Abstract
Objectives
The aim of this study was to evaluate the effectiveness of liner and base materials to reduce the stress resulting from polymerization shrinkage. The null hypothesis tested was that the presence of low-viscosity liner and base materials under the composite resin restoration reduces the polymerization shrinkage stress.
Methods
A quasi-three-dimensional photoelastic model of a second premolar with a class I preparation was restored using four experimental groups ( n = 7): RC, resin composite (Filtek Z250); FLRC, flowable liner (Filtek Flow) + resin composite restoration; VLRC, resin-modified glass-ionomer liner + resin composite restoration; and VBRC, resin-modified glass-ionomer base + resin composite restoration. The maximum shear stresses ( τ max ) were calculated along the adhesive interface in 13 predefined and standardized point locations. Data were submitted to one-way ANOVA, followed by a Tukey’s post hoc test ( p < 0.05).
Results
A significant difference was found among the experimental groups ( p = 0.001); therefore, the null hypothesis was rejected. The mean maximum shear stress was: 38.0 kPa for RC, 52.1 kPa for FLRC, 72.8 kPa for VLRC, and 90.2 kPa for VBRC. The polymerization shrinkage stress level from least to greatest was: RC < FLRC < VLRC < VBRC. The overall stress distribution in class I restoration indicated that stresses were primarily accumulated at the cavosurface and internal line angles.
Significance
Using a flowable composite or resin-modified glass-ionomer as liner or base material under composite resin restoration increases the polymerization shrinkage stresses at the adhesive interface leading to a possible adhesive failure.
1
Introduction
The use of composite resin for posterior restorations has increased considerably in recent years . The ability to achieve excellent esthetic results, improvements in composite mechanical/physical properties, as well as the development of new adhesive systems that allow for more conservative restorative techniques contributed to the enhanced performance of composite resins .
However, shrinkage during polymerization of resin-based materials remains a concern. Such shrinkage is considered the major cause of gap formation at the tooth/restoration interface. Shrinkage can initiate tooth structure microcracking, fracture of the restoration, secondary caries, post-operative sensitivity, and cusp deflexion . Conversely, few data is available regarding the polymerization shrinkage of resin-modified glass-ionomers (RMGIs) .
Restorative techniques that reduce the level of stress caused by resin composite polymerization shrinkage have been suggested . To create a stress-absorbing layer, the placement of a cavity liner or base of low-viscosity/low-elastic modulus materials such as RMGIs, filled adhesives, and flowable composites has been suggested. This layer increases the strain capacity and reduces the stresses at the adhesive interface . In an attempt to provide volumetric reduction of resin composite, RMGIs were recommended as cavity base. However, the polymerization shrinkage for RMGIs found in recent studies contradicts the notion that RMGIs have less polymerization shrinkage than that of composite resin. Thus, the benefit of these techniques to reduce polymerization shrinkage and stress-relieving remains controversial .
The mechanisms of stress in the adhesive interface of a bonded restoration are complex. Reduction in microleakage , and measurements of microtensile bond strengths confirm the benefits of low-viscosity/low-elastic modulus materials. However, none of these methodologies is a direct measurement of polymerization shrinkage stress. In order to have a proper understanding of the factors related to polymerization stress, specific methodology for stress analysis is required. Use of methodologies such as finite element analysis , extensometry , photoelasticity , and tensilometry have been responsible for important advances in polymerization stress analysis.
The aim of this study was to evaluate the efficacy of low-viscosity/low-elastic modulus liner and base materials placed under composite resin restoration. The research hypothesis was that the use of liner and base low-viscosity materials under composite resin restoration reduces the polymerization shrinkage stress.
