Development of the Dentition and Dental Occlusion
In any difficult situation, the first step toward remediation is to recognize the problem. The first step in recognizing the problem is to know how the problem has developed. Thus, the best “cure” is early recognition and proper intervention before the problem arises. A review of the prenatal stages of tooth development, especially those occurring during the embryonic period, helps to clarify the normal relationships of adult body structures and the causes of congenital anomalies.
It is not within the scope of this chapter to discuss all aspects of embryology and odontogenesis. There are many reference texts on oral histology, embryology, and developmental anatomy. There are also many contemporary research reports about the molecular level of tooth development. However, this chapter will provide a brief review of the different stages of dentition during prenatal life.
The embryonic period is one of the most critical developmental events, including weeks 3 to 8 of intrauterine life. During this stage, all major external and internal structures are established. Most developmental processes are controlled by precise coordination and interaction of genetic and environmental factors. This control mechanism guides differentiation and synchronizes tissue interactions, migration of cells, and controlled proliferation.
Most facial structure is ultimately derived from migration of neural crest cells, which occurs during the embryonic period. Any interference with this migration can cause different craniofacial anomalies. For example, teratogenic agents, such as viruses and some drugs, can produce this interference and trigger the incidence of congenital anomalies.
Development of the dentition is also a sequence of events that occurs in an orderly and timely fashion; it begins during this stage (week 6 of intrauterine life), continues as a very long process, and ends after the age of 20 years. There are many normal transitional changes during this course of development that must be separated from the abnormal.
Development of the dentition is an integral part of craniofacial structure. Skeletal growth of craniofacial bony structures also interacts with the development of the dentition, which leads to normal interdigitation between the maxillary and mandibular dental arches. Any disruption of these complicated processes may affect the ultimate occlusal status. Appropriate actions are needed to restore the normal processes of occlusal development. Depending on the age of the patient and the stage of dentition, these treatment procedures may be preventive, interceptive, or corrective.
An understanding of the complex processes of the growth and development of the face and dentition can play an important role in recognition of dentofacial disharmony and help in diagnosis and treatment planning. The growth and changes that take place during the development of the dentition are also a basis for clinical application of early-age orthodontic treatment within the complex processes of occlusal development. An understanding of the structural components can help clinicians to recognize developing problems at their initial stages and to determine the proper time of intervention.
Therefore, a thorough grasp of all stages of development of this system is a must for any dental practitioner treating children who are in the developmental stages of occlusion.
The formation, eruption, and exfoliation of teeth; their changes from a deep position within the jawbones into the oral cavity; and the concomitant growth of bones comprise one of the most fascinating processes of biology. This chapter discusses different stages of tooth formation and eruption and developmental stages of occlusion at three different stages:
1. Prenatal stage
2. Neonatal stage
3. Postnatal stage
Prenatal Development of the Dentition
The embryonic period, weeks 3 to 8 of intrauterine life, is the most critical stage of developmental events. Development of the occlusion initiates during week 6 of intrauterine life and ends after 20 years of age. Neural crest cells begin migration during the embryonic period, forming most of the facial structure. Any interference with this migration can cause different craniofacial anomalies. By the end of this period, all of the main organ systems have begun to develop.
During the first 2 months of intrauterine life, the ectomesenchyme cells, from which the orofacial structures develop, undergo a complex and coordinated series of steps of proliferation and differentiation. At approximately 7 weeks of intrauterine life, a bandlike ectodermal thickening called the dental lamina develops in maxillary and mandibular processes. Odontogenesis (tooth development) begins with budding of the dental lamina and continues into the next week of life.
Massler and Schour1 have divided the life history of the primary dentition, from initiation of tooth formation until birth and exfoliation, into six major stages: (1) growth, (2) calcification, (3) eruption, (4) attrition, (5) resorption, and (6) exfoliation.
The growth stage, or tooth formation, consists of the following five subdivisions:
1. Initiation
2. Proliferation
3. Histodifferentiation
4. Morphodifferentiation
5. Calcification
Initiation stage
At this stage, the primitive oral cavity, or stomodeum, is lined by epithelium that consists of two to three cell layers. These cells cover the embryonic connective tissue originating from the neural crest. This is known as ectomesenchyme.
Epithelial thickening occurs after 37 days and is the first sign of development of the dentition.2
At this stage, certain cells of the basal layer of the oral epithelium proliferate at a more rapid rate than adjacent cells and form a horseshoe-shaped thickened band of epithelium around the mouth, called the primary epithelial band, which corresponds in position to the future dental arch (Fig 2-1). The primary epithelial band quickly gives rise to two subdivisions, known as the dental lamina and the vestibular lamina.
