Introduction
Dental models fabricated with 3-dimensional printing technologies are revolutionizing the practice of orthodontics, but they generally comprise polymeric materials that may not be suitable for certain applications, such as soldering appliances. The objective of this study was to investigate the dimensional accuracy and mechanical properties of 3-dimensional printed ceramic-based models before and after various treatments intended to improve their mechanical properties.
Methods
Thirty identical models were printed 3-dimensionally from a calcium sulfate-based substrate and divided into 3 groups for treatment: high heat (250°C for 30 minutes), low heat (150°C for 30 minutes), and Epsom salt treatment. Each model was scanned before and after treatment with a laser scanner, and dimensional stability was analyzed by digital superimpositions using a best-fit algorithm. The models were weighed before and after treatment to evaluate mass changes. Additionally, 3-dimensional printed cylinders treated as described above and an untreated control group were subjected to compressive mechanical testing (n = 11 per group).
Results
The Epsom salt treatment group had statistically significant increases in both peak compressive stress and modulus of elasticity when compared with the other treatment groups. All treatment groups had statistically significant changes in mass, with the Epsom salt group gaining mass and the 2 heat-treatment groups losing mass. The low-temperature treatment group had a statistically significantly lower mean average for dimensional deviations (0.026 ± 0.010 mm) than did the other treatment groups (0.069 ± 0.006 and 0.059 ± 0.010 mm for high temperature and Epsom salt, respectively).
Conclusions
Dental models printed 3-dimensionally with calcium sulfate and treated with Epsom salt showed significant improvement in compressive mechanical properties and retained clinically acceptable dimensional stability.
Graphical abstract
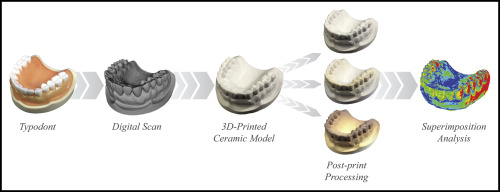
Highlights
- •
Three-dimensionally printed ceramic dental models are accurate and reproducible.
- •
Epsom salt treatment improves model strength and maintains dimensional stability.
- •
Three-dimensionally printed ceramic models may be an alternative to dental stone for some uses.
The digital revolution is changing the way orthodontists practice. Many treatment modalities, such as Invisalign (Align Technology, San Jose, Calif), SureSmile (OraMetrix, Richardson, Tex), and Insignia (Ormco, Orange, Calif) require submission of digital models for treatment planning, thus making it even more important for the orthodontic community to become familiar with the evolving digital model technology. Although digital models have a variety of distinct advantages over traditional plaster models, there are occasions when a physical model is required. Most commonly, physical models are required for fabricating aligners, retainers, and many other appliances. Converting a digital model into a physical model may be accomplished through the process of additive manufacturing, commonly called 3-dimensional (3D) printing. Various 3D printing techniques have been discussed in the literature in the context of orthodontics. These studies have shown select 3D printers to be accurate and to produce models suitable for replacing traditional dental models for diagnosis and treatment planning. However, these studies have only investigated 3D printing techniques that use polymers as the printing medium. Polymer models are well suited for study casts and for the fabrication of most orthodontic appliances but fall short when the appliance requires soldering. Polymers used in 3D printing generally cannot withstand the direct high temperature flame required by soldering, which can reach temperatures in excess of 1650°C.
Alternative 3D printing techniques can print in ceramic-based materials that may have increased heat resistance. One such material is calcium sulfate hemihydrate (CaSO 4 ·½H 2 O). Calcium sulfate hemihydrate may offer a suitable alternative to polymer-based models for the fabrication of soldered appliances; however, the “green” 3D printed models are weak and must undergo postprinting treatments to improve their mechanical properties. Traditionally, parts printed with calcium sulfate hemihydrate are strengthened by infiltration with 2-part epoxy or cyanoacrylate, but these would be inappropriate for soldering applications. Alternative postprinting treatments have been described in the literature that may prove compatible with the high-temperature demands of soldering. These treatments involve altering the crystalline structure of the calcium sulfate hemihydrate through heat treatment or by immersion in a magnesium sulfate solution. Therefore, the aim of this study was to investigate the dimensional accuracy and mechanical properties of 3D printed dental models made from calcium sulfate hemihydrate before and after various postprinting treatments.
