Highlights
- •
Occlusal veneers can be reliably produced by 3D-printing with the Lithography-based Ceramic Manufacturing process.
- •
3D-printed zirconia, milled zirconia and heat-pressed lithium disilicate can be recommended as material for ultra-thin occlusal veneers.
- •
These materials exhibit a fracture toughness surpassing the suggested values for posterior restorations if bonded to dentin.
Abstract
Objectives
The load-bearing capacity of ultra-thin occlusal veneers made of 3D-printed zirconia were compared to the ones obtained by fabricating these reconstructions by CAD/CAM milling zirconia or heat-pressing lithium-disilicate.
Methods
On 60 extracted human molars, the occlusal enamel was removed and extended into dentin. Occlusal veneers of 0.5 mm thickness were digitally designed. The specimens were divided into 3 groups (n = 20 each) differing in the restorative material and the fabrication technique of the occlusal veneer. (1) 3DP: 3D-printed zirconia (Lithoz); (2): CAM: milled zirconia (Ceramill Zolid FX); (3) HPR: heat-pressed lithium disilicate (IPS e.max Press). After conditioning procedures, the restorations were adhesively bonded onto the conditioned tooth. Thereafter, all specimens were aged in a chewing simulator by exposure to cyclic fatigue and temperature variations. Subsequently the specimens were statically loaded and the load which was necessary to decrease the maximum load by 20% and initiate a crack (F initial ) and the load which was needed to fracture the specimen (F max ) were measured. Differences between the groups were compared applying the Kruskal-Wallis (KW) test and the Wilcoxon-Mann-Whitney-Test (WMW: p < 0.05).
Results
The median F initial values for the groups 3DP, CAM and HPR were 1’650 N, 1’250 N and 500 N. The differences between all three groups were statistically significant (KW: p < 0.0001). The median F max values amounted to 2’026 N for the group 3DP, 1’500 N for the group CAM and 1’555 N for the group HPR. Significant differences were found between 3DP and CAM (WMW: p = 0.0238).
Significance
Regarding their load-bearing capacity, 3D-printed or milled zirconia as well as heat-pressed lithium disilicate can be recommended as restorative material for ultra-thin occlusal veneers to prosthetically compensate for occlusal tooth wear. Despite statistically significant differences between the restoration materials, all load-bearing capacities exceeded the clinically expected normal bite forces.
1
Introduction
Extensive tooth wear and/or erosive substancescan result in loss of occlusal tooth substance. A prosthetic rehabilitation may be necessary to compensate the lost tooth substance and to eliminate the associated symptoms. Traditional treatment concepts propose to restore the worn dentition by means of full-crown restorations [ ]. However, these concepts involve a further loss of healthy tooth substance by extensive preparation of the already impeded dentition [ ]. Nowadays, ultra-thin occlusal veneers represent an appropriate treatment alternative in cases of extensive erosive or abrasive tooth substance loss [ ]. Zirconia and lithium disilicate ceramic are both eligible materials for these kind of minimally invasive restorations [ ].
With the introduction of computer-aided design/computer-aided manufacturing (CAD/CAM), the processing of the high-strength ceramic zirconia has become possible [ ]. Zirconia reconstructions are usually milled out of pre-fabricated zirconia blanks by subtractive manufacturing. The milling process is commonly performed using a pre-sintered condition of the zirconia blank. In this state, zirconia has a low inherent strength and the fabrication of ultra-thin reconstructions can be challenging [ ]. More lately, additive manufacturing techniques have been introduced to fabricate high strength ceramics by lithography-based ceramic manufacturing (LCM) [ ]. This technique allows to fabricate complex three-dimensional structures with high accuracy [ ]. By 3D printing zirconia, restorations in a high resolution and thin walls can be produced [ ]. The fabrication of heat-pressed lithium disilicate reconstructions exhibiting thin wall thicknesses is a regular procedure and showed a good reliability [ , ].
