Introduction
Cone-beam computed tomography (CBCT) might be more accurate in identifying radicular surface lesions compared with digital periapical radiography. In this study, we compared these techniques in detecting simulated root resorption lesions.
Methods
A porcine mandible was used to support 10 human maxillary central incisors. CBCT and digital periapical radiographic images were generated before and after the introduction of standardized and sequentially larger root defects on either the mesial or the lingual root surfaces. The images were randomly labeled and evaluated by 3 examiners. Each image was classified according to defect size (0, none; 1, mild; 2, moderate; 3, severe).
Results
Interrater reliability was acceptable (0.856 ≤ P ≤0.981). The location of the root defect (mesial vs lingual) had no significant effect on the evaluation of defect size. Both periapical radiographs and CBCT were slightly better at detecting lingual defects than mesial defects (75% vs 65% and 65% vs 60%, respectively), but these effects were not statistically significant ( P = 0.49 and P = 0.74, respectively). The mean percentages of correctly diagnosed defect sizes were 65% for CBCT and 75% for periapical radiographs. Examiners using CBCT images tended to overestimate defect sizes (κ = 0.481) and correctly categorized teeth with no, mild-moderate, and severe defects 80%, 45%, and 90% of the time, respectively. Examiners using periapical radiographs tended to underestimate defect sizes (κ = 0.636) and categorized teeth with no, mild-moderate, and severe defects 100%, 50%, and 100% of the time, respectively.
Conclusions
There was no difference in accuracy of identifying defects between periapical radiographs and CBCT images.
External root resorption during orthodontic tooth movement is thought to be caused by ischemic necrosis of the periodontal ligament, with initial damage to the root cementum layer. Various biologic and mechanical risk factors have been associated with an increased risk of root resorption during orthodontic treatment. For mechanical factors, extensive tooth movement, root intrusion and torque, orthodontic force magnitude, and duration have been implicated. With respect to biologic factors, genetic predisposition to hyperinflammation, systemic disease, sex, and medications are associated with root resorption.
The orthodontic literature contains reports of clinical and laboratory investigations of root resorption. The incidence of reported root resorption during orthodontic treatment varies widely among investigators. Tooth structure, alveolar bone structure at various locations, and types of movement might explain these variations.
Root resorption of the deciduous dentition is a normal, essential, and physiologic process. Permanent teeth can also undergo significant external root resorption; however, this is not considered normal, essential, or physiologic. In 1 study, root resorption of permanent teeth was found to begin in the early leveling stages of orthodontic treatment. About 4.1% of patients studied had an average resorption of 1.5 mm or more of the 4 maxillary incisors, and about 15.5% had 1 or more maxillary incisors with resorption of 2.0 mm or more from 3 to 9 months after initiation of fixed appliance therapy. In a separate study, it was found that mean apical root resorption in permanent teeth was strongly correlated with total apical displacement of the root (R = 0.822) and treatment duration (R = 0.852). The maxillary anterior teeth were found to be the most resorbed, with 25% undergoing greater than 2 mm of root resorption. The posterior teeth were relatively unaffected.
During orthodontic therapy, early detection and correct characterization of the location and the amount of resorption of the root surface are necessary to prevent further destruction of the tooth root. External resorption is often first detected radiographically via analog or digital 2-dimensional (2D) images. Studies have shown that resorption defects are harder to detect on buccal than proximal root surfaces. Resorption defects of 0.6-mm diameter and 0.3-mm depth were hard to detect. Analog and digital 2D imaging modalities yielded false negative results in about 51.9% of cases and false positive results in about 15.3%. However, in 2 separate studies, digital radiographic methods were found to be superior in detecting simulated external root resorption lesions compared with analog radiographs.
The diagnostic accuracy of root lesions based on a single image can be improved from 73% to 87% when images are available from different angles. However, intraoral radiographs do not indicate the true dimensions of lesions. The amount of information gained from 2D periapical radiographs is incomplete, since the 3-dimensional (3D) anatomy is compressed into a 2D image. The compression causes “noise” that might result in underestimation of the size of the resorption lesion. Hence, 3D imaging techniques have the potential to offer accurate quantification of root resorption defects and, perhaps, earlier detection of lesions. Conventional computed tomography (CT) has been shown to have high sensitivity and specificity in the detection of external root resorption defects in vitro. High cost and radiation exposure make this modality generally unsuitable for routine imaging of the dentition. Cone-beam CT (CBCT) is an imaging modality that offers the advantages of 3D voxels and high diagnostic yield with short scanning times and significantly lower radiation dosages than conventional CT. In 2 ex-vivo studies evaluating CBCT for measurement of resorption defects, the influence of voxel size was assessed. CBCT was found to be an accurate method for the imaging of simulated external root resorption. A 0.3 mm 3 voxel appeared to produce the best results. In an in-vivo investigation of detection and management of root resorption lesions with intraoral radiography and CBCT, CBCT was found to be effective and reliable in detecting resorption lesions. Since this modality might be useful for enhancing diagnosis of early root resorption, there is a need to compare the diagnostic accuracy of CBCT with digital periapical radiographs for assessing root defects. Therefore, this study was designed to evaluate the accuracy of CBCT imaging vs intraoral periapical digital radiography for the identification of simulated root resorption lesions. We compared observers’ abilities to detect simulated external root resorption defects using the 2 diagnostic imaging modalities: digital periapical 2D radiographs produced by a radiation beam directed horizontally to the proximal tooth surface from a direct digital system vs reconstructed images from 3D data sets generated from a CBCT system. The main objective was to determine whether the ability to detect defects with these 2 modalities was influenced by location and amount of resorption.
