Highlights
- •
Grinding dental zirconia with tungsten carbide burs causes a significant reduction of the biaxial flexural strength.
- •
This reduction was attributed to ultra-structural subsurface micro-cracks observed to have penetrated into the material.
- •
Rubber polishing or air-particle abrasion of tungsten carbide-ground zirconia partially restored its biaxial flexural strength.
- •
Tungsten carbide burs are not recommended for grinding dental zirconia.
Abstract
Objectives
This study investigated the effects of tungsten-carbide grinding on the surface characteristics and mechanical strength of dental 3 mol% yttria-stabilized zirconia (3Y-TZP).
Methods
Two types of tungsten-carbide burs (TC), 6-blade ( TC 1 ) and 8-blade ( TC 2 ) were used to grind 3Y-TZP, in a dental air-turbine handpiece with water-cooling and were also subjected to air-particle abrasion (APA): TC 1 + APA and TC 2 + APA ; and rubber polishing (RP): TC 1 + RP and TC 2 + RP ; one group received only rubber-polishing RP . The control group received no treatment. Surface characterization was examined by surface roughness (R a ) and atomic force microscopy. Specimens were also observed with scanning electron microscopy (SEM) and X-ray-diffraction (XRD) for microstructure and crystalline phases. A piston-on-three-balls biaxial-flexural strength (BFS) test was performed with 15 samples-per-group and the broken specimen were observed under SEM to investigate the fracture origin pattern. One-way ANOVA, Kruskal–Wallis test and Weibull analysis were performed at α = 0.05.
Results
Groups TC 1 and TC 2 had the lowest mean BFS (p < 0.05) with up to 74 % reduction in strength. APA and RP both significantly increased the mean BFS after tungsten-carbide grinding but was still less than the control (p < 0.05). Compared to the control , the mean BFS was significantly reduced for all groups except for the RP group (p < 0.05). APA and rubber-polishing following TC 2 grinding had significant higher mean BFS than those following TC 1 grinding respectively (p < 0.05). SEM revealed distinct micro-cracks after tungsten-carbide grinding.
Significance
Tungsten-carbide burs (6- and 8-blade) are not recommended for zirconia grinding due to the significant reduction of biaxial-flexural strength and observed micro-structural surface and subsurface damage.
1
Introduction
The use of yttria-stablized tetragonal zirconia polycrystal (Y-TZP) for indirect dental restorations has increased dramatically in recent years due to its aesthetics, mechanical strength [ ], biocompatibility [ ] and ability for computer-aided design and computer-aided manufacture (CAD/CAM) restorations [ ]. Part of the enhanced strength characteristics of Y-TZP is its t-m phase transformation toughening where the tetragonal phase t to monoclinic phase m transformation [ ] produces a volumetric increase (∼4 %) under stress. This is considered to compress cracks in order to resist propagation thereby improving the flexural strength [ , ]. The phase transformation may be triggered by the external stresses such as thermal aging, grinding and sandblasting. Grinding, together with other surface treatments such as air-particle abrasion (APA) [ ], can cause t–m transformation thus preventing local growth of cracks. However, some studies have not observed any transformation after grinding dental zirconia with diamond burs or tungsten carbide burs [ ]. Surface flaws from grinding and sandblasting may act as stress concentrators and cause strength degradation [ ].
There is now a move towards monolithic zirconia restorations due to frequent chipping and fracturing of porcelain veneered dental zirconia frameworks during functional use. Despite the accuracy of CAD/CAM [ ], newly fabricated indirect dental restorations often require grinding and polishing to ensure a good fit and occlusal relation before permanent cementation. These procedures can cause roughness or defects, which can negatively affect the mechanical properties, ageing and wear resistance [ , ].
Grinding with tungsten carbide burs has been shown to have adverse effects on the strength attributed to the production of surface micro-cracks during grinding [ , ]. The use of APA after grinding has been shown to restore the BFS of these samples back to that of the control (as sintered) and this was attributed to the elimination of micro-cracks from tungsten carbide grinding [ ]. However, APA produces a rougher surface which may increase plaque retention or antagonist wear [ , ]. Therefore, there is a need to consider alternate ways to finish tungsten carbide ground zirconia that can remove surface micro-cracks and restore the strength. Polishing is a known method to smoothen ceramics thereby eliminating the defects created by grinding and in turn prevent or minimize wear of the opposing teeth [ ]. It is not known if rubber polishing has the potential to remove surface micro-cracks caused by using tungsten carbide burs for grinding. This study aimed to evaluate the effects of tungsten carbide grinding, with or without air-particle abrasion or rubber polishing on the surface characterization, biaxial flexural strength and fracture pattern of a dental 3Y-TZP (3 mol% yttria-stabilized zirconia).