Both materials, zirconia and lithium disilicate, demonstrate an improved fracture toughness and higher flexural strength in comparison to conventional glass ceramics [ , ]. A study compared the mechanical performance of occlusal veneers made out of either zirconia or lithium disilicate [ ]. It was found that being bonded to dentin, the load-bearing capacity of lithium disilicate is about 57% of the load-bearing capacity of zirconia [ ]. Accordingly, another study revealed a median load-bearing capacity of 2’493 N for zirconia and 1’165 N for lithium disilicate restorations in 0.5 mm thickness when bonded to dentin [ ]. The results of both groups surpassed the suggested fracture toughness for posterior reconstructions by far [ ]. Thus, both materials are mechanically appropriate for the use as ultra-thin occlusal veneers in the posterior region.
Up to now, there is no study available in the literature, comparing the mechanical performance of 3D-printed occlusal veneers made of zirconia to more traditionally applied fabrication techniques using conventional ceramic materials. Therefore, the objective of this study was to test whether the load-bearing capacity of ultra-thin 3D-printed zirconia occlusal veneers on molars exhibit differing load-bearing capacities if compared to CAD/CAM milled zirconia or pressed lithium-disilicate ceramic reconstructions.
2
Material and methods
2.1
Groups
The groups differed in the used restorative material ( Table 1 ) and the fabrication technique. In total, 3 groups of 20 specimens each (n = 20) were included: (1) “3DP”: 3D-printed occlusal veneers made out of zirconia (Lithoz, Vienna, Austria); (2) “CAM”: CAD/CAM-fabricated occlusal veneers milled out of zirconia (Ceramill Zolid FX; Amann Girrbach, Pforzheim, Germany); (3) “HPR”: heat-pressed occlusal veneers made out of lithium-disilicate ceramic (IPS e.max press; Ivoclar Vivadent, Schaan, Liechtenstein).
Group | Restorative material | Chemical composition |
---|---|---|
3DP | Zirconia (Lithoz, Wien, Austria) | ZrO 2 + HfO 2 + Y 2 O 3+ Al 2 O 3 (99.95 wt%), Y 2 O 3 (5,4–5,8 wt%), Al 2 O 3 (0.15–0.35 wt%), |
CAM | Zirconia (Ceramill zolid FX, Amann Girrbach, Pforzheim Germany) | ZrO 2 (90.4–94.5 wt%), Y 2 O 3 (4–6 wt%), HfO 2 (1.5–2.5 wt%), Al 2 O 3 (0–0.3 wt%), Er 2 O 3 (0–0.5 wt%), Fe 2 O 3 (0–0.3 wt%) |
HPR | Lithium disilicate ceramic (IPS e.max Press; Ivoclar Vivadent, Schaan, Liechtenstein) | SiO 2 (57–80 wt%), Li 2 O (11–19 wt%), K 2 O (0–13 wt%), P 2 O 5 (0–11 wt%), ZrO 2 (0–8 wt%), ZnO (0–8 wt%), other oxides and ceramic pigments (0–10 wt%) |
2.2
Specimen preparation
In total, 60 extracted and intact human molars were inserted in an acrylic hollow cylinder made out of acrylic glass. The apical part of the tooth was embedded in self-curing resin (Technovit 4071; Kulzer, Wasserburg, Germany). To create typical defects for attrition or erosion, the occlusal enamel of the crown was removed until exposure of the dentin. Furthermore, fissures were slightly opened and sharp edges were rounded off. The specimens were randomly allocated to one of the study groups. During the complete study, the specimens were stored in distilled water.
2.3
Scanning procedures and restoration design
An optical impression of the prepared tooth was taken by means of a desktop scanner (Identica 3D Scanner; Dental Concept Systems, Ulm, Germany) and transmitted to a design software (3Shape software; Copenhagen, Denmark). Occlusal veneers were designed with a homogenous thickness of 0.5 mm ( Fig. 1 ).