Material and methods
A total of 40 specimens were created for imaging via 2 modalities by securing maxillary incisors in a bony housing in a standardized fashion. A porcine mandible was obtained from a local abattoir and handled according to institutional policy. The ramus of the mandible was sectioned distally to the last molar, and the anterior portion was used to create a simulated root socket to house a sample tooth root for imaging. Ten maxillary incisors extracted for various periodontal conditions were obtained. Approval to use extracted teeth for the study was given by the institutional review board of the University of Missouri at Kansas City. Selection criteria for the sample teeth were atraumatic removal, no restorations, no previous root resorption, no previous endodontic therapy, and no periapical pathosis.
Initially, each intact incisor root (category 0, no defect) was secured in the simulated socket to create a specimen, imaged by 2 methods, and then removed from the socket. Subsequently, each root was then subjected to 3 sequentially increasing sizes of defects (categories 1, mild defect; 2, moderate defect; and 3, severe defect). After each standardized defect was developed, it was quantified, and the root was secured again in the same position and orientation in the simulated socket. The specimen was imaged via the 2 methods, and then the specimen was disassembled. Details of these methods are described in the following paragraphs.
The simulated root socket was created by using a pineapple-shaped bur (H79E040, Komet, Rock Hill, SC; diameter, 6.8 mm; depth, 13.8 mm) on a slow-speed hand piece to create a hole 10 mm in depth with a uniform circumference of 2 mm of cortical bone. Three orientation marks were made with a high-speed hand piece and a number 57 dental bur sunk to the depth of the cutting surface next to the simulated socket on the posterior, anterior, and medial surfaces of the mandible. Each tooth was placed in the simulated socket and centered by using cotton pellets. Then an external coordinate system was fabricated from impression putty (Reprosil Vinyl Polysiloxane Impression Material, 1075 mL tube; Dentsply Caulk, Milford, Del) molded around the tooth and into the orientation marks. Once set, the putty was removed and cut lengthwise just lateral to the posterior and anterior orientation marks and down the midline of the negative tooth impression ( Fig 1 ). Thus, when a tooth was replaced in the socket for subsequent CBCT and radiographic imaging, the external coordinate system was used to ensure that it was replaced in the exact same orientation and that a positive seat was formed between the tooth, the external coordinate system, and the orientation marks. To assemble a specimen, cotton pellets were removed, and mounting plaster (Pemaco Lab Stone, St. Louis, Mo; ratio, 30 cm 3 of water to 100 g of powder) was mixed to a runny consistency and vibrated into the simulated socket. The tooth was placed in the bony housing by using the external coordinate system ( Fig 1 ) and was imaged.
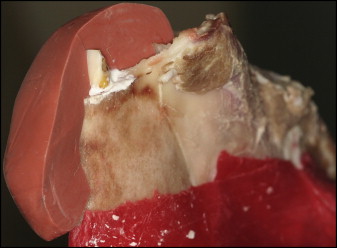
Periapical radiographs of the specimens were obtained by using a charge-coupled device connected to an x-ray machine (RVG model FV-47, Trophy Radiology, Marietta, Ga) that provided 70 kVp and 8 mA. Exposure time was 0.02 impulses, and the x-ray beam was directed at a right angle to the proximal tooth surface. CBCT images of the specimens were obtained with a CBCT machine (Classic i-CAT model, Imaging Sciences International, Hatfield, Pa) at 120 kVp, 26 mA, and 0.25-mm voxel size.
After initial imaging ( Fig 2 ), each tooth was removed from the socket and, as previously outlined, 3 standardized and sequentially larger defects were created on either the mesial or the lingual root surface, 5 mm coronal to the apex. Odd-numbered teeth (1, 3, 5, 7, and 9) received defects on the mesial surface, and even-numbered teeth (2, 4, 6, 8, and 10) received defects on the lingual surface. Lines were drawn with a permanent marker on both sides of each tooth from incisal edge to apex to divide in half the 5 odd-numbered teeth buccolingually and the 5 even-numbered teeth mesiodistally. A digital gauge was used to measure 5 mm coronal to the apex along the previously drawn line, where an ink mark was made on the appropriate surface. At the mark, a high-speed hand piece with a number ½ round dental bur was used to create the initial (mild) defect. The shaft of the bur was perpendicular to the long axis of the tooth, and the bur penetrated the root surface to the depth of the cutting surface. Similarly, moderate and severe defects were made by using number 2 and 4 round burs, respectively. All defects were made by the same investigator (L.G.) using magnified vision, and the defect depths were verified by another investigator (A.B.) also using magnified vision.
