Introduction
The aim of this study was to examine the effects of orthodontic treatment with and without extractions on the anatomic characteristics of the upper airway in adults.
Methods
For this retrospective study, the pretreatment and posttreatment cone-beam computed tomography scans of 74 adult patients meeting defined eligibility criteria were analyzed. Imaging software was used to segment and measure upper airway regions including the nasopharynx, the retropalatal, and retroglossal areas of the oropharynx, as well as the total airway. The Wilcoxon signed rank test was used to compare volumetric and minimal cross-sectional area changes from pretreatment to posttreatment.
Results
The reliability values were high for all measurements, with intraclass correlation coefficients of 0.82 or greater. The volumetric treatment changes for the extraction and nonextraction groups were as follows: total airway, 1039.6 ± 3674.3 mm 3 vs 1719.2 ± 4979.2 mm 3 ; nasopharynx, 136.1 ± 1379.3 mm 3 vs −36.5 ± 1139.8 mm 3 ; retropalatal, 412.7 ± 3042.5 mm 3 vs 399.3 ± 3294.6 mm 3 ; and retroglossal, 412.5 ± 1503.2 mm 3 vs 1109.3 ± 2328.6 mm 3 . The treatment changes in volume or minimal cross-sectional area for all airway regions examined were not significantly ( P >0.05) different between the extraction and nonextraction groups.
Conclusions
Orthodontic treatment in adults does not cause clinically significant changes to the volume or the minimally constricted area of the upper airway. These results suggest that dental extractions in conjunction with orthodontic treatment have a negligible effect on the upper airway in adults.
Highlights
- •
Upper airway sizes were assessed in adults before and after orthodontic treatment.
- •
Nasopharynx, retropalatal, and retroglossal regions of the oropharynx were measured.
- •
Oropharynx measurements were similar in extraction and nonextraction patients.
- •
Extraction orthodontic treatment does not constrict the upper airway in adults.
The proposed benefits and negative sequelae associated with the extraction of teeth have long been debated in orthodontics. Dental extractions are typically used to provide space to align crowded teeth, reduce incisor protrusion, and correct anteroposterior interarch discrepancies. However, crowded teeth may also be aligned by dental expansion of the arches, although there are physiologic limits to this process. Despite a significant amount of research investigating the effects of extractions in terms of treatment stability, smile esthetics, temporomandibular joint health, and soft tissue profile, a consensus on when to extract teeth eludes the specialty.
More recently, the prevalence and health effects of obstructive sleep apnea have become more widely known among dental professionals. The discussion of the effects of extractions in orthodontics has shifted to include the volume and function of the upper airway. Anecdotally, severely constricted dental arches that result in the tongue crowding the oropharynx are posited as the link between the dentoalveolar anatomy and the airway. However, since altered dentofacial morphology is associated with obstructive sleep apnea in both children and adults, it is desirable to understand what impact, if any, orthodontic treatment with extractions may have on the airway.
Initial investigations on the effects of orthodontic treatment on the pharyngeal airway space have used lateral cephalometrics. One study found a reduction in the dimension of the pharyngeal airway space after orthodontic treatment with the extraction of 4 premolars, whereas others have found either no change in airway dimensions or differing results depending on the specific mechanics used during treatment. The significant limitation shared by these studies is that assessments were based on 2-dimensional lateral cephalographs; therefore, only the sagittal and vertical dimensions of the airway were evaluated.
With the advent of cone-beam computed tomography (CBCT) imaging, our understanding of airway morphology has been expanded to 3 dimensions to include the overall volume and, perhaps most physiologically relevant, the cross-sectional area perpendicular to the direction of airflow as visualized in the axial plane. Valiathan et al used CBCT to compare airway changes of 20 adolescents undergoing orthodontic treatment with premolar extractions with age-matched, nonextraction controls. They reported no difference in the oropharyngeal airway volume changes between the 2 groups despite differences in incisor angulations and protrusion. Other similar studies involving adolescents have also found no differences in dimensional changes in the airways between extraction and nonextraction orthodontic treatments. However, a potential confounding factor common among these studies has been the inclusion of growing subjects. Because the airway volumes of most patients in these studies was found to increase regardless of the treatment, it is possible that treatment effects were masked by ongoing growth. Therefore, it may be desirable to eliminate the confounding effect of growth in future studies. The aim of our study was to investigate the effects of orthodontic treatment with and without extractions on the volume and minimal cross-sectional area of the upper airway in a cohort of nongrowing adult patients.