2.4
Fabrication of the restorations
According to the group allocation, the ceramic restorations were fabricated.
2.4.1
Group 3DP: fabrication procedures
The occlusal veneers of the group 3DP were fabricated by means of the Lithography-based Ceramic Manufacturing (LCM) process, which is based on the concept of photopolymerization ( Fig. 2 ) [ , ]. Ceramic powder was dispersed into a mixture of photo-curable monomers to create the ceramic slurry (LithaCon 3Y 610 white; Lithoz, Vienna). The materials processed by LCM-technology were slurries comprising a photopolymerizable monomer mixture (dynamic viscosity at 20 °C is 43 Pa s) filled with various types of ceramic powder (3 mol% Y 2 O 3 stabilized ZrO 2 in a purity of 99.9%) in a concentration of 40–60 Vol%. A thin layer of this slurry was automatically coated onto the vat, which is an assembly with transparent glass bottom. Thereafter, the building platform approached the vat, only leaving a small gap of 25 μm, which was filled with slurry. This gap correlates to the thickness of an individual layer in the green part. Consecutively, the photosensitive compounds comprised within this slurry were cured by selective exposure with blue light (wavelength 460 nm). Where this light hit the ceramic-filled slurry, the monomers photopolymerized into a 3-dimensional network, which then acted as a cage for the ceramic filler. After completing the layer, the building platform was elevated and the whole sequence was repeated all over again. The occlusal veneers were layered perpendicular to the tooth axis. The scaling factor was in XY-direction 1.358× and in Z-direction 1.370× to compensate for the sinter-shrinkage. After the layer-by-layer structuring using the CeraFab 7500-system (Lithoz, Vienna) the green parts were cleaned from the excess slurry by using compressed air and an appropriate solvent (LithaSol 20, Lithoz) capable of dissolving the slurry without damaging the cured structure. Postprocessing involved the debinding of the green part in which the organic photopolymer matrix was removed through pyrolysis at stepwise raising temperatures between 115 and 1’450 °C. Then, the resulting white parts were sintered in a high-temperature (1’450 °C, 2 h) furnace (Nabertherm HTCT 08/16; Nabertherm, Lilienthal, Germany) to fully density (99.3%). Thereafter, the supporting structure was cautiously removed ( Fig. 3 ).


2.4.2
Group CAM: fabrication procedures
The ceramic restorations of the group CAD were directly milled out of pre-fabricated zirconia discs (Ceramill Zolid FX; Amann Girrbach) using a 5-axis milling machine (Ceramill Motion2 5×; Amann Girrbach). Thereafter, the restorations were sintered to full density according to the manufacturer’s instructions (Ceramill Therm S; Amann Girrbach).
2.4.3
Group HPR: fabrication procedures
For the ceramic restorations of the group HPR, first, a PMMA template was milled out of a pre-fabricated ingot (Ceramill PMMA; Amann Girrbach) by means of a 5-axis milling machine (Ceramill Motion2 5×; Amann Girrbach). The templates were then used to produce pressed lithium disilicate restorations applying the “lost-wax and press-technique” and following the manufacturer’s instructions. For this purpose, the PMMA-templates were fixed by a wax sprue (IPS Multi Wax Pattern Form A; Ivoclar Vivadent) and vested (IPS PressVEST Premium; Ivoclar Vivadent) into a mold. In an oven (KaVo EWL 5645; KaVo, Kloten, Switzerland), the vested templated was heated to complete dissolution: rate of 5 °C min −1 from room temperature to 850 °C (holding time 60 min). Thereafter, into the resulting void, lithium-disilicate ceramic (IPS e.max Press; Ivoclar Vivadent) was heat-pressed in a heat-pressing sintering-oven (Programat EP 5010; Ivoclar Vivadent): rate 60 °C min −1 from 700 °C to 898 °C (holding time 25 min). After cooling, the restorations were carefully devested and cleaned from the investing material by air-abrasion (50 μm Al 2 O 3 ; Cobra, Renfert GmbH, Hilzingen, Germany) at a pressure of 2 bar. The surface was glazed and again placed into the sintering-oven.
