Abstract
Objectives
Clinical experience with implant-supported dentures indicates that fracture and chipping of teeth are becoming an issue. Tooth fracture and chipping rates of approximately 2.5% per year are being experienced at one university. There has been no standardized test developed for bulk fracture or chipping of denture teeth. Such a test would aid in the development of improved teeth and in their evaluation.
Methods
Central incisor teeth were embedded in acrylic and loaded on incisal edges at 90° to their long axes. Teeth tested included ones commercially available and two sets made from improved materials designed to increase toughness. Cyclic loading was done at 5 Hz from 20 N to 135 N, 150 N, 175 N and 200 N. Data was analyzed using lifetime analysis software fit at each of the accelerated loads and then extrapolated to clinical use loads (Alta 7, Reliasoft Corp.) Clinical use loads were derived from extrapolation of probability of failure (Pf) data to 2.5% Pf.
Results
When carefully embedded, teeth could be reproducibly loaded to failure by bulk fracture involving a failure mode similar to that seen clinically. Clinical use loads were calculated to be in the range of 70 N. Results from accelerated loading could be fit to similar probability of failure distributions allowing extrapolation to clinical use loads.
Significance
This work was able to develop a clinically valid bulk fracture test for the fatigue failure of incisor denture teeth. It appears that teeth fabricated with improved materials will be expected to perform better clinically. Thus both the null hypotheses were rejected.
1
Introduction
Traditional dentures consist only of the artificial teeth embedded in denture acrylic, fitted to the residual maxillary and mandibular ridges. Such prosthesis can help to regain an esthetic appearance and a natural pronunciation, but the maximal achievable bite force amounts to only about one fifth to one fourth of the maximal bite force of subjects with natural dentition .
To improve this handicap, it has become common to support denture prosthesis with implants. According to Fontijn-Tekamp et al. the maximal bite force at the incisors of patients with denture teeth can be increased from about 50 N without implant-support to about 70 N with implant-support . Implants additionally greatly increase denture stability and retention during function.
However, increasing the biting force also increases the stress the teeth are subjected to resulting in a more frequent occurrence of fractured teeth ( Fig. 1 ). These pictures show bulk fracture, which is the main subject of this paper. Chipping, the second common failure mode within denture prosthetics is likely related to bulk fracture so that improvements in one should reflect in improvement in the other.

Many investigators have examined the “bond strengths” of plastic denture teeth to acrylic denture bases . International and national standards for denture teeth do not test teeth for fracture; they only test the bond between teeth and denture-base acrylic . These standards attempt to utilize either shear loading or tensile loading to separate teeth from base acrylic. None of these standards or literature examines bulk tooth fracture or partial chipping as is commonly now encountered with implant-borne prostheses.
Only Moffitt et al. appear to have directly examined fracture of denture teeth themselves versus pullout of teeth from the denture base . Moffitt et al. compared the failure modes of three different denture teeth with point loading at a 30° off-axis angle . Point loading seems not to be an appropriate protocol since it is not a clinically relevant load. Point loading results in a failure by Hertzian cone contact damage rather than by bulk fracture from the portion of the tooth at or near its level of embedment in the denture acrylic, as is evidenced in Fig. 1 .
To get insights into the failure mechanisms, denture teeth of three different compositions were tested and compared under blunt fatigue loading at a series of different loads until failure or run-out (i.e. no failure by a specified number of cycles). The first tooth tested was the commercially available SR Phonares NHC (Ivoclar Vivadent, Schaan, Liechtenstein). The second and third sets of teeth were fabricated by the same manufacturer using the same molds, but using two different improved materials, also developed by Ivoclar Vivadent. Properties of these denture tooth compositions are provided in Table 1 , with the improvements appearing to be done to make the teeth less brittle.