2
Methods
2.1
Specimen preparation
Cylinders of pre-sintered 3Y-TZP were cut from disc blanks LAVA Plus high translucency zirconia, LOT: 3343987 and 3706728; 3M ESPE, Neuss, Germany using a trephine with 25 mm internal diameter HSS Hole Saw Bi-Metal, Starrett, United Kingdom. They were mounted onto the stainless steel fastener vise on a precision saw Isomet 5000, Buehler GmbH, Dusseldorf, Germany and then cut wet with a diamond blade Isomet 20LC, Buehler GmbH, Dusseldorf, Germany to produce disc specimens measuring ∼1.1 mm thick and ∼25 mm diameter.
After cutting, both sides of the disc specimens were hand-polished with a sequence of 600-, 800- and 1200-grit silicon carbide abrasive papers under water and were allowed to dry for 2 days before sintering. Specimens were sintered in a furnace (HT-S 9500, Mihm-Vogt GmbH, Stutensee, Germany) according to manufacturer’s recommendations to a temperature of 1450 °C for 2 h and then allowed to cool to room temperature. Sintered specimens (⌀ 20 mm × 0.85 mm ± 0.05 mm in thickness) were measured with a Vernier caliper (ABS Digimatic Caliper, 500 series, Mitutoyo, Japan) with an accuracy of ±0.01 mm, and unqualified samples were discarded.
2.2
Sample size determination and power analysis
For a sample size of 15 [ ] power analysis program (G*Power version 3.1.9.2; Franz Faul, Universitat Kiel, Germany) showed that 0.72 (i.e. medium to large) effect size difference between two groups can be detected, with 80 % power and at significance level of 0.05. In addition, three specimens were needed for scanning electron microscopy (SEM), and X-ray diffraction (XRD) analysis.
2.3
Surface treatments
Specimens were randomly distributed to eight groups (n = 18) and subjected to different surface treatments ( Table 1 ). Control ( C ) group was as sintered (no treatment). Three groups ( TC 1 , TC 1 + APA, TC 1 + RP ) were ground with a 6-blade round plain cut tungsten carbide burs (TC) ( Table 2 ) while three groups ( TC 2 , TC 2 + APA , TC 2 + RP ) were ground with an 8-blade finishing chamfer tungsten carbide burs. All grinding was performed with a dental air turbine handpiece (TA-98L, Synea HS, W&H, Austria) running at full speed (360,000 rpm) under water coolant, and was confined to a defined area 4 mm by 4 mm in the center of the specimen. Specimens were ground at a force of 80 g of loading for 20 s in a continuous forward and backward motion in one direction in the defined area and were placed on a digital weighing scale to monitor and control the force applied. Pilot testing found that 100 g loading force [ ] tended to be heavier than normal hand grinding force with a slight tendency for the bur to slow. After grinding every three specimens, the bur was discarded to maintain similar cutting efficiency.
Grinding | Treatments | Code |
---|---|---|
TC 1 | TC 1 | |
TC 1 | APA | TC 1 + APA |
TC 1 | Rubber polishing | TC 1 + RP |
TC 2 | TC 2 | |
TC 2 | APA | TC 2 + APA |
TC 2 | Rubber polishing | TC 2 + RP |
None | Rubber polishing | RP |
None | As sintered (Control) | C |
Burs or rubber polishing kits | ISO | Manufacturers | Handpiece |
---|---|---|---|
Tungsten carbide burs Round Plain Cut RA, 6-blade | 6.018 | UnoDent (United Kingdom) | High-speed |
Tungsten carbide burs parallel chamfer, 8-blade | H282.314.010 | Komet Dental Bur Gebr, Komet, Brasseler GmbH & Co (Lemgo, Germany) | High-speed |
StarGloss Diamond Porcelain Polishers | Edenta AG (Switzerland) | Low-speed | |
1. Blue wheel R1020HP | 803.104.372.533.170 | ||
2. Pink wheel R1030HP | 803.104.372.523.170 | ||
3. Grey wheel R1040HP | 803.104.372.513.170 |
Air particle abrasion of 50 μm alumina particles (Cobra, Renfert GmbH, Hilzingen, Germany) was performed for 10 s with the sandblaster (Basic Quattro IS, Renfert GmbH, Hilzingen, Germany) perpendicular and 10 mm to the specimen surface under 4 bar pressure [ ]. Three groups ( TC 1 + RP, TC 2 + RP, RP ) were treated by a 3-step rubber polishing (RP) procedure ( Table 2 ) using a dental straight handpiece at full speed 30,000 rpm (Ultimate-XL, NSK, Japan) for 2 min in total following the manufacturer’s instruction. All specimens were stored in deionized water for 24 h at 37 °C prior to a load-to-failure.
