Fig. 15.1
Severe, untreated dental caries. Dental caries initially affects the occlusal pits and fissures of posterior teeth. In severe caries, the smooth surfaces, especially the interdental surfaces of all teeth may be involved
15.1.1 Sugar, Dental Caries, and the Dental Profession
Because teeth are preserved long after death, it was evident that European populations suffered little from caries before the late eighteenth century. The rise of caries coincided with increased sucrose consumption; perhaps, the revenge of black slaves on their masters. Before the civil war ended in 1863, slaves from Africa were forced to cut cane sugar in the West Indies and far Southern US. From the late eighteenth century and throughout the nineteenth century, increasing amounts of the raw cane were exported to Atlantic-facing ports of England and Scotland. There, in sugar refineries, it was crushed, cleaned, and the fluid crystallized to give pure sucrose, refined sugar. During this period, new methods increased the yields of corn, wheat, and other grains and their starch was purified (refined) to add to various foods. Pure starch and sucrose are called refined carbohydrate. Populations in Europe, Canada, and the Northern US became addicted to refined carbohydrate because of the cold climate. The dramatic increase in caries throughout the nineteenth and early twentieth centuries led to the development of local and general anesthetics and of the dental profession to treat the associated infections, pain, and discomfort.
In 1945, the Vipeholm study was set up to determine whether increasing sucrose intake actually increased human caries experience. The marked variation in caries severity between individuals (Sect. 15.2.1) had led to doubts that increased sucrose consumption was really responsible. The study was conducted at the Vipeholm Hospital for individuals with mental handicaps outside the University City of Lund, Sweden. The study examined the effects of different diets on dental caries in the inmates and it ended in 1951. The results indicated that sticky sugar candies (toffees) between meals and popular with the inmates increased their DMFT by an average of one cavity per year. The use of mentally handicapped subjects was criticized in the Swedish press and all studies on mentally handicapped individuals were stopped in 1954.
Another series of studies was conducted on the inmates of Hopewood House, Bowral, New South Wales, Australia. This home for children of unmarried mothers opened in 1942 and closed in 1965. The children were made to eat a diet of locally grown raw vegetables with a minimal amount of protein in the form of milk and raw soybeans. The almost complete absence of dental caries compared with other Australian children was noted in studies conducted between 1955 and 1960. Unfortunately, as soon as they entered the general population as adults, all former inmates developed caries rapidly. Moreover, a harrowing description of what this diet was like, and of many other violations by the owners and employees of this orphanage, was given to the Australian Senate by Sandra Pendergast in 2003. In November 2009, the Australian government issued a formal apology to those who had grown up suffering abuses that were common to this and many other orphanages in Australia.
Today, cane sugar is mostly grown and exported throughout the world from the eastern half of Africa south of the Equator, and from India and Indonesia. The most compelling evidence for sucrose causing caries was obtained by comparing the sucrose intake in 12-year old children from 47 countries (obtained from the World Health Organization’s Global Oral Epidemiology Bank during the 1970s) with the DMFT during this period (Fig. 15.2). The number of affected teeth increased by about one DMFT for every 25 g of sugar consumed daily. About 50% of the variance in DMFT between populations is due to the daily sucrose intake.
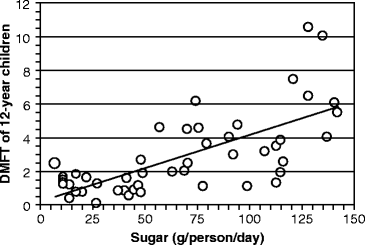
Fig. 15.2
Relationship between dietary sucrose intake and dental caries severity. The number of teeth that were decayed, missing, and filled due to caries (decayed, missing, and filled teeth [DMFT]) is graphed against mean sugar consumption in 12-year old children. Each point on the graph represents a different country. The findings were available from World Health Organization activities in oral epidemiology (The graph was assembled by the author from Table 3 in L. M. Sreebny (1982) “Sugar availability, sugar consumption and dental caries.” Community Dentistry and Oral Epidemiology 10:1–7)
15.1.2 Sucrose and the Appearance of Acid in Dental Biofilms
Bones and teeth dissolve in acid. The insoluble calcium monophosphate salt, from which hydroxyapatite is made, is converted to the more soluble calcium dihydrogen phosphate salt in an environment whose pH is less than 6.2 (Sect. 9.1.1). The severity of caries was related to the pH produced in dental biofilms (plaques) after ingesting sucrose and other sugars by Richard M Stephan. The pH response he identified is referred to as Stephan Curve. He found that the starting pH, the extent of its drop, and the time for recovery to the starting pH were all related to caries severity. The pH drop was later associated with lactic acid production due to bacterial carbohydrate fermentation (saccharolytic fermentation, Sect. 1.3.2). The subsequent rise in pH was due to the production of ammonia by bacterial asaccharolytic fermentation after the sucrose was depleted (see Sect. 15.3.1). The curve was originally shown to occur after a 10% glucose mouth rinse, but a sucrose mouth rinse produces a similar result (Fig. 15.3).