Fig 2-1 Epithelial thickening.
Dental lamina
The dental lamina develops by proliferation of the basal layer of the oral epithelium at a rate that is faster than that of adjacent cells and produces a thicker region extending along the entire free margin of the jaw (Figs 2-2 and 2-3).
Fig 2-2 Upper and lower dental lamina.
Fig 2-3 Higher magnification of the dental lamina.
Vestibular lamina
The vestibular lamina is another proliferation of the oral epithelium; it occurs outside of the dental lamina (buccally and labially). As a result of proliferation of the vestibular lamina into the ectomesenchyme, the vestibule develops and forms a separate plate between the cheeks, lips, and developing dental lamina. This separating plate rapidly degenerates and forms a sulcus, or vestibule, between the cheek and tooth area (Fig 2-4).
Fig 2-4 Vestibular lamina.
From this point, tooth development proceeds in three stages (bud, cap, and bell stages), and 10 round or ovoid swellings occur in each arch in the position to be occupied by the primary teeth.
Although these stages are discussed separately here, tooth formation is a continuous process, and a clear distinction between the transition stages is not possible.
Bud stage (proliferation stage)
Within the dental lamina, a localized proliferative activity leads to the formation of tooth buds as a series of 10 round or ovoid swellings of epithelial ingrowth into the ectomesenchyme, corresponding to the position of future primary teeth (Fig 2-5). According to Ten Cate,3 at this time the mitotic index, the labeling index, and the growth of the epithelial cells are significantly lower than corresponding indices in the underlying ectomesenchyme, which suggests that part of the “ingrowth” is achieved by ectomesenchymal upgrowth.
Fig 2-5 Proliferation stage (bud formation).
Within 6 to 8 weeks, 10 primary tooth buds develop from anterior to posterior. These proliferating cells contain the entire growth potential of the teeth. The permanent tooth germs of the incisors, canines, and premolars develop as a result of new extension and further proliferation of the buds of their primary predecessors, within the dental lamina, attached to the dental organ of the primary tooth germ, which leads to the formation of a new cap on the lingual aspect of primary germs (Fig 2-6).
Fig 2-6 Permanent tooth bud formation.
Permanent molars follow a different process, when the jaw growth and bone remodeling increase the body length of the jaw. The dental lamina burrows posteriorly beneath the epithelium of oral mucosa into the ectomesenchymal tissue and forms the first, second, and third molar germs.
Enamel knot
The enamel knot is a localization of cells on an enamel organ that appear thickened in the center of the inner enamel epithelium during the late bud stage of development at the site of the primary tooth cusps (see Fig 2-9).
According to Ten Cate,3 the function of this structure is not known, but it seems that it is involved in determining the initial position of the first cusp of the tooth during crown pattern formation.
Developing abnormalities at the bud stage
During the bud stage, abnormal proliferation, lack of initiation, or hyperactivity of the dental lamina can cause different abnormalities in tooth formation:
• Hypodontia: Congenital absence of a tooth can be the result of a lack of initiation or an arrest of the proliferation of dental lamina cells.
• Hyperdontia: Supernumerary teeth are the result of a hyperactivity of the dental lamina and continued bud formation of the enamel organ.
• Odontoma: During bud formation, and depending on the degree of differentiation from the enamel organ, a supernumerary tooth or a dysmorphic tooth tissue called an odontoma can be produced.
• Cyst formation: Excessive proliferation of cells can produce epithelial rests. When epithelial rest cells are stimulated and become active, they assume secretory functions, which can result in cyst formation.
• For additional detail on these phenomena, see chapters 7 and 8.
Cap stage (proliferation stage)
During this stage, proliferation of the cells continues. As a result of unequal growth and proliferation of different parts of the dental organ, a caplike configuration develops, and a shallow invagination appears on the deep surface of the bud. This consists of the ball of condensed ectomesenchymal cells, called the dental papilla, that later form the dentin and the pulp (Fig 2-7). Surrounding the enamel organ, the ectomesenchymal cells form the dental follicles (Fig 2-8). The peripheral cells of the cap later form the outer and inner enamel epithelium.
Fig 2-7 Early cap stage. A— dental follicle; B—dental papilla; C—enamel organ.
Fig 2-8 Early bell stage. A—enamel organ; B—outer enamel epithelium; C—inner enamel epithelium.