Material and methods
Fabricating 3D printed models
A master stereolithography (STL) file of a maxillary arch form was created based on an ideal dental typodont (CON2001-UL-UP-FEM-32; Nissin, Kyoto, Japan). First, a polyvinyl siloxane (Aquasil Ultra XLV; Dentsply, York, Pa) impression was taken of the typodont, and traditional dental stone (0-67 Snow White; Heraeus Kulzer, South Bend, Ind) was used to cast a stone model. Next, the stone model was scanned using an Ortho Insight 3D laser scanner (Motion View Software, Chattanooga, Tenn) to produce the master STL file. This file was then used to 3-dimensionally print 30 identical ceramic-based models using a ProJet 660 Pro 3D printer (3D Systems, Rock Hill, SC). The printing material consisted of calcium sulfate hemihydrate powder (VisiJet PXL Core) and liquid inkjet binder (VisiJet PXL Clear) both from 3D Systems. Each 3D printed model was individually scanned before treatment with the 3D laser scanner, resulting in 30 pretreatment STL files.
Experimental treatment
Three treatment protocols were performed on the 3D printed models. The first group (n = 10) was heat treated to 250°C in a bench-top muffle furnace (Vulcan A-550; Dentsply/Ceramco, York, Pa). The models were heated at a rate of 20°C per minute until the target temperature was reached and then held for 30 minutes. After 30 minutes, the furnace was switched off and allowed to cool to room temperature. The second group (n = 10) was also heat treated using the same process but to a temperature of 150°C. The final group (n = 10) was treated in a solution of Epsom salt (magnesium sulfate USP 100%; CVS, Woonsocket, RI) in accordance with a protocol from 3D Systems. Briefly, a solution (1 part water by volume to 0.7 part by volume salt) was prepared using tap water and Epsom salt. The solute was allowed to fully dissolve. The models were then fully immersed for 30 seconds. They were then slowly removed, and excess water was allowed to drip off. The models were then placed on paper towels to air dry for 1 hour. The models were then dried in a metal box heated with a 75-W incandescent light bulb for 24 hours. After treatment, all 3 groups were rescanned using the 3D laser scanner, resulting in 30 posttreatment STL files.
Dimensional stability analysis
Overall dimensional changes were analyzed using Rapidform 2006 software (Inus Technology, Seoul, Korea). Two comparisons were made for each of the 30 printed models: master STL vs pretreatment STL, and pretreatment STL vs posttreatment STL.
First, the 2 STL files being compared were imported into the software, and nonanatomic portions of the models (eg, the base) were cropped out, resulting in 2 shells containing structures representing the teeth, gingiva, palatal vault, and oral tissues adjacent to the mucogingival junction ( Fig 1 ). Next, the files were superimposed, one on top of the other, using a best-fit algorithm in the software. Finally, the software reported the average deviation between the shells (in millimeters) and the standard deviation. The software reports the average deviation as an absolute value.
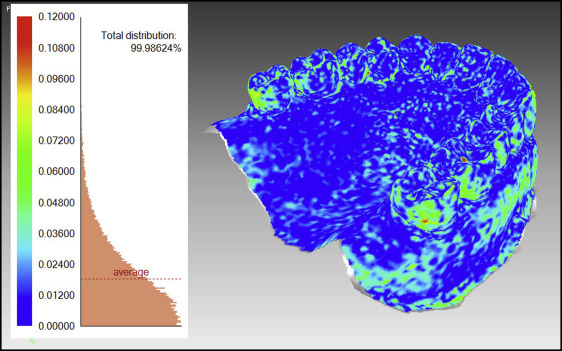
Material properties
Forty-four 3D printed test cylinders (6 mm diameter × 12 mm height) were mechanically tested in accordance with ASTM C1424 . The z-print axis was oriented parallel to the long axis of the cylinder. The cylinders were divided into 3 treatment groups and an untreated control group: (1) high-temperature treatment (250°C, 30 minutes), (2) low-temperature treatment (150°C, 30 minutes), (3) Epsom salt treatment, and (4) green state (control, no treatment). Compressive mechanical testing was performed using a universal testing machine with a 5-kN static load cell (model 4465; Instron, Norwood, Mass) at a displacement rate of 0.5 mm per minute. Peak compressive stress (megapascals) and compressive modulus of elasticity (megapascals) were recorded.