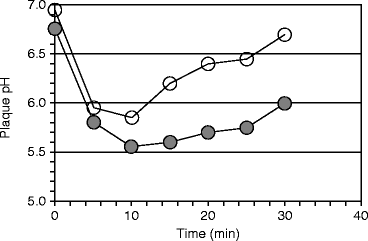
Fig. 15.3
Biofilm (plaque) pH in caries-resistant and caries-susceptible subjects. The pH decreased and returned to initial values more rapidly following a 10% sucrose rinse in caries-resistant subjects (○) than in caries-susceptible subjects (●). Each group had five subjects (Figure 2 in Mandel and Zengo (1973) “Genetic and chemical aspects in caries resistance.” Comparative immunology of the oral cavity, ed. H. Scherp and S. Mergenhagen, pp. 118 – 137, US Government Printing Office)
15.1.3 Cavities in Animals and Streptococcus mutans
In rodents, caries induction was unpredictable until a high-carbohydrate low-fat diet (∼60% sucrose plus soy and milk proteins plus vitamins and minerals) was developed. Germ-free rodents fed this diet did not develop caries and normal rodents (rats, hamsters, and mice) also did not develop caries when their diet was supplemented with penicillin (0.01–0.05% in the drinking water). Penicillin inhibits the growth of gram-positive bacteria by interfering with cell wall formation (Sect. 1.4.1). Young mice fed the diet without penicillin developed rampant caries within 35 days, but no caries in the presence of penicillin. If the penicillin was removed from the drinking water after one generation, the mice produced several generations of progeny that developed little caries despite the diet, suggesting that the absence of gram-positive bacteria resulted in a stable microbiota associated with caries resistance. These experiments are illustrated in Fig. 15.4.
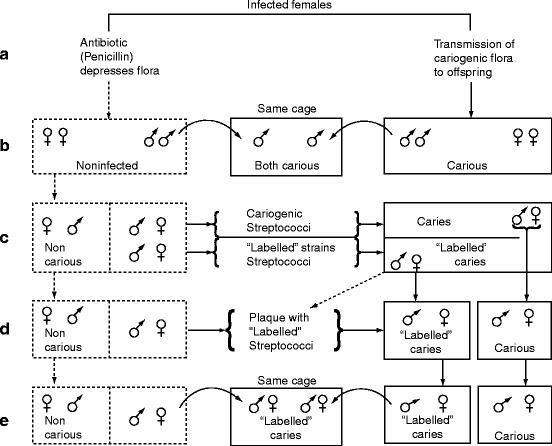
Fig. 15.4
Identification of a cariogenic microbiota – the Fitzgerald–Keyes experiments. (a) Penicillin inhibits bacterial colonization. When penicillin was added to the drinking water, gram-positive bacteria were depleted (depressed) and caries did not develop. (b) Caries is transmissible. After a generation, penicillin was no longer provided, but the offspring remained caries resistant to the sucrose diet for many generations (left side of figure). The caries-resistant rodents developed caries only if they were placed in the same cage as the animals that did develop cavities (not receiving penicillin). (c) Adding streptococci to the caries-resistant microbiota causes caries. Strains of what were later called mutans streptococci were isolated. Some were labeled by being made streptomycin resistant. Oral inoculation of the unlabeled or streptomycin-resistant strains into caries-resistant animals caused them to develop caries. (d) Adding streptomycin-resistant “labeled” strains to biofilm (plaque) from caries-resistant males or females cause caries. (e) These streptococci cause caries even if obtained from feces. The streptomycin-resistant streptococci in feces smeared on the body of a caries-resistant rodent caused the animal to develop caries (Figure 3 in Fitzgerald RJ and Keyes PH (1965 Oct) “Dental caries as a major disease problem.” Medical Annals of the District of Columbia 34(10):463–467)
In 1960, Robert Fitzgerald and Paul Keyes demonstrated the types of bacteria responsible for caries. They isolated streptococci from a carious lesion and made several of the isolates resistant to a non-penicillin antibiotic (streptomycin) which provided them with a means of isolating and identifying these bacteria subsequently. The streptomycin-resistant strains were used to infect young hamsters that were resistant to caries despite the diet. The infected hamsters developed caries and the streptomycin-resistant bacteria appeared in their oral cavity and feces. These bacteria remained absent from separately caged caries-resistant animals fed the same diet in the same room. Thus, the caries-susceptible microbiota is composed of streptococci and is transmitted by direct contact, not aerosols (Fig. 15.4). The streptococci were later identified as Streptococcus mutans. Although a caries-resistant microbiota is stable in the presence of a sucrose diet, the procedures required to develop and maintain it are impracticable for human populations. Removing sucrose from the diet or adding fluoride to the diet markedly decreased caries development in rodents possessing the cariogenic microbiota (flora).