Material and methods
Initial compilation of the study data consisted of application of screening criteria to all patients treated from September 2008 to June 2012 at the Division of Orthodontics, University of Minnesota, in Minneapolis, Minn. Initial eligibility criteria included at least 18 years of age at treatment start, complete pretreatment and posttreatment CBCT scans available, no missing teeth (excluding third molars), and a negative history of previous orthodontic treatment or orthognathic or airway surgery. Patients with a Class III skeletal relationship (ANB angle, <0°) or a history of cleft lip or palate or any craniofacial syndrome were also excluded. Within this time period, 202 patients were adults, and 74 met the eligibility criteria. Patients in the extraction group had at least 2 premolars extracted as part of their orthodontic treatment. For this sample, the pretreatment and posttreatment DICOM files were exported, and the following deidentified data were collected: age, sex, amounts of dental crowding in the maxillary and mandibular arches, amounts of overbite and overjet, treatment time, number of teeth removed, and orthodontic appliances used. The Research Ethics Board at the University of British Columbia (H12-00951) approved the study protocol.
All CBCT scans were taken using an i-CAT Next Generation CBCT unit (Imaging Sciences International, Hatfield, Pa) with a 17-cm field of view and scan times of 8.9 to 17.8 seconds. At the time of image acquisition, no specific instructions had been given to patients in regard to mode of breathing or tongue position beyond “remain perfectly still and breathe quietly through your nose.” DICOM files were imported into and analyzed using Dolphin software (version 11.5; Dolphin Imaging and Management Solutions, Chatsworth, Calif) for this study. Before we generated the lateral cephalometric and airway images, the 3-dimensional images were all standardized in orientation with the midsagittal plane determined from the skeletal midline of the face using a line connecting the incisive foramen to opisthion. The axial plane was adjusted with a line connecting the inferior border of the left and right orbits parallel to the horizontal grid. The coronal plane was adjusted from the Frankfort horizontal plane (right porion to right orbitale) perpendicular to a line passing through the level of the furcation point of the maxillary right first molar. Lateral cephalographs were generated from the DICOM files of each patient, and the cephalometric analysis was performed according to the guidelines of the American Board of Orthodontics.
Airway volumetric renderings of the subjects’ CBCT scans were developed to measure the volume and minimum axial areas using the airway function of the Dolphin software program. The anatomic landmarks ( Table I ) were identified, and airways were measured in the nasopharygeal, retropalatal, retroglossal, and total airway regions as defined by Arens and Marcus ( Fig ). The posterior superior pharyngeal wall point is defined as a line extending posteriorly from the palatal plane to the posterior pharyngeal wall. The palatal plane is defined as a line connecting the anterior nasal spine to the posterior nasal spine. The posterior middle pharyngeal wall point is defined as a line extending from the posterior inferior tip of the soft palate to the posterior pharyngeal wall and parallel to the palatal plane. The posterior inferior pharyngeal wall point is defined as a line extending posteriorly from the tip of the epiglottis to the posterior pharyngeal wall and parallel to the palatal plane. Airway segmentation threshold values were adjusted to eliminate imaging artifacts and ranged from 50 to 75. The airway volume was then calculated in cubic millimeters, and the most constricted axial area of the airway was calculated in square millimeters.
Anterior boundary | Posterior boundary | Superior boundary | Inferior boundary | |
---|---|---|---|---|
Nasopharynx | Line from sella (S) to posterior nasal spine (PNS) | Line from S to the posterior superior pharyngeal wall | Sella | Line from PNS to the posterior superior pharyngeal wall (SP) |
Retropalatal | Line from PNS to the most posterior inferior point of the soft palate | Line from SP to the posterior middle pharyngeal wall (MP) | Line from PNS to SP | Line from the posterior inferior point of the soft palate to MP |
Retroglossal | Line from the posterior inferior point of the soft palate to the tip of the epiglottis | Line from MP to the posterior inferior pharyngeal wall (IP) | Line from IP of the soft palate to MP | Line from the tip of the epiglottis to IP |
Total airway | Line from S to PNS to the tip of the epiglottis | Line from S to SP to IP | Sella | Line from the tip of the epiglottis to IP |
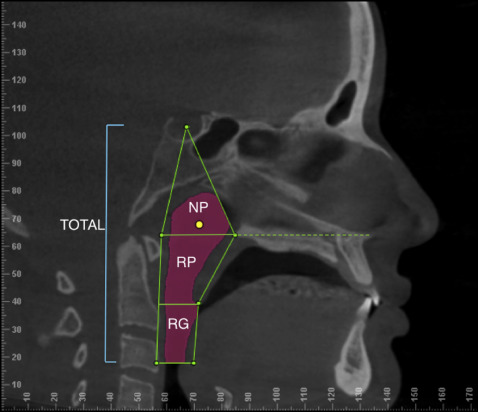
Statistical analysis
The influence of potentially confounding variables such as skeletal classification Class I (ANB angle, 0°-4°) vs Class II (ANB angle, >4°), and sex classification (male vs female) on the baseline (T0) characteristics of the airway were assessed with the unpaired Student t test. Analysis of variance (ANOVA) was used to assess T0 differences between patients with low, normal, and high mandibular plane angles (low angle, ≤27°; normal angle, >27° to <38°; and high angle, ≥38°). The significance of treatment changes (T0 to posttreatment [T1]) of all variables was determined with the paired Student t test. The Wilcoxon signed rank test was used for comparing any nonnormally distributed variable changes. The Bonferroni adjustment was applied to multiple comparisons, and statistical significance was set at P <0.05. Spearman correlation coefficients were calculated to evaluate the relationships between baseline crowding and changes (T0-T1) in volume and minimal cross sectional areas of the airway.
