Introduction
Impression-free techniques might eliminate the potential shortcomings of digital dental models. Chairside scanners offer the advantage of obtaining digital dental models directly from the patient without the need for dental impressions. The aim of this study was to evaluate the accuracy of 3-dimensional digital models acquired from a chairside intraoral scanner compared with both manual and cone-beam computed tomography measurements of the same dental anatomy.
Methods
The study sample comprised 60 dry skulls. Each skull had the maxillary and mandibular arches scanned with a Cadent iTero scanner (Align Technology, San Jose, Calif) and had a cone-beam computed tomography scan taken with a CS 9300 unit (Carestream Health, Atlanta, Ga). Linear measurements in all 3 dimensions of the space in each dental arch together with tooth-size arch-length analysis for both the maxillary and mandibular arches were carried out manually on the dry skulls with calipers and digitally on the scanned 3-dimensional models and cone-beam computed tomography images. Intraclass correlation (ICC) analysis was performed for all variables tested in the study groups, with the manual measurements on the dry skulls as the gold standard. The Bland-Altman analysis was also applied to the data to graphically display the agreement of the diagnostic measurements obtained from these methods.
Results
Measurements from the iTero models demonstrated near-perfect agreement (ICC, 0.91-0.99) with the caliper measurements. Cone-beam computed tomography measurements had moderate to high levels of agreement (ICC, 0.65-0.99) compared with the caliper measurements.
Conclusions
Direct digital acquisition of the dental arches with a chairside scanner provided almost 1-to-1 diagnostic information of the investigated anatomy and was superior to the cone-beam computed tomography measurements.
Through advances in biomaterials, robust and dimensionally accurate dental plaster models are possible and have long been the gold standard in orthodontics. In the new digital era, various technological advancements have made their way into dental practices. The past decade has seen the advent of digital models with acceptable quality, allowing the orthodontic record to become completely digitized.
For orthodontics, the most important expectation from a digital model system lies in its diagnostic accuracy and reliability. Although the consensus is that measurements with digital models compare well with those derived from plaster models, several studies that investigated complex measurements such as space available, irregularity index, and Bolton analysis indicated that mean differences between the plaster and digital models can exceed 1.5 mm. This much difference might not be clinically acceptable. However, there is also contrary evidence in the literature that supports the validity of digital models for the aforementioned measurements.
Many professionals obtain their digital models through the use of proprietary services. Traditional impressions and plaster models must be submitted to the selected company so that they can be scanned and the digital models can be generated and made available for downloading. Submission of models or impressions by mail can result in breakage of the models or distortion of the impressions during shipment. Even if no breakage or distortion occurs during shipment, the impression materials have an inherent amount of distortion at their time of use. Moreover, there are technique-specific differences in the production phase of digital models such as surface scanning algorithms. All of these might account for the statistical variability related to the accuracy of digital dental models.
Perhaps elimination of the conventional impression step and generation of dental models directly from the 3-dimensional (3D) dental anatomy will yield more accuracy. One way of doing this is to use the DICOM files produced by cone-beam computed tomography (CBCT) scanners. An advantage of this technology is that it allows for the visualization of supragingival structures as well as impacted teeth, bone levels, and joints. Studies using various scanners have been published showing that diagnostically accurate measurements can be made from CBCT scans. Perhaps the greatest drawback of using these scanners to acquire digital models is the required radiation exposure to the patient and the equipment expense to the practitioner. Some authors have also expressed concern over the absence of gingival tissues in the resultant files and the inability to accurately depict buccal alveolar bone.
For those wishing to gain an accurate depiction of gingival tissues and avoid exposing the patient to radiation, an intraoral scanner might be more appropriate. Intraoral scanners, now widely available from several manufacturers, are self-contained in rolling units, making them portable around the office. In this study, we evaluated the diagnostic accuracy of an intraoral scanner and CBCT as sources of digital models from natural dentitions for orthodontic diagnosis compared with direct caliper measurements of the investigated dental anatomy.
Material and methods
The study sample comprised 60 dry skulls with intact dentition chosen from a unique collection at the University of Texas Health Science Center in Houston.