At this stage, each bud consists of three parts (see Fig 2-8): (1) the enamel organ, derived from ectoderm; (2) the dental follicle, derived from mesenchyme; and (3) the dental papilla, derived from mesenchyme.
Early bell stage (histodifferentiation stage)
At this stage, the epithelium continues to invaginate and deepen. The enamel organ changes from a caplike configuration to a bell-shaped form; dentinogenesis and amelogenesis both begin (Fig 2-9). Late in the cap stage, during the transition of the tooth germ from cap to bell, important developmental changes begin. This process is called histodifferentiation.
Fig 2-9 Enamel knot (arrow).
The enamel organ undergoes histodifferentiation, and the cells in the center of the dental organ continue to synthesize and secrete glycosaminoglycans into the extracellular space between the epithelial cells. Glycosaminoglycans are hydrophilic and absorb water into the dental organ.
The increasing amount of fluid increases the volume of the extracellular compartment of the dental organ. This causes the cells to separate while they are connected with each other by desmosomal contacts. Next, the stretched cells become star shaped and are then known as the stellate reticulum (Fig 2-10).
Histodifferentiation is actually the end of the proliferative stage, as the cells lose their capacity to multiply.
Fig 2-10 Histodifferentiation. A—stellate re-ticulum; B—outer enamel epithelium; C— stratum intermedium; D—ameloblasts.
Fig 2-11 Histodifferentiation. A—ameloblast; B—enamel; C—dentin; D—cytoplasmic extension; E—odontoblast.
Stratum intermedium
Some epithelial cells between the internal dental epithelium and the stellate reticulum differentiate into a layer called the stratum intermedium. This layer is composed of two to three layers of flattened cells. The stratum intermedium gives rise to ameloblasts at a later stage, which produce enamel (Figs 2-10 and 2-11). Although the cells of this layer are histologically distinct from the cells of the internal dental epithelium, both layers should be considered as a single functional unit responsible for the formation of enamel.4
The outer surface of the enamel organ consists of simple cubical cells called the external enamel epithelium or outer enamel epithelium. Stellate reticulum cells are connected to each other and to the cells of the external dental epithelium and stratum intermedium by attachment plaques known as desmosomes.
Odontoblasts differentiate under an organizing influence stemming from the cells of the internal dental epithelium. During this stage, the cells of the dental papilla differentiate into odontoblasts, and the cells of the internal dental epithelium differentiate into ameloblasts (Figs 2-11 and 2-12; see also Fig 2-6).
Fig 2-12 Morphodifferentiation. A—ameloblast; B—enamel; C— dentin; D—odontoblast.
Developing abnormalities at the histodifferentiation stage
Disturbances during the histodifferentiation stage of tooth formation cause abnormalities in the differentiation of the formative cells of tooth germs. This results in abnormal structures of the dentin or enamel:
• Amelogenesis imperfecta: Amelogenesis imperfecta is a clinical example of the failure of ameloblasts to differentiate properly to enamel at this stage.
• Dentinogenesis imperfecta: Dentinogenesis imperfecta is a clinical example of failure of the odontoblasts to differentiate properly to dentin during the histodifferentiation stage of tooth formation.
When the tooth germ is growing rapidly during the early bell stage, cell division occurs throughout the internal dental epithelium. As development continues, cell division ceases because the cells are beginning to differentiate and assume their eventual function of producing enamel.
Late bell stage (morphodifferentiation stage)
In this stage of tooth formation, the formative cells of ameloblasts and odontoblasts are arranged to outline the final form and size of the teeth. Until the completion of the bell stage, all cells of the internal dental epithelium are continually dividing and tooth germs are rapidly growing. At the end of this stage, the overall growth and final morphologic pattern of the tooth germ have been established.
The cessation of mitotic division determines the final shape and size of a tooth. This process occurs before matrix deposition.
When the inner enamel epithelium is arranged, the boundary between it and the odontoblast outlines the future dentinoenamel junction (see Fig 2-12).
Developing abnormalities at the morphodifferentiation stage
At the end of the morphodifferentiation stage, proliferation ends, and the morphology and size of the tooth germ reach their final form. Disturbances and aberrations occurring during the morphodifferentiation stage lead to teeth with abnormal forms and sizes. Some examples of these abnormalities are peg-shaped lateral incisors, mulberry molars, microdontia, and macrodontia.