To assess the measurement error of the cephalometric and airway analyses, 40 CBCT scans were randomly selected, and their cephalometric variables and airway dimensions were remeasured 2 weeks after the initial measurements by the same investigator (I.T.T.). Method errors were calculated using Dahlberg’s statistic. The range of errors of the cephalometric analysis was 0.51° to 1.24° for angular measurements. The errors for airway volume were 294.3 mm 3 for total airway, 221.4 mm 3 for the nasopharyngeal airway, 281.3 mm 3 for the retropalatal airway, and 201.4 mm 3 for the retroglossal airway. Measurement errors of the minimal cross-sectional area ranged from 17.6 to 46.7 mm 2 .
Results
The upper airways were studied in 74 healthy adults treated orthodontically with and without dental extractions ( Table II ). No significant differences were found in the mean initial and final airway volumes between mandibular plane angles (normal vs high vs low), anteroposterior skeletal relationships (Class I vs Class II), and the sexes. Consequently, female and male patients, all 3 mandibular plane angle groups, and the Class I and Class II groups were combined as 1 sample to examine the effects of extraction and nonextraction treatments on the airway volumetric changes.
Total n (%) | Nonextraction n (%) | Extraction n (%) | |
---|---|---|---|
Patients | 74 | 48 | 26 |
Male/female ratio | 25/49 | 17/31 | 8/18 |
Age (y) | 30.4 ± 11.4 | 31.9 ± 12.0 | 27.4 ± 9.7 |
Treatment length (mo) | 20.4 ± 5.6 | 18.7 ± 5.4 | 23.5 ± 4.5 |
Class I skeletal relationship | 38 (51.4) | 29 (60.4) | 9 (34.6) |
Class II skeletal relationship | 36 (48.6) | 19 (39.6) | 17 (65.4) |
Low mandibular plane angle (≤27°) | 15 (20.3) | 13 (27.1) | 2 (7.7) |
Normal mandibular plane angle (27°-38°) | 45 (60.8) | 29 (60.4) | 16 (61.5) |
High mandibular plane angle (≥38°) | 14 (18.9) | 6 (12.5) | 8 (30.8) |
The nonextraction group was composed of 17 men and 31 women who were treated orthodontically without the removal of any teeth, excluding third molars. The extraction group consisted of 8 men and 18 women who had at least 2 premolars extracted in conjunction with their orthodontic treatment. At T0, the mean ages were 31.9 ± 12.0 years for the nonextraction group and 27.4 ± 9.7 years for the extraction group. The average treatment times for the nonextraction and extraction groups were 18.7 ± 5.4 months and 23.5 ± 4.5 months, respectively, and this difference was statistically significant ( P <0.05).
The volumetric measurements and minimal cross-sectional areas, as well as treatment changes for the various regions of the airway are described in Table III . The initial and final volumes and the minimal cross-sectional areas were not significantly ( P >0.05) different for the nasopharyngeal, retropalatal, retroglossal, and total airway regions between the extraction and nonextraction groups. The volumes of the total airway were found to decrease by 6.6% (1704.1 mm 3 ) and 6.8% (1366.3 mm 3 ) for the nonextraction and extraction groups, respectively, and the difference was not significant. Similarly, with a large individual variations, the treatment changes for the nasopharyngeal, retropalatal, retroglossal, and total airway regions examined were also not significantly ( P >0.05) different between the 2 groups. The changes in the minimum cross-sectional area also followed this same trend. Reductions in minimal cross-sectional area for the total airway of 13.1% (32.3 mm 2 ) for the nonextraction group, and 16.0% (33.1 mm 2 ) in patients with extractions were observed, and differences between groups were not significant for all airway regions.
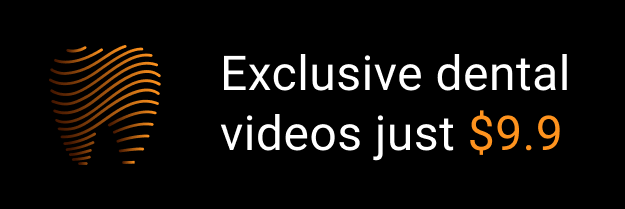