Mass analysis
Each 3D printed model was weighed before and after experimental treatment. Measurements were made using a laboratory digital scale (PM 4600; Mettler Toledo, Greifensee, Switzerland) and recorded to the nearest 0.01 g. The difference between the pretreatment and posttreatment masses for each model was then calculated.
Statistical analysis
Average deviations of the superimpositions, peak compressive stresses, compressive modulus of elasticity values, and changes in mass were evaluated using 1-way analysis of variance (ANOVA) with a level of significance set at P <0.05. Post hoc pairwise comparisons with the Tukey test were then applied. All calculations were performed using SPSS Statistics for Windows (version 23; IBM, Armonk, NY).
Results
Mechanical testing
Both mean peak compressive stress and compressive modulus of elasticity showed statistically significant differences between the treatment groups via 1-way ANOVA ( P <0.001) ( Table I ). Post hoc pairwise comparisons with the Tukey test indicated that the Epsom salt treatment group was significantly higher than all other groups for both variables ( Table II ). In addition, the high-temperature group had a significantly lower peak compressive stress than did the low-temperature group ( P <0.05).
Green | High temperature | Low temperature | Epsom salt | P | |
---|---|---|---|---|---|
Stress (MPa) | 4.06 ± 0.56 | 3.82 ± 0.76 | 4.85 ± 0.47 | 7.13 ± 1.04 | 0.000 ∗ |
Modulus (MPa) | 209.09 ± 45.13 | 209.82 ± 41.08 | 233.23 ± 36.25 | 478.00 ± 140.81 | 0.000 ∗ |
Epsom salt | Low temp | High temp | Green | |
---|---|---|---|---|
Peak compressive stress | ||||
Epsom salt | – | 0.000 ∗ | 0.000 ∗ | 0.000 ∗ |
Low temp | 0.000 ∗ | – | 0.012 ∗ | 0.078 |
High temp | 0.000 ∗ | 0.012 ∗ | – | 0.868 |
Green | 0.000 ∗ | 0.078 | 0.868 | – |
Modulus of elasticity | ||||
Epsom salt | 0.000 ∗ | 0.000 ∗ | 0.000 ∗ | |
Low temp | 0.000 ∗ | – | 0.898 | 0.889 |
High temp | 0.000 ∗ | 0.898 | – | 1.000 |
Green | 0.000 ∗ | 0.889 | 1.000 | – |
Mass analysis
One-way ANOVA ( P <0.001) indicated a statistically significant difference in the mass change upon treatment between the groups. Post hoc pairwise comparisons with the Tukey test showed that mass changes for all treatment groups were significant with respect to each other ( P <0.001) ( Table III ). Specifically, the Epsom salt treatment group on average gained 6.85 ± 1.77 g, whereas the high-temperature and low-temperature groups on average lost 7.79 ± 0.32 and 3.55 ± 0.41 g, respectively ( Table III ).
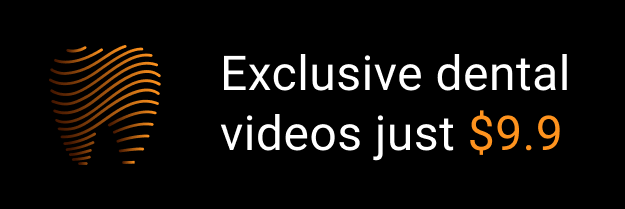