The Carestream CS 9300 (Carestream Health, Atlanta, Ga) was used to capture the CBCT images ( Fig 1 ) of the skulls with exposure parameters of 90 kV, 4 mA, and 300-μm voxel size and volume dimensions of 17 × 13.5 cm. The image reconstruction time was approximately 5 minutes. Each arch was then scanned using the reference model setting on the Cadent iTero scanner running software version 4.0.5.31 (Align Technology, San Jose, Calif). The raw images were available for chairside viewing within 2 minutes after completion of the scanning. These images were then sent via the Internet to Align Technology, where they were reprocessed and made available for downloading within 48 hours as a stereolithography file ( Fig 2 ). This file format is an industry standard native to stereolithography computer-aided design and can be easily viewed and manipulated in various software applications without loss of quality.
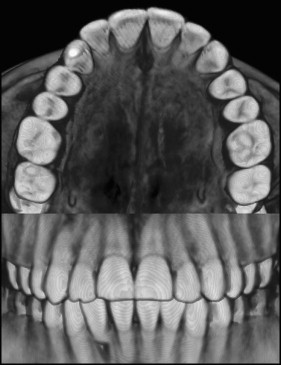
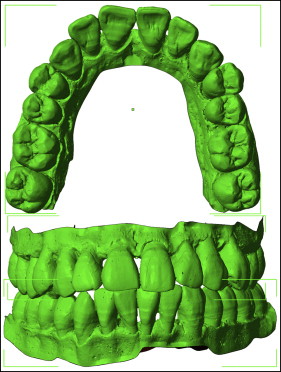
With digital calipers, a tooth-size arch-length analysis was performed in each arch. In addition to crowding measurements, 3 teeth in each arch were measured in the anteroposterior, transverse, and vertical dimensions ( Fig 3 ). For the transverse measurement, each tooth was measured at the center of its long axis at its most convex points on the buccal and lingual surfaces ( Fig 3 , A ). The anteroposterior measurement was defined as the most mesiobuccal contact point to the most distobuccal contact point ( Fig 3 , B ). In the vertical plane, each tooth was measured on the buccal surface at the middle of its long axis from the height of the crestal bone to the cusp tip ( Fig 3 , C ). In the maxillary arch, measurements were made on a molar, a premolar, and a canine. In the mandibular arch, measurements were made on a molar, a premolar, and an incisor ( Table I ).
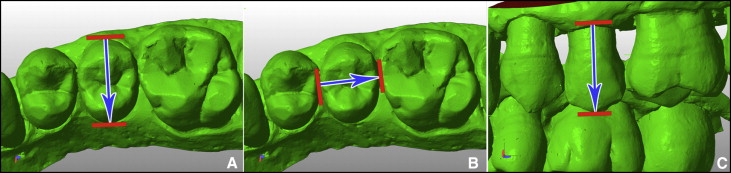
Label | Description |
---|---|
UM MD | Mesial distal width of upper permanent molar |
UM V | Vertical height of upper permanent molar |
UM BL | Buccolingual width of upper permanent molar |
UP MD | Mesiodistal width of upper permanent premolar |
UP V | Vertical height of upper permanent premolar |
UP BL | Buccolingual width of upper permanent premolar |
UC MD | Mesiodistal width of upper canine |
UC V | Vertical height of upper canine |
UC BL | Buccolingual width of upper canine |
LI MD | Mesiodistal width of lower permanent incisor |
LI V | Vertical height of lower permanent incisor |
LI BL | Buccolingual width of lower permanent incisor |
LP MD | Mesiodistal width of lower permanent premolar |
LP V | Vertical height of lower permanent premolar |
LP BL | Buccolingual width of lower permanent premolar |
LM MD | Mesiodistal width of lower permanent molar |
LM V | Vertical height of lower permanent molar |
LM BL | Buccolingual width of lower permanent molar |
The CBCT files were opened using the InVivo Dental software (version 5.1.9; Anatomage, San Jose, Calif), and measurements were made in millimeters on sectioned views of a gray-scale 3D reconstruction using the software’s built-in tool for measuring the distance between 2 defined points. Scanner stereolithography files were opened in Studio Basic (version 0.1; nettfabb, Lupburg, Germany). The measurements were made on unsectioned solid shaded models using the application’s built-in ruler tool.