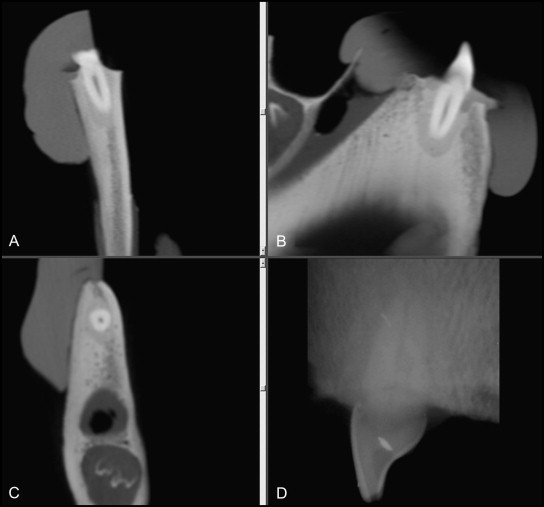
After each defect was created, an impression was made of it with polyvinyl siloxane light-body impression material (Aquasil, Dentsply Caulk), and then the defect was filled with base plate wax to prevent the mounting plaster from flowing into the defect. The diameter of each impression was quantified by using a measuring microscope (Luxo Magnifier, Coe-Bilt Laboratories, Chicago, Ill; 118 V, 22 W, 60 cycles) for calculation of the volume of the defect. The 10 CBCT and 10 periapical radiographic images of root defects in the 4 categories (0, no defect [ Fig 2 ]; 1, mild defect [ Fig 3 ]; 2, moderate defect [ Fig 4 ]; and 3, severe defect [ Fig 5 ]) were randomly labeled and viewed by 3 blinded examiners, of whom 1 was a board-certified oral radiologist and 2 were board-certified orthodontists. The examiners were allowed to adjust the gray scale and the magnification of the images and to access all CBCT plane images to arrive at a categorization of the size of root defect (0-3) represented in each image. The examiners were required to complete the assessments of all 80 images in a 40-minute time frame, thus producing a realistic clinical assessment. A repeat session of the image categorization was performed approximately 1 month later to test for intra-assessor and interassessor reliability (Spearman rho statistic). For statistical comparison of the diagnostic accuracy of the defects, the examiners’ ratings for defect sizes were collapsed separately to produce 1 composite score for the periapical and the CBCT images. If either 2 or 3 examiners agreed on the size of the defect, that categorical ranking was used as the composite score. This ranking was then compared with the known gold standard and determined to be either accurate (1) or inaccurate (0). If the 3 examiners all categorized the defects differently for an image, it was determined to be inaccurate. The McNemar change test was used to compare the accuracy of identifying defects with CBCT images and periapical radiographs. The direction of misclassification was also explored between the 2 modalities descriptively. Additionally, the effect of location of the defect (mesial vs lingual) was compared to determine whether accuracy was related to location for CBCT and periapical radiographs separately by using chi-square analysis.
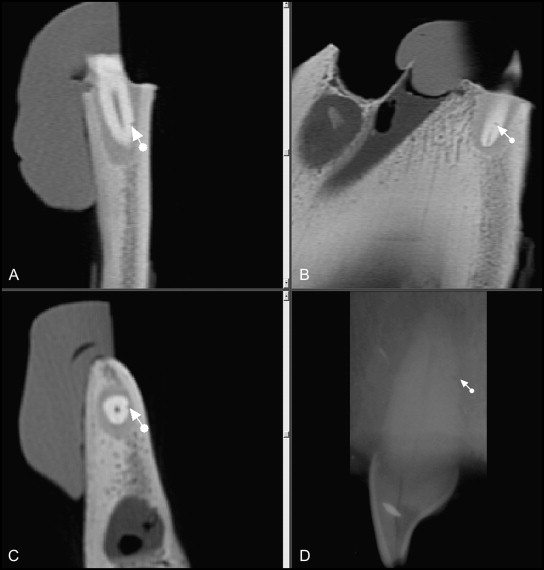
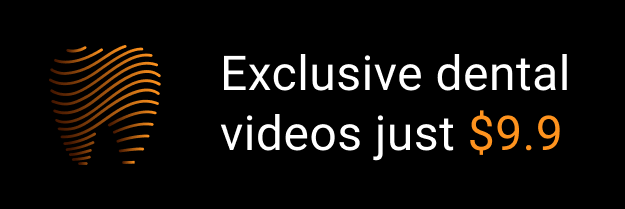