Phonares | Facial enamel and dentin | Cervical, palatal enamel |
---|---|---|
Flexural strength in MPa | >125 | >120 |
Modulus of elasticity in MPa | >4500 | >3000 |
Ball indentation hardness in MPa | >240 | >170 |
Water sorption in μg/mm 3 | <32 | <26 |
Water solubility in μg/mm 3 | <0.1 | <0.1 |
Vickers hardness HV 0.5/30 in MPa | >260 | >190 |
Improved material 1 | Facial enamel and dentin | Cervical, palatal enamel |
---|---|---|
Flexural strength in MPa | >100 | >120 |
Modulus of elasticity in MPa | >3800 | >3000 |
Ball indentation hardness in MPa | >200 | >170 |
Water sorption in μg/mm 3 | <32 | <26 |
Water solubility in μg/mm 3 | <0.08 | <0.1 |
Vickers hardness HV 0.5/30 in MPa | >240 | >190 |
Improved material 2 | Facial enamel; dentin | Cervical, palatal enamel |
---|---|---|
Flexural strength in MPa | >100; >120 | >120 |
Modulus of elasticity in MPa | >3800; >4200 | >3000 |
Ball indentation hardness in MPa | >200 | >170 |
Water sorption in μg/mm 3 | <36; <34 | <26 |
Water solubility in μg/mm 3 | <0.08; <0.1 | <0.1 |
Vickers hardness HV 0.5/30 in MPa | >240 | >190 |
Fatigue is known as the behavior of a material to fail at stresses which are lower than their maximum monotonic loading stress after a certain number of cycles. A common visualization for fatigue behavior involves S–N-curves, which present the number of cycles to failure versus the stress (or load) under cyclic conditions. Depending on the kind of application and the material tested there are different definitions. According to Läpple typical definitions within mechanical engineering include: (1) low-cycle fatigue (resisting less than 10 3 cycles); (2) finite-life fatigue strength (resisting between 10 3 cycles and 10 6 –10 7 cycles) and (3) fatigue limit (resisting more than 10 7 cycles) . In Young et al. the fatigue limit is described as an assumption that “stresses below this limit will not cause failure regardless of the number of repetitions” .
No standard protocol could be found for the experimental testing of the fracture and fatigue strength of denture teeth, specifically for bulk fracture. However, due to the equivalent environmental factors and loads some parts of the ISO 14801:2007 were used to provide guiding principles for the experimental test design; for example the load-frequency and wave form, loading at clinically-relevant angle and characteristics of the testing machine. In addition, both ISO 14801:2007 and the method developed are designed to cause bulk failure under fatigue conditions. The null hypotheses being tested are: (1) accelerated fatigue testing cannot be applied to examine the bulk fracture of denture teeth and (2) material improvements will not increase the predicted lifetime of denture teeth.
2
Methods
2.1
Experimental setup
Cyclic loading (sinusoidal) was performed with a hydraulic universal testing machine (858 Mini Bionix II, MTS Systems Corp., Eden Prairie, MN, USA). Fatigue tests were run at 5 Hz which is within the 2–15 Hz specified under ISO 14801:2007. Initially, the teeth were mounted in a fixture which allowed loading at an angle of 30° with a cylindrical piston (glass-fiber/epoxy, NEMA Grade G10) having a diameter of 10 mm. Preliminary tests using this fixture resulted in a fracture behavior which differs from the clinical failure. Instead of a complete fracture as observed for example in Fig. 1 , only the lower labial side of the tooth fractured.
This fact required the design of a second fixture which allowed loading at 90° (perpendicular to the tooth). It consisted of two parts, with the main part (part 1) mounted on the adapter-plate of the testing machine and the second part (part 2) used for mounting the specimen. In both fixtures, the embedded specimen can be placed and mounted by tight fit. The details of the test setup are shown in Fig. 2 . Elongated holes enabled step-less adjustment possibilities within the adapter-plate perpendicular to the direction of the movement of the piston. The incisal edges of the teeth were protected from contact damage fracture by first lubricating the contact point and then curing photo-polymerizing resin between the loading piston and the tooth. This assured low contact stresses between the piston and the tooth resulting in consistent bulk fracture. Subsequent fracture surface analysis was performed by light-microscopy and SEM to check and confirm the assumed fracture mode. The complete procedure can be seen in Fig. 3 .