Dental caries (cavities or tooth decay) led to the development of the dental profession by the late nineteenth century. It is caused by refined carbohydrates, pure sucrose, and starch in the diet. Sucrose between meals, especially sticky candies, increased cavities in most adults, and raising children on a diet without refined carbohydrate prevented caries development. A World Health Organization study showed that the average number of carious teeth in populations of 12-year old children from 47 countries increased by one for every 25 g of sugar consumed daily. Experiments in rodents confirmed that a high sucrose diet caused caries and that the responsible microbiota was sensitive to penicillin. Administering penicillin to the drinking water protected the rodents from developing caries despite the diet. Caries resistance was transmitted after removing penicillin by keeping the offspring isolated from caries-susceptible rodents despite the diet. The caries-resistant rodents developed caries if they came into direct contact with penicillin-sensitive streptococci subsequently identified as Streptococcus mutans from caries-susceptible rodents.
15.1.4 How Sucrose Connects S. mutans to the Oral Microbiota and Dental Caries
By the late 1960s, the rodent studies had led to dental caries being generally accepted as caused by refined carbohydrate promoting a saccharolytic gram-positive microbiota that adhered to teeth surfaces. Mutans streptococci were considered key components of this microbiota. The name “mutans” is derived from the Latin word for change, “mutatio.” On agar, Streptococcus mutans grows as small round colonies in the presence of glucose, but as large, sticky, gelatinous colonies in the presence of sucrose. The gelatinous colonies are caused by a capsule