Formation of the permanent dentition
The permanent teeth (successional teeth) also arise from the dental lamina, but not all permanent tooth formation is alike. Permanent incisors, canines, and premolars form as a result of extraproliferative activity from the dental organ of the primary teeth within the dental lamina. This extraproliferative activity leads to the formation of another epithelial cap located on the lingual aspect of the primary tooth germ that initiates the formation of the permanent tooth bud (see Fig 2-6).
The tooth germs of the first, second, and third permanent molars, which have no primary tooth predecessor, originate in a different way. When jaw growth and bone remodeling increase the body length of the jaw, the dental lamina burrows posteriorly beneath the epithelium of the oral mucosa. This, and the associated ectomesenchymal response, forms the tooth germs of the first, second, and third molars. This backward extension of dental lamina into the ramus area develops from the mandibular body, which has a more horizontal position at this age.
Occasionally, insufficient mandibular growth or insufficient resorption of the anterior border of the ramus during remodeling results in a space deficiency that can lead to third molar impaction in the bony ramus of the adult mandible.
Hard tissue formation
Dentin and enamel are two principle hard tissues of the tooth that form during the late bell stage. Dentin, as the bulk-forming tissue of the tooth, is a specialized hard connective tissue; dentin formation always precedes enamel formation and marks the onset of the crown stage of tooth development.
Overall growth of the tooth germ continues by cell division of all the cells of the internal dental epithelium until the completion of the bell stage (Fig 2-13).
Fig 2-13 Early crown formation.
The first area of dentinogenesis appears at the future cusp tip, or the growth center, where mitotic cell division ceases. The internal dental epithelial cells differentiate and assume their role in producing enamel. The occurrence of second, third, and more zones of maturation within the internal dental epithelium lead to the final cuspal pattern of the tooth.
Role of the enamel knot in cusp formation. The enamel knot is a localized thickening of cells that appears in the center of the inner enamel epithelium of the enamel organ during the late bud stage of development. This corresponds to the site of the primary tooth cusps (see Fig 2-9). Vaahtokari et al5 concluded that the enamel knot acts as a signaling center, responsible for directing cell proliferation and subsequent cuspal morphogenesis in the developing enamel organ. The enamel knot is seemingly necessary for morphogenesis of the tooth germ to progress from the bud to the cap stage. The ectomesenchyme eventually assumes the dominant role in determining the final pattern of the cusps and the shape of the teeth.
Dentinogenesis. Dentinogenesis, or dentin formation, in the crown stage is the first identifiable feature of tooth development, which starts at the tip of future cusps. At this time, mitotic activity ceases, and the ectomesenchymal cells increase rapidly in size and differentiate into odontoblasts. Odontoblasts are the dentin-forming cells.
Odontoblasts start collagen formation and elaborate the organic matrix of dentin by formation of collagen fibers and ground substance. Mineralization takes place following matrix deposition and involves the precipitation of inorganic calcium salts.
When organic matrix is deposited, the odontoblasts move toward the center of the dental papilla, leaving behind a cytoplasmic extension called the odontoblastic process. The odontoblastic process is embedded in mineralized dentin tissues, and the tubular character of dentin is established. Dentin formation proceeds toward the inside of the dental papilla and odontoblastic process and results in the secretion of hydroxyapatite crystals and mineralization of the matrix (see Fig 2-11).
Amelogenesis. Although dentin must be present for enamel formation, within the enamel organ the preameloblasts differentiate before the odontoblasts and have an inductive influence on the odontoblasts, sending a message that causes them to differentiate and secrete dentin. Ameloblasts also require the signal of dentin formation to initiate their secretory activities. A message is sent from the newly differentiated odontoblasts to the inner enamel epithelium, causing further cell differentiation and activating secretory ameloblasts to form enamel tissue. This prerequisite is an example of the biologic concept termed reciprocal induction.
Enamel formation generally occurs in two stages: (1) the secretory stage and (2) the maturation stage. In the first stage of amelogenesis, the function of the ameloblasts is to secrete and release enamel proteins into the surrounding area and produce an organic matrix against the newly formed dentin surface. Ameloblasts immediately become mineralized by alkaline phosphatase enzymes and form the first layer of enamel tissue, causing a separation of the ameloblastic cells from the dentin and leaving enamel tissue behind (see Fig 2-6).
Initial mineralization of enamel matrix takes place almost simultaneously with organic matrix production; an unmineralized organic matrix cannot be found.
In the maturation stage, ameloblasts change their function from enamel matrix production to mineralization. Mineralization takes place by transferring substances used in the formation of enamel. In this stage, most of the materials transported by ameloblasts are proteins used to complete mineralization.