2.4
Surface characteristics
The surface roughness R a (μm) was measured before and after surface treatments with a profilometer (Surtronic3+; Taylor Hobson, Leicester, United Kingdom — tip radius 5 μm) for all specimens. The profilometer was calibrated before use. For each specimen, three different locations near the center of the specimen were measured and the average was obtained.
After surface treatments, one specimen per group was sputter-coated with gold and analyzed with a field-emission scanning electron microscope (SU1510, Hitachi, Japan) at 15.0 kV. Images were captured at magnifications of 500×, 2000× and 5000×.
2.5
Biaxial flexural strength
Biaxial flexural strength (BFS) was measured with a universal testing machine (E3000, Instron Ltd., England) according to ISO 6872/2015 [ ]. Fifteen specimens per group were subjected to a piston-on-three-ball test with the treated surfaces positioned on the tensile side. The center of three balls (⌀ = 2.0 mm) were 5.0 mm apart. Specimens were ultrasonically cleaned in water for 30 s and dried thoroughly before loading. An adhesive tape was fixed on the compressive side of the discs to retain the fragments and enhance the contact between piston and specimen. A flat loading pin (⌀ = 1.0 mm) was used to load the specimen at a crosshead speed of 1.0 mm/min until failure. The BFS was the maximum center tensile stress (MPa) and calculated by the following equation:
BFS=-0.2387P(X-Y)b2
where P is the breaking force i.e. total load causing fracture (N); <SPAN role=presentation tabIndex=0 id=MathJax-Element-2-Frame class=MathJax style="POSITION: relative" data-mathml='X=1+νln(r2r3)2+(1-ν2)(r2r3)2;Y=1+ν[1+ln(r1r3)2]+(1-ν)(r1r3)2′>?=(1+?)ln(?2?3)2+(1−?2)(?2?3)2;?=(1+?)[1+ln(?1?3)2]+(1−?)(?1?3)2X=1+νln(r2r3)2+(1-ν2)(r2r3)2;Y=1+ν[1+ln(r1r3)2]+(1-ν)(r1r3)2
X = 1 + ν ln ( r 2 r 3 ) 2 + ( 1 – ν 2 ) ( r 2 r 3 ) 2 ; Y = 1 + ν [ 1 + ln ( r 1 r 3 ) 2 ] + ( 1 – ν ) ( r 1 r 3 ) 2
; b is the specimen thickness at fracture origin (mm); ν is Poisson’s ratio (0.25 for dental ceramics); r 1 is the radius of support circle (mm); r 2 is the radius of loaded area (mm); r 3 is the radius of specimen (mm).
2.6
Crystalline phases
The crystalline structures of representative specimens were examined by an X-ray diff ;ractometer (XRD) (D8 Advance, Bruker AXS, GmbH, Karlsruhe, Germany) with a Lynxeye detector at 40 kV and 40 mA with Cu Kα radiation at room temperature. The 2 θ scan range was from 10° to 70° with a step size of 0.02° and a counting time of 1 s. The obtained XRD patterns were used to identify the mineral phases on the specimens under different surface treatments. The diffraction peaks observed in the analyzed XRD patterns were identified by comparing with the standard patterns from Power Diffraction File database of International Center for Diffraction Data [ ].
2.7
Fractographic analysis
After the biaxial flexural strength test, specimens were analyzed by scanning electron microscope (SU1510, Hitachi, Japan) at 15.0 kV. Images were obtained at 50×, 100× and 200× magnification to determine the fracture pattern.
2.8
Data analysis
The results of this study were statistically analyzed with statistical analysis software (IBM SPSS Statistics 23.0 for Windows, IBM, USA). A descriptive analysis was performed to determine the mean and standard deviation of the surface roughness (R a ), specimen thickness and BFS. Normal distribution of continuous variables was evaluated by Shapiro–Wilk test. For roughness, one-way ANOVA with post-hoc Tukey test was performed. For thickness and BFS, non-parametric Kruskal–Wallis test with stepwise step-down comparisons were performed. The level of significance was set as 0.05. Pearson correlation analysis was also performed to determine the association between roughness and the biaxial flexural strength parameters such as number of fragments.
The Weibull analysis consists of a scale (σ θ ) and shape ( m ) parameters, and is determined using linear regression according to methods described by Quinn and Quinn [ ]. The scale parameter (Weibull characteristic strength) corresponds to the 63.2 % failure probability whereas the shape parameter (Weibull modulus) indicates the homogeneity of the strength data thus expressing reliability of the material.