As mentioned above, the first specimens examined within this work were the commercially available denture teeth of the type SR Phonares NHC ( Table 1 ). These are nanohybrid teeth consisting of four different layers; two layers of PMMA and two layers of nano-hybrid-composite (NHC). NHC is composed of a urethane dimethacrylate (UDMA) matrix, PMMA and “highly crosslinked inorganically filled macrofillers, highly densified inorganic microfillers and silanized nanoscale fillers based on silicon dioxide” .
Two other denture teeth used were based on material improvements designed to increase the toughness of the teeth while keeping the same external design and layering approach equal to the SR Phonares NHC. Material properties of the three teeth appear in Table 1 . Within this paper the material SR NHC Phonares is called P1 (Phonares 1), the second material IM1 (improved material 1) and the third material IM2 (improved material 2). Artificial teeth of the anterior maxilla (teeth numbers 6–11) were provided for this project by Ivoclar Vivadent. Work was focused on upper central incisors, and since pilot testing demonstrated no differences in fatigue behavior between left and right central incisors these were uniformly mixed in all three groups.
Bases of each tooth were embedded in cold-curing acrylic (Lucitone199 Repair Material Powder, Lucitone Fas-Por Liquid, Dentsply, USA) without the use of additional containment material. Two critical details needed to be controlled: (1) the angle of the embedded tooth; and (2) the embedment depth. To accomplish this, two-piece casting molds were made using silicone putty (Virtual Refill Putty Fast Set, Ivoclar Vivadent, Liechtenstein). Initially, the tooth to be mounted was put in a putty-filled pipe section with a diameter of 25 mm and a height of 20 mm using a dental survey instrument to align the tooth in the correct axis. After setting of the putty, the mold was cut at the embedding height (2.5 mm apical). For the second part of the mold, a cylinder (diameter of 9.5 mm and a height of 12 mm) was placed on the first mold, aligned using a dental survey instrument. Subsequently putty was filled around it, again using the pipe section as an outer border. Finally, the outer part of the second mold part was cut to allow a filling opening for the acrylic resin. The different parts of the mold and an embedded specimen are shown in Fig. 4 . An additional advantage of this method is the better reproducibility of the embedding procedure due to the molds. To reduce air bubbles and to enhance the curing process the tooth and acrylic filled molds were placed in a water-filled pressure pot with a temperature of 60 °C and a pressure of 0.76 MPa for duration of 30 min. Due to shrinking of the embedment material while processing a hollow occurred at the side opposed to the tooth, which was filled afterwards with cold-curing acrylic. This surface was subsequently ground to achieve a flat surface.

For the test design, the following procedure was followed: at first, the fracture load of the teeth made of material P1 was determined within quasi-static strength tests (actuator speed = 0.5 mm/min). First load-level for fatigue testing was set at approximately 60% of the average monotonic fracture load, this equals to the average monotonic fracture load minus 2 SD (approximated) allowing a high probability to achieve repeated load cycles until fracture. An additional load level was chosen according to the results of the test. For both load-levels three fatigue tests were performed. Initial fatigue tests were performed at this initial load under load control within the MTS machine with a sinusoidal wave form between 20 N and the test load.
This initial data was then used to develop pilot data sets for further fatigue testing designs using test design features for accelerated testing within lifetime analysis software ALTA 7 (Reliasoft, Tucson, USA). For the fatigue-tests with the improved materials the same load-levels were chosen to simplify the comparison of the different materials.
For pilot data, since the average fracture load of the material P1 amounted to 322.0 N (SD 63.3 N), the first load level for fatigue testing was set at 200 N. Due to the low number of cycles to failure at this load (average = 6553 cycles), the second load-level was set at 150 N. Three specimens were run for each load level. This pilot data set was used within the accelerated testing design feature of ALTA7 for a “3 Level Best Compromise Plan”. For this particular data example further loads of 175 N (3 specimen) and 135 N (8 specimen) were chosen. The complete list of the different load-levels and the according number of tested specimens for the tests of the different materials can be seen in Table 2 .