Statistical analysis
Intraclass correlation analysis (ICC) was performed for all variables tested in the study groups, with the manual measurements from the dry skulls serving as the gold standard. ICC values were computed using the 2-way random effect model (absolute agreement definition). SPSS statistical software (version 21; IBM, Armonk, NY) was used to analyze the data. Additionally, the Bland-Altman analysis was performed with XLSTAT Mac (version 2012; Addinsoft, New York, NY) to visually demonstrate the agreement of the maxillary and mandibular crowding values between the manual caliper measurements and the 2 methods tested. The within-observer repeatability was evaluated with ICC values by repeating all measurements from 10 randomly selected skulls 1 month later.
Results
Descriptive statistics for the measurements are presented in Table II . Intraobserver repeatability was excellent with ICC values between 0.97 and 0.99. Table III displays the ICC values for linear and crowding measurements for both CBCT and the iTero models compared with the manual caliper measurements. The ICC values for the linear measurements in the comparison of caliper measurements with CBCT measurements ranged between 0.88 and 0.99, displaying near-perfect agreement, except for the buccolingual measurement of the mandibular incisor (ICC, 0.65). The ICC values of the comparison of caliper measurements with the measurements made on the iTero models ranged between 0.92 and 0.99, showing a much higher level of agreement. However, both methods had ICC values greater than 0.98 for maxillary and mandibular crowding compared with manual measurements, indicating strong evidence of their diagnostic capability.
