Introduction
This in-vitro study presents the development and validation of an artificial tooth-periodontal ligament-bone complex (ATPBC) and comparison of its behavior with that of rigid dowels during third-order torque simulation.
Methods
ATPBCs were coupled using a 1:1 mixture of room-temperature vulcanization silicone and gasket sealant to act as a periodontal ligament simulant (PDLS). PDLS thicknesses ranging from 0.2 to 0.7 mm, in increments of 0.1 mm (n = 5 for each thickness), were tested using a linear crown displacement procedure. A suitable PDLS thickness was selected for use in third-order torque simulations to compare ATPBC (n = 29) and rigid (n = 24) dowel behavior. Their results were compared for archwire rotations up to 20° for both loading and unloading curves with repeated-measures analysis of variance.
Results
When used in third-order torque simulations, the ATPBC dowels with a 0.5-mm PDLS thickness showed a statistically significant difference from rigid dowels ( P = 0.020), with a 95% confidence interval (0.254, 2.897 N·mm) and a mean difference of 1.575 N·mm.
Conclusions
Inclusion of a PDLS in an ATPBC resulted in a statistical difference when compared with rigid dowels; however, the region where behavior differed was at low angles of archwire rotation, and the resultant torque was arguably outside a clinically relevant range.
Highlights
- •
An artificial tooth-periodontal ligament (PDL)-bone complex (ATPBC) was developed.
- •
Crown displacement tests were used to evaluate ATPBC PDL simulant (PDLS) thicknesses.
- •
An in-vitro orthodontic torque simulator (OTS) was used to simulate third-order torque.
- •
ATPBC and rigid dowel responses when implemented in the OTS were compared.
- •
Results indicate that inclusion of PDLS compliance in the OTS is unnecessary.
In-vitro experimental procedures continue to be used extensively in the literature to study force and moment systems generated by orthodontic appliances. Their attractiveness spawns from their relatively low cost compared with studies involving living specimens, the lack of necessity for ethical approval, and the availability of equipment. Additionally, these procedures allow for control of variables that cannot be regulated in typical patient studies and measurement of data (eg, orthodontic loads) that are not easy, or are impossible, to obtain in clinical trials. Despite their popularity, they come under scrutiny as unable to properly simulate the oral environment. This is especially true of the biologic effects—eg, tissue remodeling—that result from orthodontic treatment. Although it might not be possible to replicate all factors of an in-vivo experiment, some can be incorporated into an in-vitro study, increasing its applicability to the oral environment, and should be investigated further.
A particular limitation of many dental-related in-vitro studies is the lack of periodontal ligament (PDL) compliance when considering force-displacement or torque-angle relationships generated by orthodontic appliances. It is a common assertion that inclusion of this compliance would greatly impact the loads measured or predicted through in-vitro research. Specifically, forces resulting from a prescribed displacement would be lower than predicted, and displacements for a given force would be larger. Although this trend is certainly expected, the magnitude at which added compliance would affect in-vitro results has yet to be investigated in detail.
Xia and Chen considered the development of an artificial tooth-PDL-bone complex (ATPBC) through the integration of a PDL simulant (PDLS). A 1:1 mixture of room-temperature vulcanization silicone and gasket sealant incorporated in an ATPBC showed good agreement with the human crown displacement data from Christiansen and Burstone. The creep, stress relaxation, and hysteresis behavior of the ATPBC developed by Xia and Chen also agreed well with results from the literature. This particular PDLS, used in conjunction with an ATPBC, shows promise for including PDL compliance in orthodontic in-vitro research.
The in-vitro study of third-order torque through archwire rotation has been extensive in the literature. As archwire and bracket dimensions and materials change, it will be imperative to continue this line of research to understand how such changes impact torque expression and thus overall treatment mechanics. To date, no study has included PDL compliance with an ATPBC or otherwise. Understanding how this compliance influences the results, if at all, is imperative for future research on third-order rotational mechanics.
There were 2 main objectives in this study. The first was the development and validation of an ATPBC that accurately replicates the true biologic compliance illustrated in the literature. Second, the ATPBC was used in an orthodontic torque simulator (OTS) to obtain torque-angle data during the third-order rotation of an archwire relative to a bracket. Rigid dowel specimens were also used, allowing for the comparison of data with and without PDLS compliance. In doing so, we investigated the magnitude to which compliant behavior influences third-order torque expression during in-vitro testing.
Material and methods
The initial portion of the study focused on developing an ATPBC for the maxillary central incisor. This process was briefly detailed in a previous study but will be considered again for completeness. The ATPBCs were manufactured using 1 section of aluminum representing the tooth and another piece used as a socket, as illustrated in Figure 1 . These components were coupled through a 1:1 mixture of room-temperature vulcanization silicone (587 Blue RTV Silicone; Loctite, Westlake, Ohio) and gasket sealant (number 2; Loctite) and allowed to cure for 72 hours before testing. The choices of root shape and gasket were based on the methodology used by Xia and Chen, since they obtained ATPBC behavior representing data from the literature. Additionally, the use of a purely conical shape for the root geometry imitated the general root shape of the maxillary central incisor reasonably well and greatly simplified manufacturing time and cost.