2
Materials and methods
2.1
Photoelastic model
An extracted caries-free human maxillary second premolar was selected for the present study after examination with a stereomicroscope (Carl Zeiss Microimaging, Inc., Thornwood, NY) at 20× magnification to detect possible enamel cracks or fissures, and stored in 0.5% chloramine (Fisher Scientific, Pittsburgh, PA) at 4 °C. The tooth was then cross-sectioned through its long axis with a precision, water-cooled, slow-speed diamond saw (IsoMet 1000; Buehler Ltd., Lake Bluff, IL). The resultant facial-lingual cross-sections were cleaned and polished with silicon carbide papers of decreasing abrasiveness (600-, and 800-grit). The two hemi-sections were then evaluated with a stereomicroscope (Carl Zeiss Microimaging) at 20× magnification and the hemi-section exhibiting the best structural integrity was selected. The selected cross-section was scanned (Scanjet 3200C HP, Hewlett Packard de Mexico S.A., Guadalajara, Jalisco, Mexico) and the digital image was transferred to a computer aid designer (CAD) software (Microstation, MicroStation Bentley Systems Inc., St. Louis, USA). A class I preparation was digitally designed and created on the computer screen exhibiting the following features: faciolingual walls smooth and slightly converging pulpo-occlusally, pulpal floor flat, smooth, and perpendicular to the tooth long axis, rounded internal line angles, occlusal cavosurface margins well-defined (cavosurface angle of 90°). The digitalized preparation had the followed dimensions: 2.04 mm faciolingual isthmus width, 2.79 mm pulpal floor length, and 3.89 mm pulpo-occlusal extension.
The computerized prepared tooth image was calculated in a 3:1 scale and transferred to an acrylic plate (30.0 mm 2 and 5.0 mm thickness). Then a prepared tooth acrylic template was generated in order to build a photoelastic model. Since volume and polymerization shrinkage stress have a linear relationship , the acrylic template consisted of three times magnification of all the preparation dimensions to facilitate the reading of the photoelastic pattern (6.0 mm faciolingual isthmus width, 8.4 mm pulpal floor length, and 11.7 mm pulpo-occlusal extension). This particular photoelastic model is considered a quasi-three-dimensional model .
Twenty-eight impressions of the acrylic template were made using a vinyl polysiloxane impression material (ASB 10 Azul, Polipox Industria e Comercio Ltda, São Paulo, SP, Brazil). A special designed epoxy resin (Adesivo Brinde Flexivel, Polipox Industria e Comercio Ltda) with photoelastic properties was selected (photoelastic constant: K σ = 0.62 N/mm). The epoxy resin was mixed according to the manufacturer’s instructions and poured into the silicone mold. The sets were stored at 35 °C for 72 h to allow for complete setting.
2.2
Restorative procedures
The photoelastic models were retrieved from the silicone mold and assigned to four experimental groups ( n = 7) according to the following restorative techniques: RC, resin composite; FLRC, flowable liner + resin composite restoration; VLRC, resin-modified glass-ionomer liner + resin composite restoration; and VBRC, resin-modified glass-ionomer + resin composite restoration ( Table 1 ). All materials were used according to the manufacturer’s instructions.
Material | Composition | Manufacturer |
---|---|---|
Adper Scothbond Multi Purpose Plus Adhesive | Bis-GMA and HEMA, photoinitiators, stabilizers (lot: 1MH) | 3M ESPE, St. Paul, MN, EUA |
Filtek Z250 | Bis-GMA, bis-EMA(6), UDMA, photoinitiators, zirconia/silica filler, 82% filler by weight (lot: 3FG) | |
Filtek Flow | Bis-GMA, TEGDMA, dimethacrylate polymer, zirconia/silica filler, photoinitiators, 68% filler by weight (lot: 3FK) | |
Vitrebond | Powder: fluoroaluminosilicate glass (lot: 3FE) Liquid: acrylic acid copolymer containing pendant, methacryloxy groups, HEMA, water, photoinitiators (lot: 3EB) |
For all photoelastic replicas, before the restorative procedures, the preparations were acid-etched with 35% phosphoric acid for 60 s (ScotchBond Etchant; 3M ESPE, St. Paul, MN, USA) to remove any contaminant that could interfere with the bonding procedures. The preparations were then rinsed with distilled water for 10 s and blot-dried using an absorbent paper.
