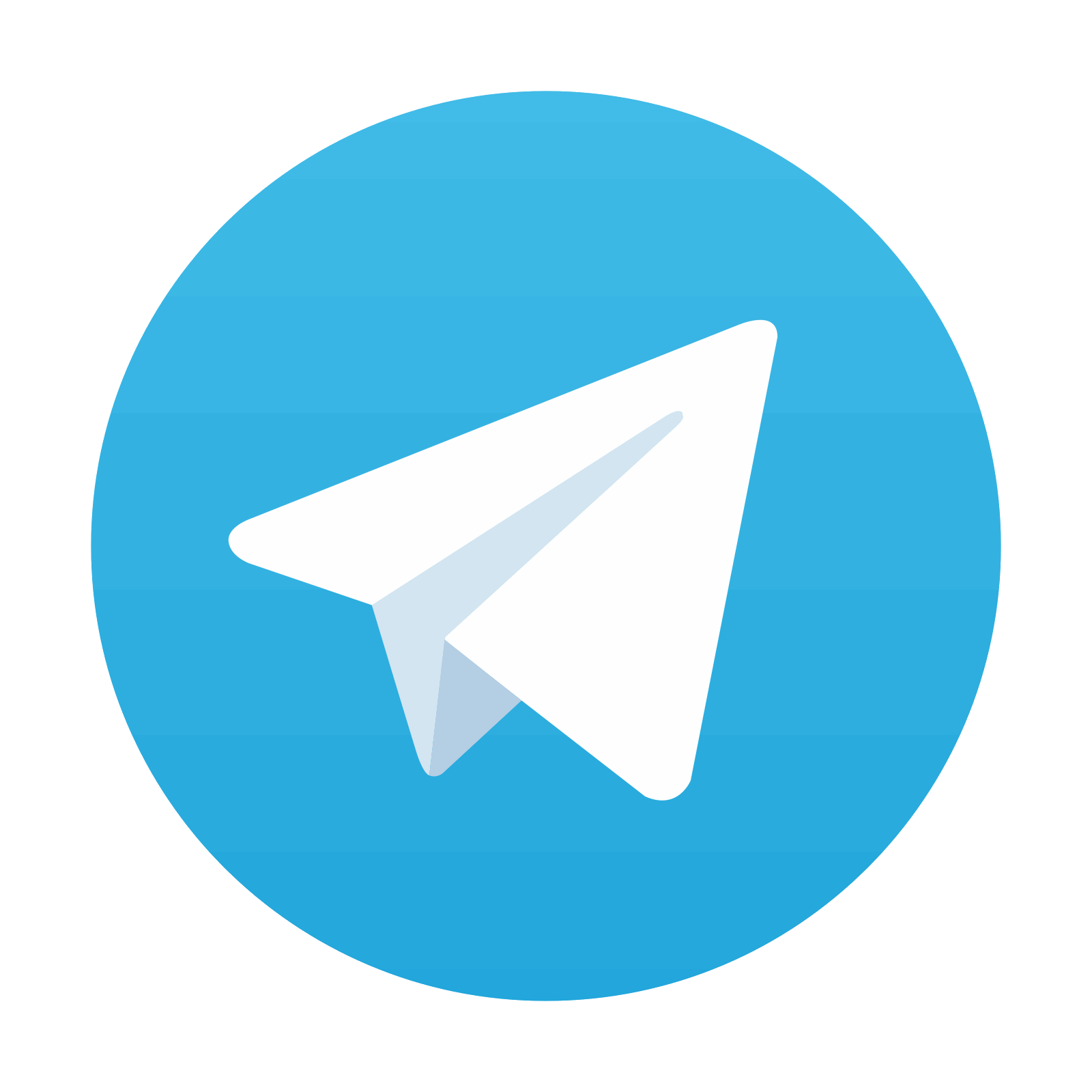
Stay updated, free dental videos. Join our Telegram channel

VIDEdental - Online dental courses
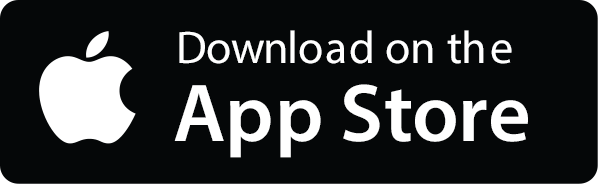
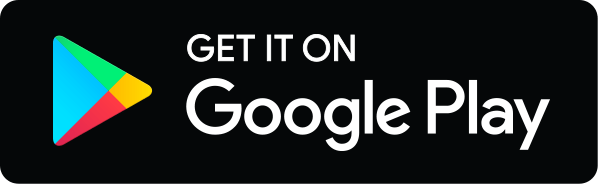
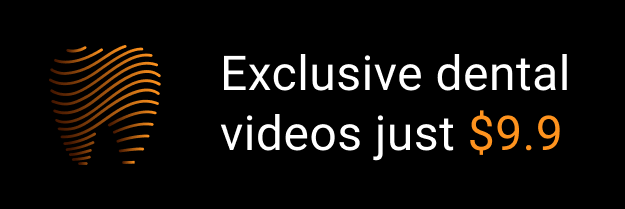