Mineralization of dental hard tissues (calcification stage)
The production of mineralized tissues involves two major processes, apposition and calcification.
Apposition
As explained earlier, at the morphodifferentiation stage, enamel and dentin tissues are initially secreted by ameloblasts and odontoblasts as a nonvital extracellular secretion in the form of a tissue matrix. The tissue matrix is deposited layer by layer along the future dentinoenamel and dentinocemental junctions. The organic matrix for enamel tissue is not collagenous, but the organic matrix for dentin, cementum, and bone is collagenous. The organic matrix for enamel comprises mainly unique proteins (mostly amelogenin), reflecting the tissue’s epithelial origin. The organic matrix in dentin forms a substantial part of the mineralized tissue.
The organic matrix of enamel is removed during the final stage of mineralization and leaves less than 1% of the weight of organic matrix. Matured enamel organic matrix is replaced with hydroxyapatite. Thus, enamel is physically different from dentin and cementum because it is formed from a noncollagenous matrix (mostly amelogenin) that is almost completely removed after mineralization.
As already mentioned, the formation of dentin always precedes enamel formation and marks the onset of the crown stage of tooth development. At this stage, the dental lamina is disintegrating, so the tooth germ now continues its development separated from the oral epithelium. The crown pattern of the tooth is established by folding of the internal dental epithelium. This folding reduces the amount of stellate reticulum over the future cusp tip. Dentin and enamel have begun to form at the crest of the folded internal dental epithelium. This stage is associated with the formation of the dental hard tissues, commencing at about the 18th week (Fig 2-14).
Fig 2-14 Crown stage. A shows the enamel space that has been lost during slide preparation. B— dentin; C—dental papilla.
Calcification
The second stage of dental hard tissue formation, like other hard tissues, is calcification or mineralization. This process takes place following the matrix deposition by precipitation of inorganic calcium salts (primarily in the form of calcium hydroxyapatite crystals) within the deposited matrix.
The mechanism involved in mineralization is not completely understood, especially in the first step, the formation of first crystals. According to Berkovitz et al,4 any tissue could calcify after crystal formation because blood plasma is supersaturated with calcium and phosphate ions, meaning that mineralization is not limited by the supply of basic ions. An example of this phenomenon is the pathologic calcification that can occur in soft tissues (eg, muscle and tendon).
The structural unit of calcified tissues is the crystal or crystallite, which has a basic shape in all four calcified tissues (enamel, dentin, cementum, and bone). The sizes of the crystals within these tissues are all different.
Calcification of cartilage, which is controlled by the cells, is another mechanism that seems to be occurring in the initial formation of dentin and bone. These cells form small matrix vesicles that contain calcium and phosphate ions, alkaline phosphatase, and calcium-binding lipids. These vesicles separate from the cells, and conditions within the vesicles permit formation of hydroxyapatite crystals.
Precipitation of small niduses of crystals continues with precipitation of further niduses (calcospherites) spreading around the original nidus and increasing its size by the addition of concentric lamination. Finally, through approximation and fusion, these individual calcospherites transform into a homogenously mineralized layer of tissue matrix.
After the secretory phase of amelogenesis is completed and the ameloblasts enter the maturation stage, they perform a functional and structural task, removing proteins and water from the maturing enamel and allowing them to achieve complete mineralization. Once the enamel is completely mineralized, the ameloblasts shrink from columnar to cubical or flattened cells but remain a part of the reduced enamel epithelium that forms a more or less continuous lining over the completed enamel.
Figure 2-14 shows the crown stage of tooth development at a point when hard tissue formation is well advanced.
Developing abnormalities at the mineralization stage
Any systemic disturbance or local trauma that injures the ameloblastic cells during enamel formation can interrupt or arrest matrix apposition, resulting in enamel hypoplasia. These disturbances include tetracycline deposition, which causes yellow to brown hypoplastic enamel, and dental fluorosis, which results when an individual receives too much fluoride during tooth development.
Dentin hypoplasia is less common than enamel hypoplasia and occurs only after severe systemic disturbances. If the calcification process is disturbed, there is a lack of fusion of the calcospherites. These deficiencies are not readily identified in enamel, but they are evident microscopically in dentin and are referred to as interglobular dentin.
Root formation
After enamel formation of the crown is complete and the crown is fully formed, root formation begins, and the inner and outer dental epithelium reaches and joins at the rim of the dental organ. This junctional zone is known as the cervical loop (Fig 2-15).
Fig 2-15 Root formation.