Number of specimens | |||
---|---|---|---|
Load in N | P1 | IM1 | IM2 |
100 | 1 | – | – |
135 | 8 | 4 | 4 |
150 | 4 | 4 | 4 |
175 | 3 | 3 | 4 |
200 | 4 | 3 | 4 |
2.2
Estimated clinical use load
To get an estimate of the clinical use load, it was necessary to (1) estimate the annual number of chewing cycles and (2) using this information to project a clinical load range. According to observations at the student clinic, the graduate prosthodontic clinic and the Center for Implant and Restorative Dentistry of the University of Connecticut Health Center the annual probability of failure (Pf) is about 2.5% (predominantly Ivoclar Phonares teeth) . In Fig. 5 the probability of failure versus the use load for P1 and 500,000 cycles, 1,000,000 cycles and 1,500,000 cycles is shown (calculated within ALTA 7). These values seem to be a reasonable range for an annual amount of chewing cycles variously reported as being between 200,000 and 250,000 and 657,000 for chewing alone (swallowing seems not to have been measured or estimated but would easily double the cycles per year) . From this graph a use load between about 63 N (1,500,000 cycles, 2.5% Pf) and about 80 N (500,000 cycles, 2.5% Pf) could be estimated. This is surprisingly consistent with the result of Fontijn-Tekamp et al. . For estimation purposes, an annual amount of 1,000,000 cycles and a use load of 70 N were assumed (note from Fig. 5 that the estimated use load is not extremely sensitive to assumptions regarding annual chewing cycle numbers).
2.3
Statistical methods
Lifetime analysis and lifetime predictions were done using ALTA 7 assuming a general log-linear failure model (lifetime versus load) and lognormal probability distribution functions (Pdf’s) for describing cycles to failure at any given load. When the Pdf’s do not differ at each load used, ALTA 7 calculates a combined general-log linear failure model/lognormal distribution function allowing lifetime predictions from accelerated test conditions to use (or service) conditions, also regarding different assumed probabilities of failure (Pfs). Another advantage of ALTA 7 compared to “normal statistics” is that teeth which did not fracture after a certain amount of cycles (survivors) are weighted different than fractured (failed) teeth; so “runouts” were also included in the data analysis.
2
Methods
2.1
Experimental setup
Cyclic loading (sinusoidal) was performed with a hydraulic universal testing machine (858 Mini Bionix II, MTS Systems Corp., Eden Prairie, MN, USA). Fatigue tests were run at 5 Hz which is within the 2–15 Hz specified under ISO 14801:2007. Initially, the teeth were mounted in a fixture which allowed loading at an angle of 30° with a cylindrical piston (glass-fiber/epoxy, NEMA Grade G10) having a diameter of 10 mm. Preliminary tests using this fixture resulted in a fracture behavior which differs from the clinical failure. Instead of a complete fracture as observed for example in Fig. 1 , only the lower labial side of the tooth fractured.
This fact required the design of a second fixture which allowed loading at 90° (perpendicular to the tooth). It consisted of two parts, with the main part (part 1) mounted on the adapter-plate of the testing machine and the second part (part 2) used for mounting the specimen. In both fixtures, the embedded specimen can be placed and mounted by tight fit. The details of the test setup are shown in Fig. 2 . Elongated holes enabled step-less adjustment possibilities within the adapter-plate perpendicular to the direction of the movement of the piston. The incisal edges of the teeth were protected from contact damage fracture by first lubricating the contact point and then curing photo-polymerizing resin between the loading piston and the tooth. This assured low contact stresses between the piston and the tooth resulting in consistent bulk fracture. Subsequent fracture surface analysis was performed by light-microscopy and SEM to check and confirm the assumed fracture mode. The complete procedure can be seen in Fig. 3 .
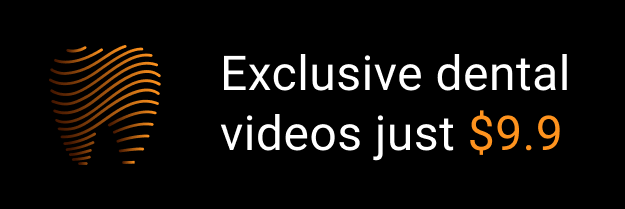