around the bacteria: mutan, an α1→3 glucose polymer (Fig. 15.5), and dextran, an α1→6 glucose polymer. Mutan and dextran are glucans (made from glucose) like starch, glycogen, and cellulose. Functionally, the most important portion of the S. mutans capsule is its mutan. The aqueous solubility of a glucan depends on its major bonds; cellulose (β1→4) and mutan (α1→3) are mostly insoluble, whereas dextran (α1→6), glycogen, and starch (α1→4) are mostly soluble.
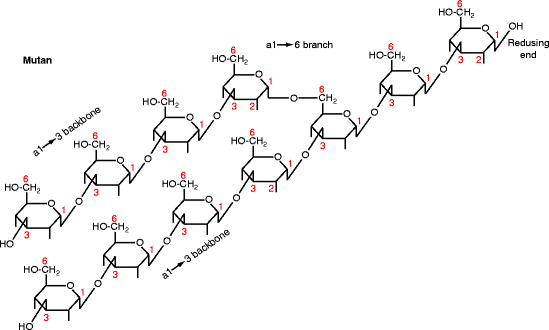
Fig. 15.5
Structure of mutan. Diagram shows the glucose pyranose ring with extensions indicating the OH group positions. The carbon atoms are numbered. The residues are linked together by α1→3 bonds with α1→6 branching (shown) and occasional α1→2 branching (not shown). The reducing end is at the top right. Mutan is the product of GTF-I
In sucrose, the glucose and fructose moieties are connected by their respective anomeric carbon atoms (Fig. 15.6) The alpha anomer predominates in glucose, whereas the beta anomer predominates in fructose (indicated by the larger size of the predominant form in Fig. 15.6 c compared with b). The hydrolysis of sucrose releases two anomeric carbon atoms that provide the energy for bacterial capsule formation at the stagnation areas where caries develops. Glucan capsules (i.e. mutan and dextran) are made from the glucosyl moiety of sucrose. Some oral bacteria produce instead a capsule called levan from the fructose moiety of sucrose (Fig. 15.7). The enzymes that synthesize these polysaccharides, glucosyl- and fructosyl-transferases
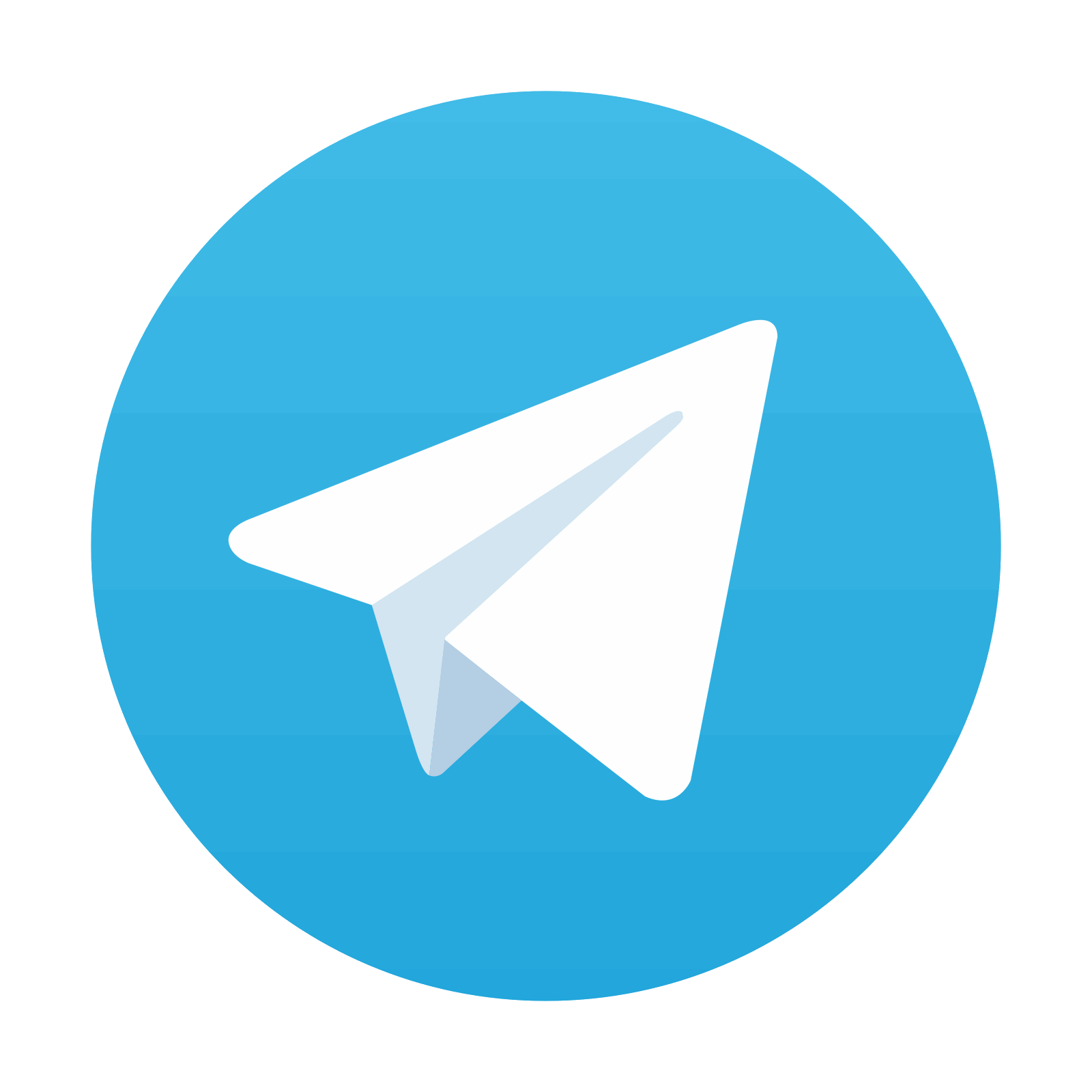
Stay updated, free dental videos. Join our Telegram channel

VIDEdental - Online dental courses
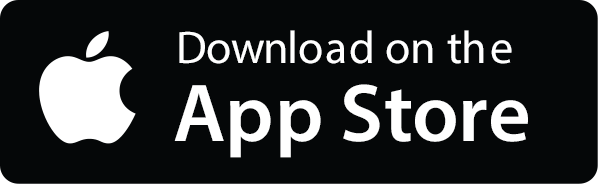
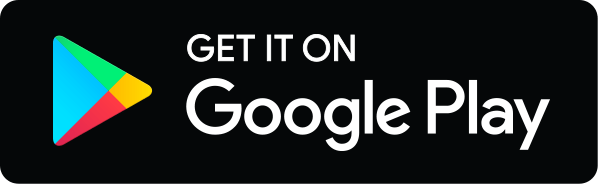