As epithelial cells of the external and internal dental epithelium continue proliferation, the cervical loop forms a double layer of cells known as Hertwig’s epithelial root sheath (Fig 2-16).
Fig 2-16 Hertwig’s epithelial root sheath.
This epithelial sheath grows around the dental papilla, between the papilla and the dental follicle, and covers the entire dental follicle except the basal portion of the papilla that encloses the primary apical foramen. Hertwig’s epithelial root sheath is responsible for the shape and number of root formations.
The dental follicle lies external to the root sheath and forms cementum, periodontal ligament (PDL), and alveolar bone. Root development involves interactions between the dental follicles and the epithelial root sheath. The onset of root development coincides with the axial phase of tooth eruption.
As the inner epithelial cells of the root sheath progressively enclose more and more of the expanding dental papilla, they initiate the differentiation of odontoblasts from cells at the periphery of the dental papilla. These cells eventually form the dentin of the root. In this way, a single-rooted tooth is formed. Multirooted teeth are formed in the same way, and all teeth undergo the same process. It is still not clear, however, how various crown shapes develop.
Molecular level of tooth development
The main objective in discussion of the molecular level of tooth development is to give an overview of the more established theories on the molecular regulation of tooth development and to briefly introduce some recent advances.
The prechordal mesoderm, the craniofacial ectoderm, and the neural crest cells that develop on the dorsal side of the neural tube play a complex interaction in the formation of all craniofacial structures. The migration of neural crest cells begins cranially and gradually extends caudally, ultimately differentiating into a wide variety of adult structures.
Neural crest cells are derived from the ectoderm, but because of their importance in ectodermal-mesenchymal interaction, they are called the fourth germ layer. Neural crest cells express homeobox genes in the mandibular and maxillary arches for orofacial development. Homeobox genes are a large group of genes that code for transcription factors responsible for regulating the expression of downstream target genes. A homeobox is a DNA sequence found within genes that are involved in the regulation of patterns of morphogenesis in animals, fungi, and plants.
The different stages of tooth formation and morphologic changes, including bud, cap, and bell stages and terminal differentiation, have been known for several decades, but the molecular interactions involved in this process have been researched widely only in the past two to three decades. In this process, a series of inductive and reciprocal signals between the epithelium and mesenchyme determine the number, growth, morphology, and the ultimate differentiation of craniofacial and tooth tissues and organs.
Development of the mammalian teeth as a model system has been intensively studied for epithelial-mesenchymal interactions during organogenesis, and progress has been made in identifying key molecules involved in this signaling. It is known that the morphogenesis and cell differentiation in developing teeth are governed by interactions between the oral epithelium and neural crest–derived ectomesenchyme. It has also become evident recently that the inductive interactions between epithelial and mesenchymal cells that operate in these processes are the same signaling molecules involved in the development of most vertebrate organs.
Coin et al6 reported that bone morphogenetic protein 2 (BMP-2), combined with apatite, could induce both morphologic and functional differentiation of ameloblasts using the in vitro culture system. BMPs are known to be a group of growth factors and cytokines that have the ability to induce the formation of bone, tooth, and cartilage. BMPs are also available commercially as oral supplements.
Fibroblast growth factors (FGFs), a family of growth factors involved in embryonic development, have been implicated as regulators of mesenchymal gene expression and cell proliferation during tooth initiation, epithelial folding morphogenesis, and the establishment of tooth shape (specifically FGF-4, -8, and -9). Kettunen et al7 analyzed the roles of FGF-3, -7, and -10 in developing mouse teeth. They found that FGF-10 stimulated cell proliferation in the dental epithelium and that FGF-3 may participate in signaling functions of the enamel knot. They concluded that the expression patterns and interactions of FGFs in dental epithelium and mesenchyme suggest their involvement in regulatory signaling pathways during tooth development.
Tompkins8 stated that the FGF, BMP, hedgehog, and wingless protein families and their downstream transcription factors have been identified as key players in the epithelial-mesenchymal signaling loops that drive tooth development. He also added that recent results suggest that phenotypic proteins of both ameloblasts and odontoblasts, such as amelogenin and dentin matrix protein 2 (DMP-2), may act as the final instructive signals in cytodifferentiation. Amelogenin is a protein found in the developing tooth enamel, and it belongs to a family of extracellular matrix proteins that constitute 90% of enamel proteins.