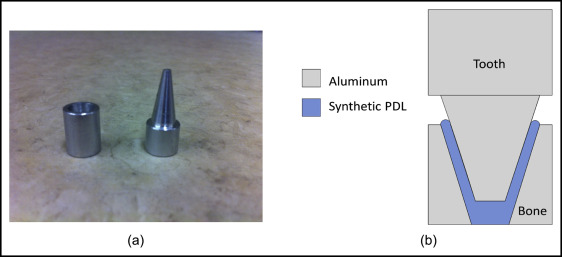
Thickness of the PDL was controlled by fixing the height of the tooth relative to the socket as the samples were coupled with the PDLS mixture. Concentricity of the tooth relative to the socket was controlled by assembling the ATPBC in a drill bushing. Because PDL thickness is based on a variety of factors (eg, location on the tooth, functionality), a range from 0.2 to 0.7 mm in 0.1-mm increments was tested to determine which could most accurately represent the true complex response. The PDL thickness was measured in the horizontal plane and was calculated based on the relative height of the tooth and the taper angle of the tooth. A load cell (Nano17; ATI Industrial Automation, Apex, NC) was set facing a micrometer (PI M-230.10; Physik Instrumente (PI) LP, Auburn, Mass), allowing for force to be measured while a linear displacement was applied, as depicted in Figure 2 . The contact was applied to the tooth adaptor at a distance of 10.3 mm from the top edge of the socket piece. This distance was selected to maintain consistency with the location used by Christiansen and Burstone, allowing for comparison of the data. The displacement was applied quasi-statically at a velocity of 0.01 μm per second to a maximum displacement of 0.2 mm. A total of 5 specimens were tested at each PDLS thickness, and the results were compared with previous in-vivo studies by Christiansen and Burstone and Wei et al, as well as the similar ATPBC study conducted by Xia and Chen. To the best of our knowledge, this is the first time that both the displacement/loading control and data acquisition have been fully automated.
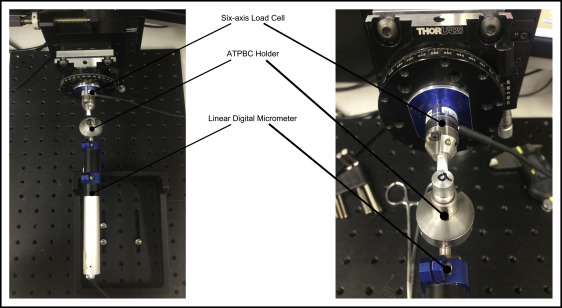
In the second portion of this study, an OTS was used to investigate differences between compliant and rigid connections during in-vitro torque simulations. Thirty control specimens with solid stainless steel dowels and 30 aluminum ATPBC samples were tested. The control dowels had a nominal diameter of 10 mm and were 24 mm in height. The ATBPC samples had an expected height of 25.3 mm, PDLS thickness of 0.5 mm, and a nominal diameter of 10 mm. As will be discussed later, a 0.5-mm PDLS thickness was chosen because it resulted in the best fit to the experimental data during simulated crown linear displacement tests. Adhesive (E-60HP Hysol Epoxy; Loctite) was used to attach Damon Q (Ormco, Orange, Calif) self-ligating brackets to the surface of each sample as shown in Figure 3 . The brackets were placed 4.5 mm from the top edge of the ATBPC samples and 3.5 mm from the top of the controls to obtain a consistent distance between the bracket and the load-cell adapter. The location of the brackets was controlled using an orthodontic positioning gauge.
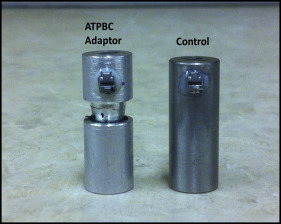
The samples were randomly tested using a 0.019 × 0.025-in stainless steel wire in the OTS, with a new section of wire used for each test. The archwire and the bracket were aligned relative to each other so that all forces and moments were negligible before each test. The wire was rotated from the neutral position, taken as 0°, up to 30°, and then unloaded back to the neutral position. All forces and moments were recorded from the load cell at 1° increments during the loading and unloading stages. Load cell readings were recorded and averaged over 100 data points at each experimental angle. The third-order torque was transformed from the load cell to the location of the bracket using the following relationship.