2.2.1
RC: resin composite
Because the photoelastic material is sensitive to solvents, the primer was not applied to avoid atypical fringe formation. A phosphate-based adhesive (Adper Scotchbond Multi Purpose Adhesive, 3M ESPE) was selected and applied over the entire surface of the preparation, carefully air-thinned, and light-polymerized for 60 s (XL2500, 3M ESPE). The preparation was restored with six horizontal increments of 2.0 mm each using a microhybrid composite resin (Filtek Z250, 3M ESPE). Each increment was light-polymerized for 60 s (XL2500, 3M ESPE) at a 0.5 mm curing distance and light intensity of 600 mW/cm 2 constantly monitored with a radiometer, before its succeeding layer was applied.
2.2.2
FLRC: flowable composite liner + resin composite
The adhesive (Adper Scotchbond Multi Purpose Adhesive, 3M ESPE) was applied to another photoelastic replica as described above. The first layer was a 2.0 mm thick layer of flowable composite (Filtek Flow, 3M ESPE) and light-polymerized for 60 s. The resin composite (Filtek Z250, 3M ESPE) was then applied in 2.0 mm thick horizontal increments until the preparation was completed (5 increments). Each resin composite increment was light-polymerized for 60 s (XL2500, 3M ESPE) before its successor.
2.2.3
VLRC: resin-modified glass-ionomer (RMGI) liner + resin composite
A 2.0 mm thick liner of RMGI (Vitrebond, 3M ESPE, St. Paul) was applied to a fresh replica and light-polymerized for 60 s. The adhesive (Adper Scotchbond Multi Purpose Adhesive, 3M ESPE) was applied to the preparation and RMGI liner, carefully air-thinned, and light-polymerized for 60 s (XL2500, 3M ESPE). The preparation was then restored with microhybrid composite (Filtek Z250, 3M ESPE) in 2.0 mm increments, each increment being light-polymerized for 60 s (XL2500, 3M ESPE). Five resin composite increments were added to restore the preparation.
2.2.4
VBRC: resin-modified glass-ionomer base + resin composite
A 4.0 mm thick cavity base was produced for this experimental group. The RMGI base consisted of two increments: a 2.0 mm thick layer of RMGI (Vitrebond, 3M ESPE) applied on the pulpal floor and light-polymerized for 60 s (XL2500, 3M ESPE), then a second 2.0 mm thick layer was applied and light-polymerized for 60 s (XL2500, 3M ESPE). The adhesive (Adper Scotchbond Multi Purpose Adhesive, 3M ESPE) was applied to the preparation and RMGI base, carefully air-thinned, and light-polymerized for 60 s (XL2500, 3M ESPE). Four horizontal increments of resin composite (Filtek Z250, 3M ESPE) were used to restore the preparation. Each increment was placed and light-polymerized for 60 s (XL2500, 3M ESPE).
2.3
Photoelastic measurement
The restored photoelastic models were analyzed using a transmission polariscope (Profile Projector Mitutoyo, Mitutoyo Corporation, Kawasaki, Japan). Images of the restored photoelastic models were recorded at the baseline (immediately), 15 min, 30 min, 60 min, 8 h, and 48 h after final polymerization. The stress along the adhesive interface was evaluated for all experimental groups. The isochromatic fringe patterns of the stressed sites were determined by their respective fringe orders. The photoelastic color patterns were used to compare the distribution of polymerization shrinkage stress within the different experimental groups ( Fig. 1 a) . A pilot study showed no changes on the maximum shear stress of the studied materials after 48 h. Therefore, the quantitative analysis of stress was recorded 48 h after light-polymerization and the values of maximum shear stress ( τ max ) calculated in kPa. Thirteen point locations along the adhesive interface were selected and standardized for all specimens ( Fig. 1 b). Isochromatic fringe order values were calculated from the 13 standardized selected points determined by the Tardy compensation method . Then, the relationship between principal stress ( σ 1 and σ 2 ) and maximum shear stress ( τ max ) was defined by the Stress Optic Law using the following equation:
σ 1 − σ 2 = N t ⋅ K σ = 2 ⋅ τ max
where σ 1 and σ 2 are the principal stress, τ max is the maximum shear stress, N is the fringe order (isochromatic), K σ is the photoelastic constant/optical coefficient, and t is the thickness of the model. The optical coefficient of the photoelastic material was defined utilizing the calibration technique of Dally and Riley and set at 0.62 N/mm. The photoelastic model was standardized for all experimental groups in 5.0 mm thickness. By applying the values of the photoelastic constant, thickness, and fringe order, the τ max was calculated using the above equation. Maximum shear stress data of the 13 selected points along the adhesive interface were submitted to repeated measurements one-way ANOVA (analysis of variance), followed by Tukey’s post hoc test at p = 0.05.