DMP is an extracellular matrix protein that is critical for proper mineralization of bone and dentin. DMP is primarily present as a nuclear protein in an undifferentiated osteoblast that regulates the expression of osteoblast-specific genes; during osteoblast maturation, it becomes phosphorylated and is exported to the extracellular matrix, where it coordinates mineralized matrix formation. Mutations in the DMP gene are known to cause autosomal-recessive hypophosphatemia, a disease that manifests as rickets and osteomalacia.
Advancements in molecular biology approaches, coupled with mapping of the complete human genome sequence, have shown a number of putative disease genes and loci to be associated with the hypodontia and oligodontia phenotypes. Missing maxillary lateral incisors along with the lack of BMP4 expression were reported by Neubuser et al9 to be the manifestation of odontogenic potential shifts from the epithelium to the dental mesenchyme.
Family studies by Burzynski and Escobar10 have established that incisor and premolar hypodontia is inherited via an autosomal-dominant gene that demonstrates incomplete penetrance. Expression of the human MSX1 and MSX2 genes in relation to hypodontia, affecting one or a few teeth, has recently been investigated by many researchers, including Nieminen et al.11
Formation of tooth-supporting tissues
While roots are forming, the supporting tissues of the teeth, such as cementum, PDL, and alveolar bone, are also developing from the dental follicle in the late stages of tooth formation.
The dental follicle (sac) is a condensation of the ectomesenchymal cells that initially surrounds the enamel organ and the enclosed dental papilla. ElNesr and Avery2 stated that the dental follicle has an important role in the initiation, formation, and maintenance of the tissues of the tooth root, PDL, cementum, and alveolar bone.
Cementum
Cementum formation, or cementogenesis, occurs late in tooth formation, just before the degeneration of the epithelial root sheath and root dentin deposition. It manifests as a thin, amorphous, structureless, and highly mineralized secretion on the surface of the root dentin. ElNesr and Avery2 noted that this secretion is more evident in the apical region of the root and averages some 10 to 20 μm in thickness.
After root formation and proliferation of the epithelial root sheath, some fragmentation occurs. Ectomesenchymal cells of the dental follicle penetrate between the epithelial fenestrations and become closed to the newly formed dentin of the root. The cells differentiate into cementum-forming cells, or cementoblasts. Cementoblasts secrete an organic matrix, consisting of fine collagen fibrils, ground substance, osteocalcin, and sialoprotein, that is mineralized later by minerals in the oral fluid (Fig 2-17).
Fig 2-17 Formation of tooth-supporting tissue.
Following mineralization, the cementoblasts move away from the cementum and collagen deposition continues to lengthen and thicken the bundle fibers. Finally, the surface fibers join the forming PDLs and become anchored with them.
During cementogenesis, two types of cementum form: (1) acellular cementum and (2) cellular cementum. Acellular cementum forms first, in the coronal two-thirds of the root. When the cementoblasts are trapped in lacunae in their own matrixlike bone cells, the cementum is called cellular or secondary cementum. This cementum is present only in the apical third of the root. At this time, the cementoblasts lose their secretory activity and become cementocytes. Cellular cementum develops after most of the tooth formation is complete and after contact is established with a tooth in the opposing arch.
According to Ten Cate,3 cellular cementum is not found in teeth with one root; in premolars and molars, it is found only in the part of the root closest to the apex and in interradicular areas between multiple roots.
Periodontal ligament
The supporting tissues of the tooth develop during root formation from the dental follicle, a fibrocellular layer investing the dental papilla and enamel organ. The cells and the fiber bundles of the PDL differentiate from the primitive dental sac or dental follicle (Fig 2-18).
Fig 2-18 Principal collagen fiber bundles of the PDL.
When the root sheath fragments, ectomesenchymal cells of the dental follicle penetrate between the epithelial root sheath and the newly formed dentin of the root. These cells differentiate into cementum-forming cells (cementoblasts); according to Ten Cate,3 some cells from Hertwig’s epithelial root sheath may also transform into cementoblasts. These cells elaborate an organic matrix that becomes mineralized and in which collagen fiber bundles of the PDL become anchored.
The dental follicle also differentiates the cells and fiber bundles of the PDL. Recent evidence indicates that dental follicle cells also form the bone in which the ligament fiber bundles are embedded.3
Fibroblasts of the dental follicle also secrete collagen fibers, which interact with fibers on the surface of adjacent bone and cementum. This interaction between the alveolar socket and the tooth root is believed to help the process of tooth eruption.
