Abstract
Objective
This study investigated the effect of plasma-treated leno weaved ultra-high-molecular-weight polyethylene fiber placement on gap formation and microtensile bond strength (MTBS) of a bulk-fill composite in deep cavity.
Methods
Resin composite molds (3 mm width, 4 mm depth) were treated with Clearfil SE Bond 2 and restored with 3 techniques : (1) Surefil SDR flow (SDR) placed in bulk (BLK), (2) SDR placed in two unequal increments (INC) and (3) SDR placed after an increment of SDR placed with wetted polyethylene fiber (Ribbond Ultra) at the cavity floor (FRC). As a control, the cavities were bulk-filled with SDR and no bonding agent (n = 12). All the specimens were subjected to real-time and 3D imaging by SS-OCT (1330 nm) to calculate the total volume of gap formed (mm 3 ) at the cavity floor and between the composite increments. For MTBS, the occlusal cavities of the similar dimensions (3 × 3 × 4 mm 3 ) were prepared on extracted molars with similar composite placement techniques (BLK, INC and FRC). After 24 h 37 °C water storage, the specimens were sectioned using a diamond saw to create 0.7 × 0.7 mm 2 beams for MTBS, and subjected to bond testing at a crosshead speed of 1 mm/min. Data for both tests was analyzed by one-way ANOVA and multiple-comparisons with Bonferroni correction (α = 0.05).
Results
The gap volumes were different among the groups (p < 0.05). The largest cavity floor gaps (mm 3 ) were observed in the control group (2.00 ± 0.08); followed by BLK (0.74 ± 0.20) and INC (0.02 ± 0.01). In FRC, the cavity floor was gap-free in all specimens but some separation was observed between the two increments. MTBS values (MPa) were 13.8 ± 7.6, 31.7 ± 12.5 and 28.3 ± 8.5 for BLK, INC and FRC groups. There was no significant difference between FRC and INC and both were different from BLK (p < 0.05).
Significance
Gap formation of the bulk-fill composite at cavity floor was significantly reduced with the placement of a fiber-reinforced increment at the base of the deep preparation. The fiber-reinforced increment acts as a shrinkage stress breaker and protects the bonded interface at deep dentin.
1
Introduction
Resin composite has witnessed a rapid growth in utilization for posterior teeth to correspond to aesthetic and functional demand [ ]. Adhesive systems are used to provide efficient bond between resin composites and the tooth structure, which is critical for the success and longevity of aesthetic restorations [ ]. Many factors such as the bonding substrate, bonding area, preparation geometry, technique of composite application, light curing efficiency and occlusal force equilibration contribute to gaining clinical success with direct posterior resin restorations [ ]. The shrinkage of methacrylate-based composite resins as direct result of polymerization is a widely researched phenomenon in literature [ ]. The shrinkage inevitably occurs as the resin monomers in the paste form move closer to each other and link covalently to form the polymer.
If the internal stresses arising from shrinkage forces exceed the resisting forces at the cavity internal surfaces, it would lead to the loss of sealing at the interface between the tooth and composite [ ]. The polymerization shrinkage stress in composite resins may result in marginal disintegration, cuspal deflection, enamel crack formation, reduced bond strength, compromised mechanical properties, and interfacial gaps between composite and tooth structure, all of which are factors accounting for the success or failure of a composite restoration. The stress could be strong enough to immediately initiate a crack within the restorative material itself [ ].
Modifying restorative techniques may reduce the stress of polymerization shrinkage. It is not clear which restorative technique, if any, is the most appropriate one to eliminate shrinkage stress. Incremental application of composite instead of a bulk technique has been proposed to reduce stress of shrinkage in cavities [ ]. However, the incremental technique in restoring deep preparations is time-consuming and relatively technique sensitive [ ], and some reports have even proposed that they have little effect on reducing the total shrinkage stress [ ]. Recently, bulk-fill composites, which can be inserted as a single increment layer 4–5 mm in thickness have been proposed as an alternative to simplify the restorative procedure and decrease polymerization shrinkage stress [ ]. Provided that the quality of the resulting restoration is comparable with or surpasses that of the incremental technique, the bulk-fill composite would seem to be a preferred technique for restoring deep cavities [ ].
Nevertheless, bonding to cavity floor dentin may be challenging in large deep composite restorations, even using state-of-the-art bulk-fill composites [ ]. Using optical coherence tomography (OCT) imaging technique, Hayashi et al. reported that all the tested light-cured bulk-fill composites lost adaptation to a 4-mm deep cavity floor due to polymerization shrinkage [ ].