2.5
Cementation protocols
The conditioning procedure of the inner surface of the ceramic restoration varied according to the groups. In group HPR, etching for 20 s with 5% hydrofluoric acid (IPS ceramic etching gel; Ivoclar Vivadent) followed by water-spraying and air-drying. In groups 3DP and CAM, air-abrasion was applied to the inner parts (Rocatec Plus 30 μm, 2.5 bar; 3 M ESPE, Seefeld, Germany). In all groups, a silane (Monobond Plus; Ivoclar Vivadent) was used and gently air-dried after 60 s. The enamel and the dentin of all specimens were etched with 35% phosphoric acid for 30 s (Ultraetch; Ultradent, Utah, USA). The surface was thereafter water-sprayed (30 s) and air-dried. An adhesive (Syntac Primer/Syntac Adhesive; Ivoclar Vivadent) was used for the dentinal parts. A bonding agent (Heliobond; Ivoclar Vivadent) was applied to the tooth surface and to the ceramic restorations and after 20 s gently air-blown. The restorations were adhesively cemented using a dual-curing resin cement (Variolink Esthetic DC; Ivoclar Vivadent). After removal of excess cement, light-curing was performed (6 × 40 s).
2.6
Aging procedures
A chewing simulator [ ] was used to age the specimens by a vertical indenter (rounded tip of ∅ 8 mm) executing a vertical movement of 1 mm in a perpendicular direction to the occlusal plane (1’200’000 cycles of 49 N force at 1.67 Hz loading frequency) and thermo-cycling (5–55 °C and a dwelling time of 120 s). After aging, the specimens were evaluated under a stereomicroscope (magnification 1.25×) to check for integrity.
2.7
Static loading
The aged specimens were loaded in a universal testing machine (Zwick/Roell Z010; Zwick, Ulm, Germany) to test the static fracture load. An indenter axially hit the occlusal surface of the specimen with a crosshead speed of 1 mm/min. The load which was necessary to initiate a crack (F initial ) and the load which was needed to completely fracture the specimen (F max ) were measured. The type of failure was specified under a stereomicroscope (9× magnification; Leica DFC300 FX; Wetzlar, Germany) and on photographs. The following scores were categorized: (1) score 0 = no visible fracture, (2) score 1 = cohesive fracture within the restoration, (3) score 2 = cohesive fracture of the restoration and of the cement layer, (4) score 3 = fracture of the restoration-cement-tooth complex.
2.8
Statistical analysis
The metric variables (F initial , F max ) were described with mean, median, standard deviations, quartiles, minimum and maximum. They were compared using a non-parametric Kruskal–Wallis test (KW). The exact p-values were calculated for the pair-wise comparisons between the groups using the Wilcoxon–Mann–Whitney-Test (WMW), applying the Bonferroni correction for the multiple testing.
The categorical variables (failure scores) were summarized by counts and proportions of the categories and compared applying the Chi–squares test with exact determination of the p-value.