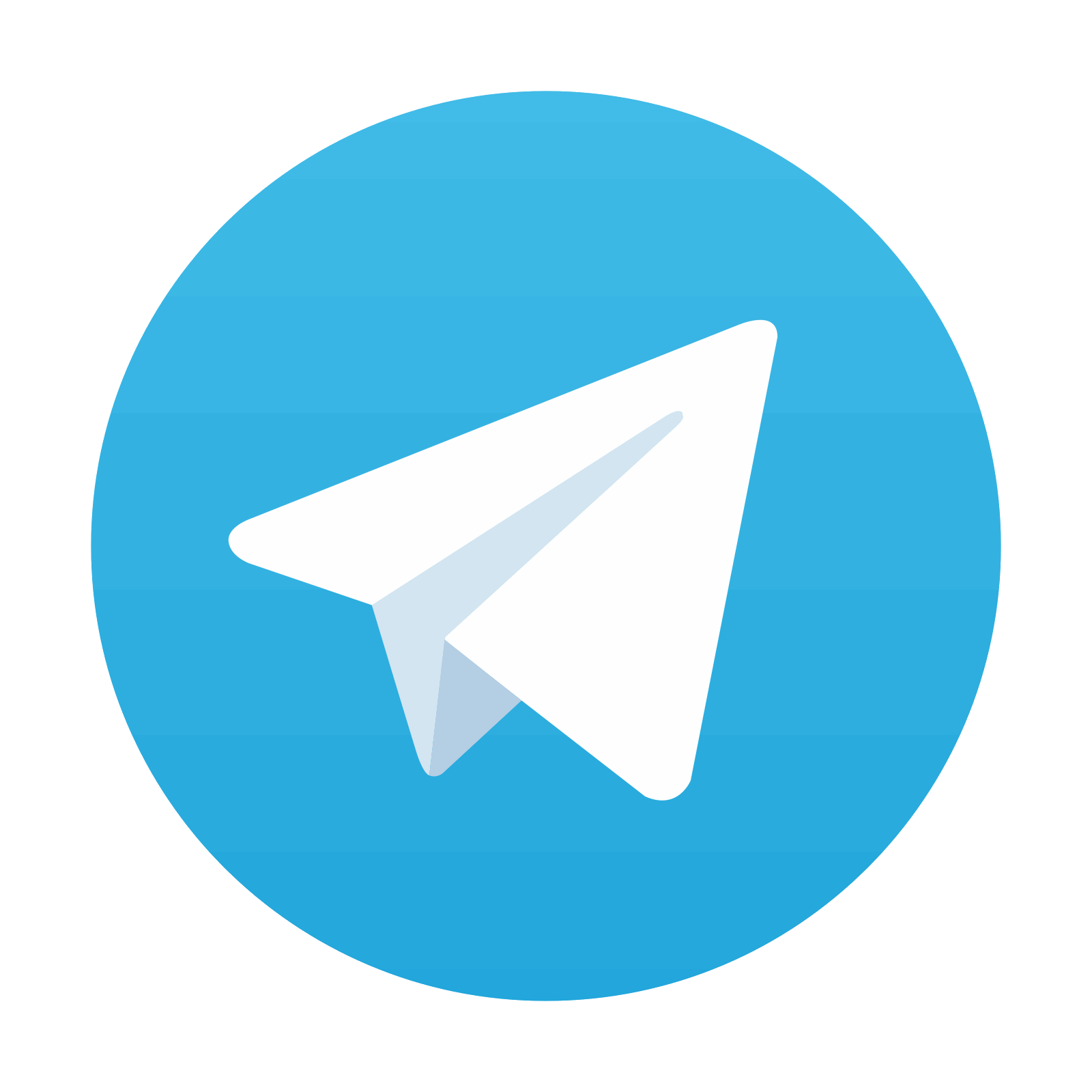
Stay updated, free dental videos. Join our Telegram channel

VIDEdental - Online dental courses
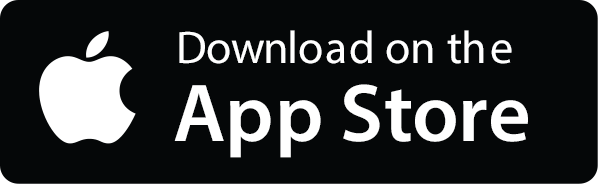
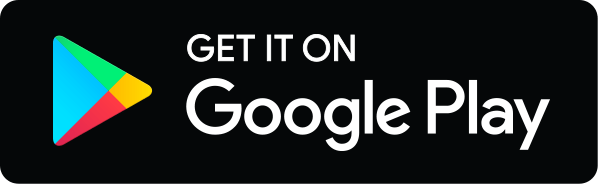