Since shrinkage is an intrinsic resin property, reducing resin volume by adding non-monomer components such as organic or non-organic fillers has been considered as an effective way to reduce the magnitude of shrinkage [ ]. Fiber-reinforced composite (FRC) is a material that carries such an effect on polymerization shrinkage, while enhancing physical properties of the composite and potentially acting as a crack stopping mechanism [ ]. The mechanical properties and reinforcing capacity of FRC used in dentistry depend on the fiber type, fiber orientation relative to the load, fiber position in the restoration, fiber volume and impregnation of the fiber to the resin matrix [ ].
The use of fiber-reinforced composites have been broadly evaluated in various studies [ ]. Chairside incorporation of ultra-high molecular-weight polyethylene fiber (UHMWPE) into resin composite has received a renewed attention for the direct restoration of structurally compromised teeth that require the use of a large amount of composite [ ]; however, the combination of UHMWPE fiber and bulk-fill composite resin in deep cavities has not been evaluated. The current study investigated whether an increment of composite placed with plasma-treated leno-weaved UHMWPE fiber (Ribbond Ultra, Ribbond Inc, Seattle, WA) at the base of a deep cavity affected the debonding of composite from the cavity floor, total gap formation in the composite and the microtensile bond strength (MTBS) of composite to deep cavity dentin. The null hypotheses were that the gap formation at the cavity floor and the bond strength were not affected by the placement technique.
2
Materials and methods
2.1
Experimental design
This study consisted of two major experiments: adaptation of the composite to 4-mm deep molds using OCT imaging and MTBS of the composite to deep dentin in a restored preparation in human teeth. The bulk-fill composite (SDR; SDR flow, Dentsply Sirona, DE, USA) was used in combination with the UHMWPE fiber.
2.2
Adaption to cavity floor
In order to standardize the cavity geometry and ensure adequate OCT signal intensity to observe composite adaptation at the cavity floor, a standard composite mold was created using flowable composite (Estelite Flow Quick, Tokuyama Dental, Tokyo, Japan) [ ]. The internal surfaces of the molds (3 mm width and length, 4 mm depth,) were sand-blasted with 50 μm alumina particles (MicroEtcher II; Danville Materials, Carlsbad, CA, USA) at 0.4 MPa for 15 s, followed by ultrasonic cleaning for 10 min (Model US 102; SND, Nagano, Japan), phosphoric acid cleaning (K-etchant gel; Kuraray Noritake Dental, Tokyo, Japan), silanization (Clearfil Ceramic Primer Plus; Kuraray Noritake Dental, Tokyo, Japan) and bond application (CSE; Clearfil SE Bond 2 Bonding Agent; Kuraray Noritake Dental, Tokyo, Japan). Light curing was proceeded for 20 s using a halogen light curing unit with 600 mW/cm 2 light irradiance (Optilux 501, Kerr, Orange, CA, USA) according to the manufacturer instruction. The specimens were allocated into 3 groups according to placement techniques (n = 12). In group 1 (BLK), SDR was placed with a syringe tip in bulk and cured for 20 s. In group 2 (INC), bulk-fill composite was applied in two unequal increments (approx. 0.3 mm and 3.7 mm in thickness) and each increment was light cured for 20 s. In group 3 (FRC), SDR was placed after an increment of composite reinforced with UHMWPE fiber as follows; a thin layer of SDR bulk-fill composite (similar to INC) was applied on the cavity floor with a syringe tip without curing. A 3 mm × 3 mm piece of fiber was cut and soaked in CSE bonding agent; the excess of the bonding was removed by gently tapping a dry micro brush on fiber, then the fiber was placed over the bulk-fill composite in the cavity and gently pushed through the uncured composite to create a FRC increment. The increment was manipulated with a non-sticking plastic hand instrument so that the layer of fiber was laminated as closely as possible against the cavity floor. This combination was cured for 20 s, creating an approximately 0.3 mm thick FRC increment. Finally, the cavity was bulk filled with SDR and cured for 20 s. As for a control group, the cavities were bulk-filled with SDR without any primer or bonding agent treatment of the mold.
2.3
OCT imaging and analysis
The swept-source OCT system (Prototype 2, Panasonic Healthcare Co., Ltd., Ehime, Japan) was used to monitor the internal integrity of the specimen during the light curing of all groups; BLK, INC, FRC and control group in 2D real-time videos as well as 3D imaging of the cavity after polymerization. The prototype system has been presented with details [ ]. A center wavelength at 1330 nm with a 30-kHz sweep rate was used in this system. The transversal resolution is 20 μm and axial resolution is 12 μm in air. It also provides a photographic image of the specimen. 3D scan was obtained after light curing at 256 × 256 × 1024 pixels over an XYZ volume of 5 × 5×7 mm 3 . The experimental set up for deep cavity imaging has been reported in detail [ ]. For analysis of the volume of gaps formed, the OCT 3D data were imported to Amira software (version 5.5.0, FEI Visualization Science Group). Using the labelling function of the 3D image analysis software and based on the increased reflectivity at the gap boundaries [ ], the gap volume was calculated in two stages in mm 3 ; first for the cavity floor only and then for the whole restoration, which included both the floor and the composite increments. The data were analyzed by one-way ANOVA for the two types of gaps; the cavity floor and the whole restoration.