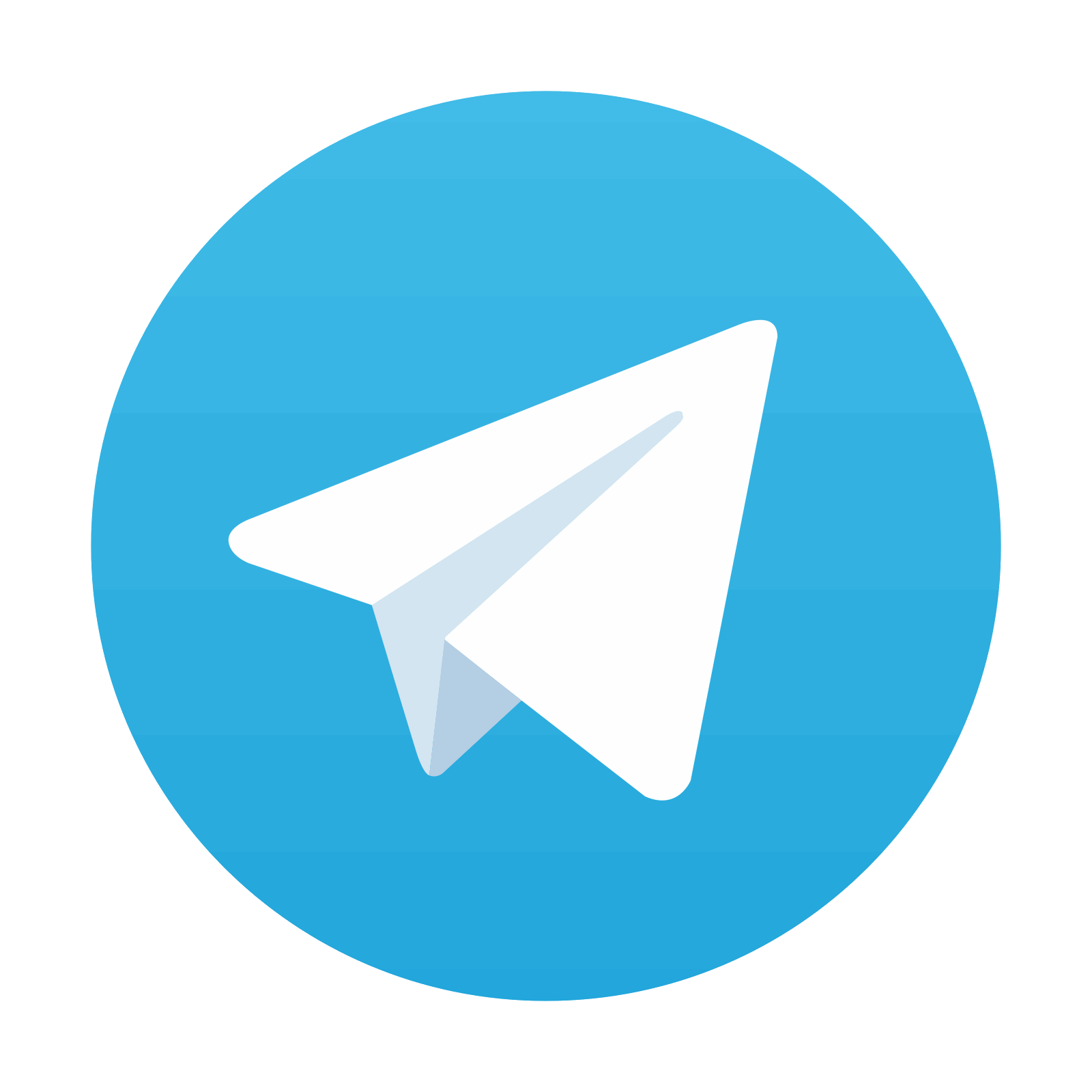
Stay updated, free dental videos. Join our Telegram channel

VIDEdental - Online dental courses
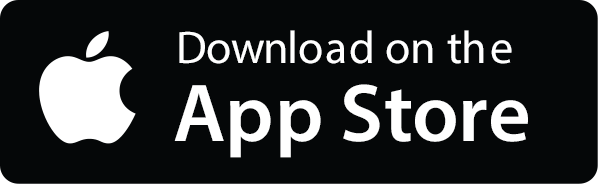
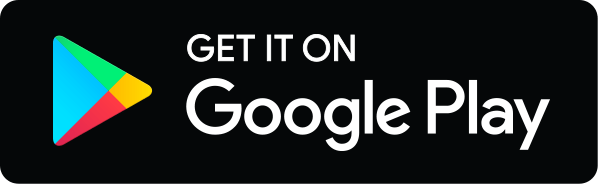