T x ‘ = T x cos ( θ ) − F z [ Δ y cos ( θ ) − Δ x sin ( θ ) ] + T y sin ( θ ) + F y Δ z [ cos ( θ ) − sin ( θ ) ]
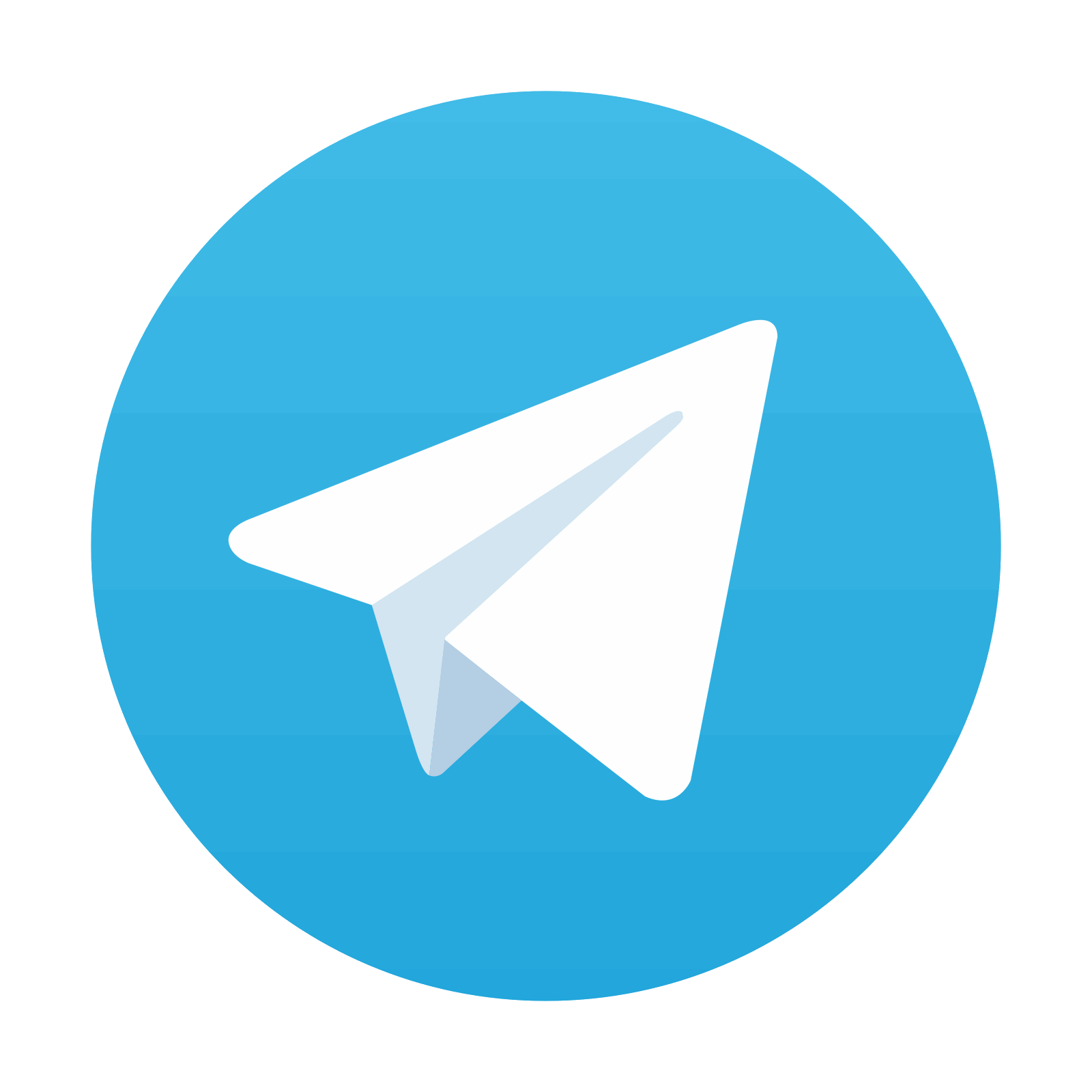
Stay updated, free dental videos. Join our Telegram channel

VIDEdental - Online dental courses
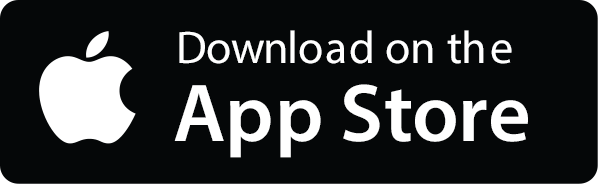
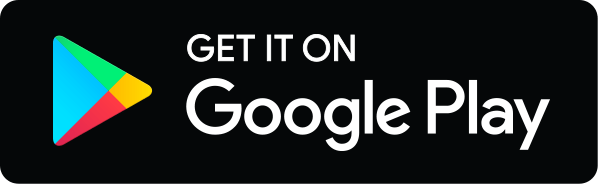