2
Materials and methods
2.1
Photoelastic model
An extracted caries-free human maxillary second premolar was selected for the present study after examination with a stereomicroscope (Carl Zeiss Microimaging, Inc., Thornwood, NY) at 20× magnification to detect possible enamel cracks or fissures, and stored in 0.5% chloramine (Fisher Scientific, Pittsburgh, PA) at 4 °C. The tooth was then cross-sectioned through its long axis with a precision, water-cooled, slow-speed diamond saw (IsoMet 1000; Buehler Ltd., Lake Bluff, IL). The resultant facial-lingual cross-sections were cleaned and polished with silicon carbide papers of decreasing abrasiveness (600-, and 800-grit). The two hemi-sections were then evaluated with a stereomicroscope (Carl Zeiss Microimaging) at 20× magnification and the hemi-section exhibiting the best structural integrity was selected. The selected cross-section was scanned (Scanjet 3200C HP, Hewlett Packard de Mexico S.A., Guadalajara, Jalisco, Mexico) and the digital image was transferred to a computer aid designer (CAD) software (Microstation, MicroStation Bentley Systems Inc., St. Louis, USA). A class I preparation was digitally designed and created on the computer screen exhibiting the following features: faciolingual walls smooth and slightly converging pulpo-occlusally, pulpal floor flat, smooth, and perpendicular to the tooth long axis, rounded internal line angles, occlusal cavosurface margins well-defined (cavosurface angle of 90°). The digitalized preparation had the followed dimensions: 2.04 mm faciolingual isthmus width, 2.79 mm pulpal floor length, and 3.89 mm pulpo-occlusal extension.
The computerized prepared tooth image was calculated in a 3:1 scale and transferred to an acrylic plate (30.0 mm 2 and 5.0 mm thickness). Then a prepared tooth acrylic template was generated in order to build a photoelastic model. Since volume and polymerization shrinkage stress have a linear relationship , the acrylic template consisted of three times magnification of all the preparation dimensions to facilitate the reading of the photoelastic pattern (6.0 mm faciolingual isthmus width, 8.4 mm pulpal floor length, and 11.7 mm pulpo-occlusal extension). This particular photoelastic model is considered a quasi-three-dimensional model .
Twenty-eight impressions of the acrylic template were made using a vinyl polysiloxane impression material (ASB 10 Azul, Polipox Industria e Comercio Ltda, São Paulo, SP, Brazil). A special designed epoxy resin (Adesivo Brinde Flexivel, Polipox Industria e Comercio Ltda) with photoelastic properties was selected (photoelastic constant: K σ = 0.62 N/mm). The epoxy resin was mixed according to the manufacturer’s instructions and poured into the silicone mold. The sets were stored at 35 °C for 72 h to allow for complete setting.
2.2
Restorative procedures
The photoelastic models were retrieved from the silicone mold and assigned to four experimental groups ( n = 7) according to the following restorative techniques: RC, resin composite; FLRC, flowable liner + resin composite restoration; VLRC, resin-modified glass-ionomer liner + resin composite restoration; and VBRC, resin-modified glass-ionomer + resin composite restoration ( Table 1 ). All materials were used according to the manufacturer’s instructions.
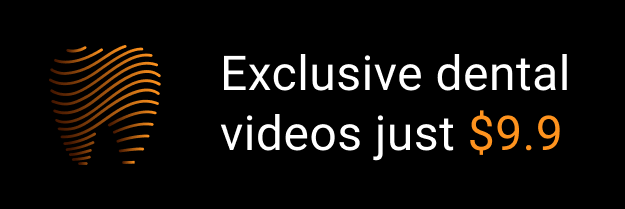