Variable (mm) | Dry skulls | CBCT | iTero | |||
---|---|---|---|---|---|---|
Mean | SD | Mean | SD | Mean | SD | |
UM MD | 9.86 | 0.64 | 9.84 | 0.69 | 9.83 | 0.67 |
UM V | 7.86 | 1.49 | 7.73 | 1.53 | 7.81 | 1.47 |
UM BL | 10.67 | 0.66 | 10.62 | 0.74 | 10.69 | 0.63 |
UP MD | 7.02 | 0.89 | 7.07 | 0.95 | 6.94 | 0.91 |
UP V | 9.25 | 1.85 | 9.22 | 1.9 | 9.23 | 1.86 |
UP BL | 9.17 | 0.56 | 9.23 | 0.56 | 9.19 | 0.62 |
UC MD | 7.26 | 0.69 | 7.34 | 0.71 | 7.2 | 0.63 |
UP V | 12.21 | 2.21 | 12.25 | 2.29 | 12.1 | 2.21 |
UC BL | 7.68 | 0.89 | 7.66 | 0.89 | 7.61 | 0.9 |
LI MD | 5.76 | 1.42 | 5.6 | 1.48 | 5.51 | 0.41 |
LI V | 11.46 | 1.82 | 11.56 | 2.03 | 11.32 | 1.63 |
LI BL | 5.86 | 0.41 | 5.98 | 0.56 | 5.86 | 0.44 |
LP MD | 7.21 | 1.28 | 7.28 | 1.27 | 7.16 | 1.29 |
LP V | 8.56 | 1.6 | 8.49 | 1.54 | 8.55 | 1.57 |
LP BL | 7.96 | 0.71 | 8.04 | 0.64 | 7.98 | 0.68 |
LM MD | 10.66 | 0.7 | 10.75 | 0.66 | 10.61 | 0.72 |
LM V | 7.6 | 0.98 | 7.29 | 1.04 | 7.56 | 1.01 |
LM BL | 10.03 | 0.62 | 9.98 | 0.59 | 10.01 | 0.61 |
Mandibular crowding | 4.18 | 6.28 | 4.36 | 6.25 | 4.25 | 6.28 |
Maxillary crowding | 2.22 | 6.36 | 2.56 | 6.9 | 2.47 | 6.38 |
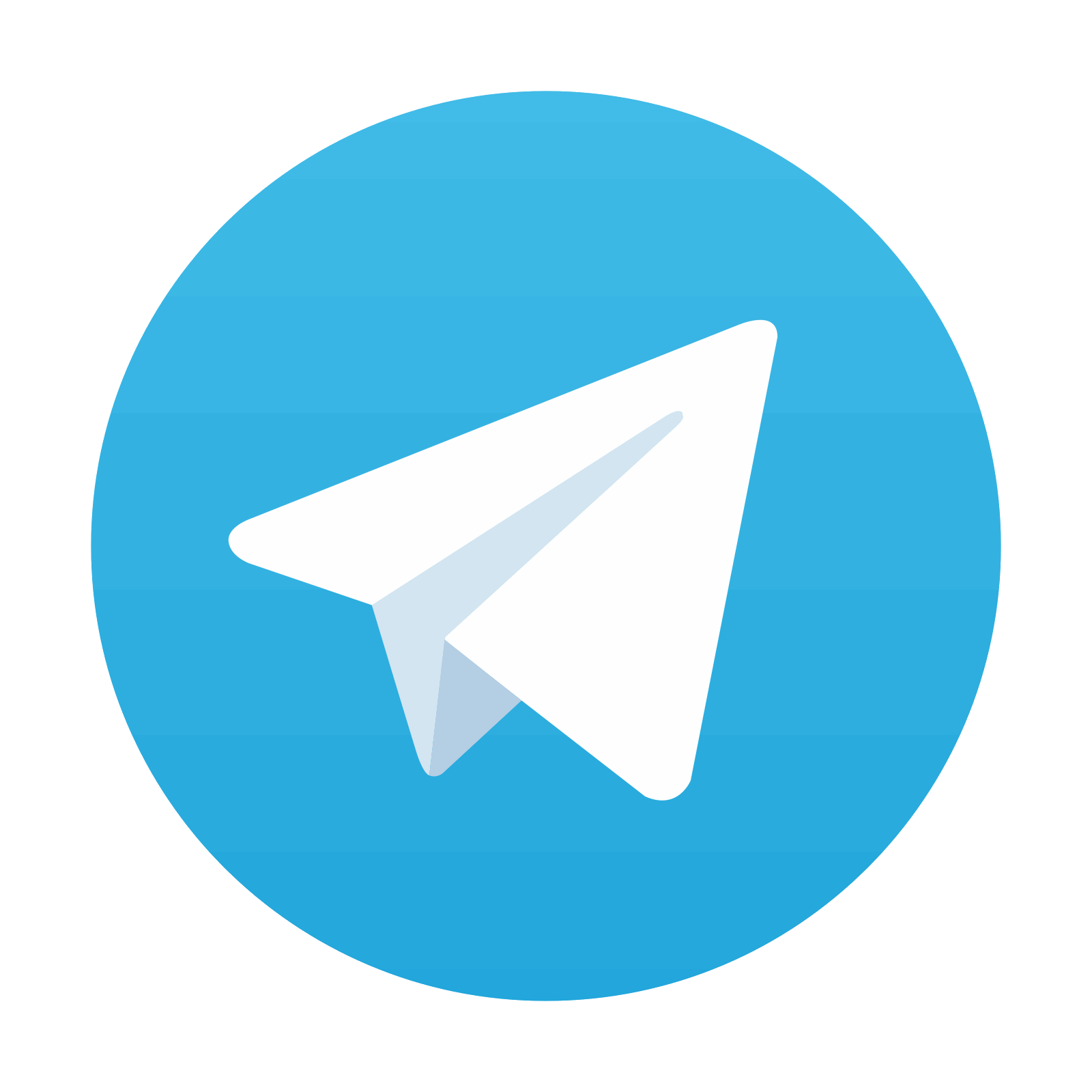
Stay updated, free dental videos. Join our Telegram channel

VIDEdental - Online dental courses
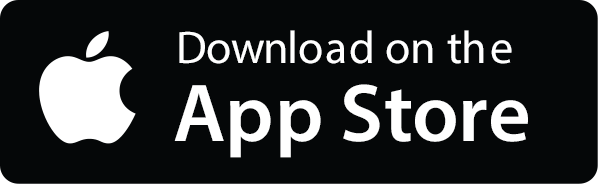
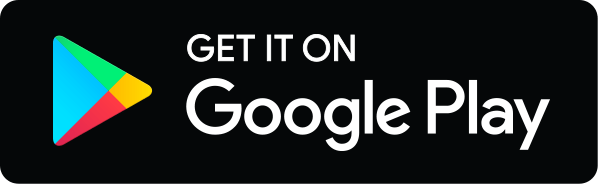
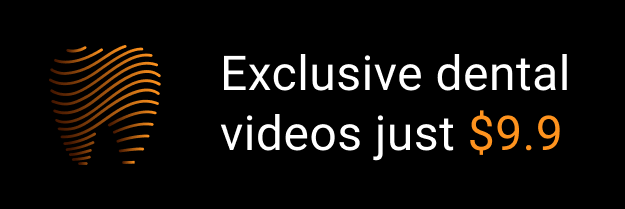