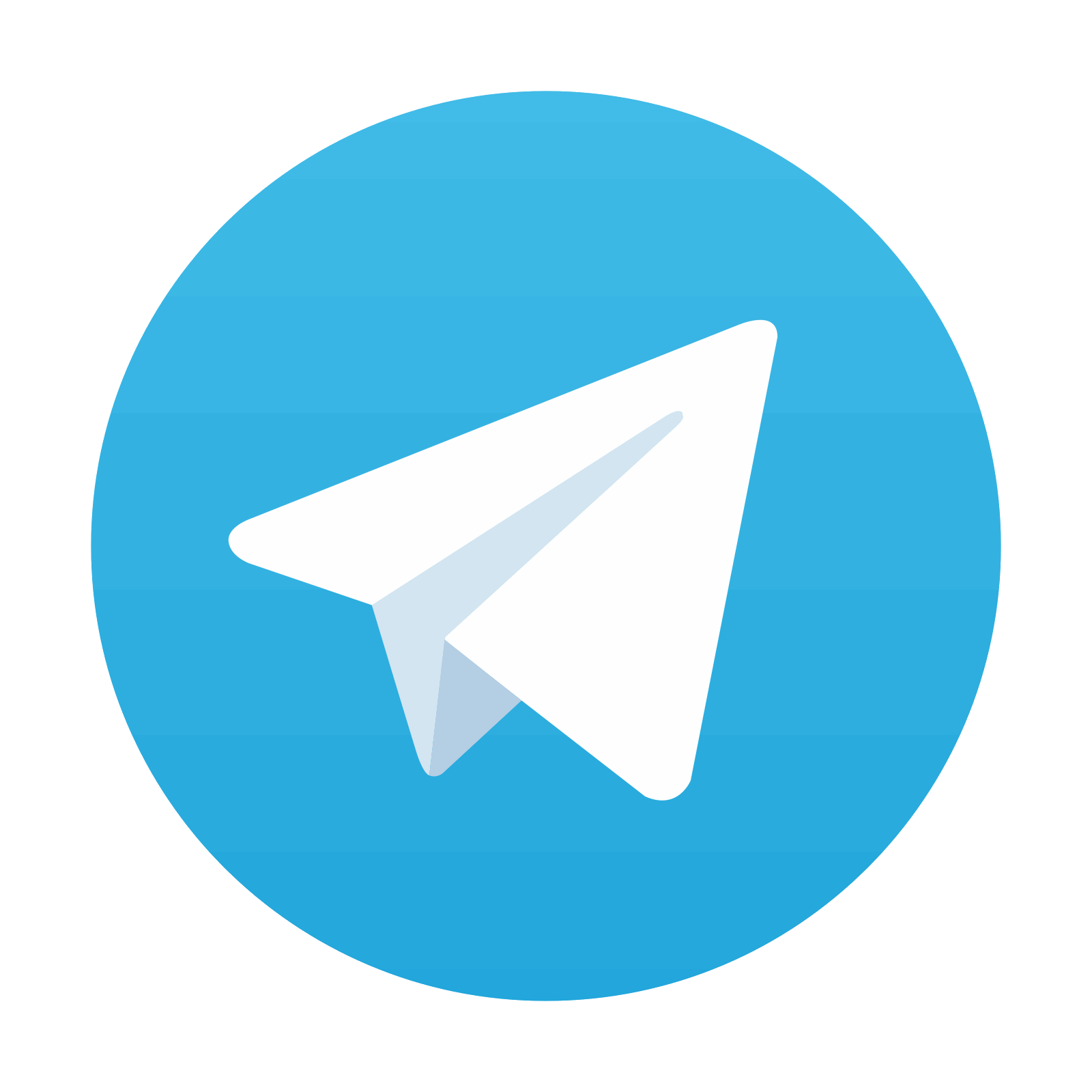
Stay updated, free dental videos. Join our Telegram channel

VIDEdental - Online dental courses
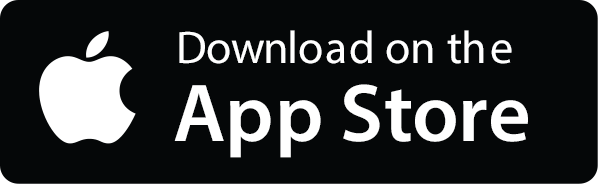
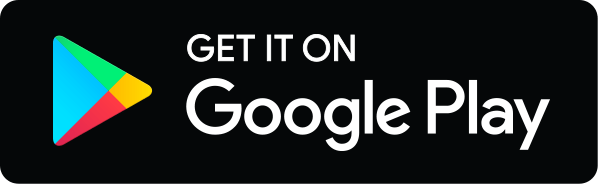