2.4
Cross-sectional microscopy
Furthermore, confocal laser scanning microscopy (CLSM, 1LM21H/W, Lasertec Co., Yokohama, Japan) was used for cross-sectional microscopy. The specimens of each group were sectioned and mirror-polished up to 0.25 μm with diamond pastes in circular motion under cooling water to physically reach the axial cross-section imaged in 2D real-time videos. The cavity wall and floor on each cross section were observed at 1250 magnification using CLSM. The representative specimens were further sputter gold-coated and observed under scanning electron microscopy (SEM; JSM-6010PLUS/LA, JEOL, Tokyo, Japan).
2.5
Bond strength to cavity floor dentin specimens
For the MTBS test, the occlusal cavities were prepared on the human teeth. Twenty-four intact human mandibular molars were collected after extraction for periodontal, orthodontic or surgical reasons. Before proceeding the study, teeth were examined to ensure they were intact, non-restored and non-carious. From the time of extraction, teeth were stored in water at 4 °C for less than two months. The teeth were mounted in epoxy resin (Total Boat, Bristol, RI) and the cusps were sectioned with a low-speed diamond saw (Buehler, Lake Bluff, IL) under abundant cooling water to form an occlusal flat surface. To ensure reaching a 4-mm deep preparation without exposure of the pulp chamber or too thin remaining dentin thickness, a 1-mm thick layer of hybrid resin composite (AP-X, Kuraray Noritake Dental, Tokyo, Japan) was placed on the occlusal flat surface and cured for 20 s with CSE according to the manufacturer’s instructions.
An occlusal cavity (3 × 3×4 mm 3 ) was prepared in each tooth using a long flat-end cylindrical diamond bur (Shofu, Kyoto, Japan) under cooling water to obtain a flat cavity floor. The cavities were treated using CSE according to the manufacturer instructions and restored with 3 placement techniques similar to the cavity adaptation study to obtain 3 experimental groups: BLK, INC and FRC.
2.6
Microtensile bond test
After 24 h of storage in 37 °C water, teeth were sectioned using a low speed diamond saw under abundant cooling water to create 0.7 × 0.7 mm 2 beams. The marginal beams and beams with remaining dentin thickness of less than 2 mm were excluded from this study. Four central beam with no defects were selected for the MTBS test at a crosshead speed of 1 mm/min (Bisco Microtensile Tester, Schaumburg, IL). A bond strength value of zero was recorded for central beams with pre-test failure. For statistical analysis purposes, each tooth was considered as a statistical unit and therefore, all the values obtained from each tooth were averaged (n = 8). One-way ANOVA with post-hoc Tukey HSD was used to compare MTBS at a significance level of alpha = 0.05. After MTBS test, the dentin side of the beams was collected and the mode of failure was observed by SEM.
3
Results
The volumetric gap values are reported in Table 1 , and representative OCT images are presented in Fig. 1 . One-way ANOVA showed that the placement technique significantly affected the volume of gap formed after polymerization (p < 0.05). When considering the cavity floor only, the largest gap was seen in the control (unbonded) group. All other groups showed some gap formation at the cavity floor except for FRC, where the cavity floor was gap-free in all specimens. There was a significant difference between INC, BLK and control (p < 0.05). When the whole restoration void including the gap between increments was considered, there was no significant difference between FRC and INC (p > 0.05). No further voids were observed within the bulk-filled BLK and control groups. Real-time videos are presented online (BLK and FRC), with key screen shots in the video still. CLSM images ( Fig. 2 ) confirmed that in BLK, the separation occurred at the bonded interface while in FRC, it happened between the two increments. CLSM confirmed close adaptation of SDR to the fiber within the FRC increment, which appeared to be around 0.3 mm in thickness.
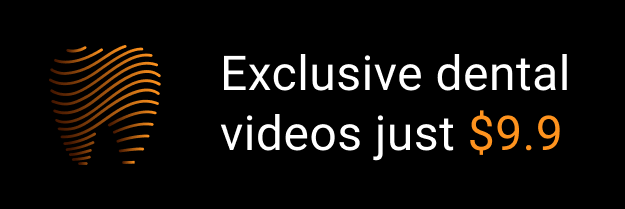