Abstract
Objective
The dentin–enamel junction (DEJ) plays a crucial role in dental biomechanics; however, little is known about its structure and mechanical behavior. Nevertheless, natural teeth are a necessary model for prosthetic crowns. The mechanical behavior of the natural DEJ and the dentin ceramic junction (DCJ) manufactured with a CAD-CAM system are compared.
Methods
The reference samples undergo no modification, while the experimental samples were drilled to receive a cemented feldspathic ceramic crown. Longitudinally cut samples were used to achieve a planar object observation and to look “inside” the tooth. A complete apparatus enabling the study of the compressive mechanical behavior of the involved tooth by a non-contact laser speckle interferometry (SI) was developed to allow nanometric displacements to be tracked during the compression test.
Results
It is observed that the DEJ acted as a critical zone accommodating the movement between dentin and enamel. A smooth transition occurs between dentin and enamel. In the modeled prosthetic, the same kind of accommodation effects also occurs, but with a steeper transition slope between dentin and ceramic.
Significance
On the natural tooth, the stress accommodation arises from a differential behavior between enamel and dentin from the DEJ. In the ceramic crown, the cemented dentin–ceramic junction should play this role. This study demonstrates the possible realization of prosthetic crown reconstructions approaching biomechanical behaviors.
1
Introduction
The dentin–enamel junction (DEJ) in teeth is the zone between two distinct calcified tissues with very different biomechanical properties: enamel and dentin . Enamel is hard and brittle and envelops the softer dentin. The enamel and dentin work together during the many load cycles experienced by the tooth over its lifetime. Generally, interfaces between materials with dissimilar elastic and mechanical properties represent “weak links” in a structure; however, the DEJ acts to successfully transfer applied loads (e.g., masticatory or impact) from the enamel to the dentin and inhibits enamel cracks from propagating into the dentin and causing tooth fracture .
The DEJ appears as a discrete line when visualized microscopically and is thought to represent the original position of the basement membrane of the ameloblasts and odontoblasts where they coincide in the embryological tooth bud . In human enamel and dentin, fatigue damage is the end result of extreme loads and is frequently associated with pathology or extensive wear. The fracture-resistant properties of the DEJ are believed to originate from a gradual change in microstructure and in the properties of dentin and enamel rather than from the abrupt transition between the two dissimilar materials . Wang and Weiner suggested that the DEJ is one of the working sites of the tooth during mastication . Imbeni et al. believe that collagen fibrils perpendicular to the interface constitute the key reinforcing mechanism at the DEJ, thus explaining why so few cracking events cause delamination when they impinge on the DEJ. Zaslansky et al. highlighted the importance of the DEJ as the binding interface between enamel and dentin. They have shown that adjacent to the DEJ is a 200–300 mm-thick zone of dentin of a much lower stiffness (compression elastic modulus) than the bulk of the dentin in the tooth.
Restorations that are all ceramic require proper adhesive bonding on the dentin to achieve their required life expectancy. All-ceramic restorations are made with feldspathic or zirconia ceramics. The strongest ceramics have a fracture toughness of at least 3.0 MPa m 1/2 , which is relatively close to the enamel fracture toughness of 1.3 MPa m 1/2 , in a direction perpendicular to the enamel rods . Nevertheless, fractures of the ceramic part of all-ceramic crowns are difficult to prevent, and crack growth is a significant problem . This phenomenon can be explained by the absence of a stress accommodation zone. The natural stress accommodation zone of 200–300 μm-thick dentin has a much lower stiffness than the bulk of the dentin core .
Bonding agents must be selected very carefully because they determine not only the adhesion but also the ultimate strength of full-ceramic crowns ; therefore, it is important to compare the mechanical behavior of natural teeth and of the all-ceramic crown cemented on dentin. Instead of “cement joint”, we will use the term “dentin–ceramic junction” (DCJ).
We applied compressive forces representative of those occurring in the oral cavity on natural teeth and all-ceramic crowns, and we determine the relative movement of enamel and dentin, or ceramic crown and dentin, respectively.
2
Materials and methods
2.1
Natural teeth
Intact lower first premolars free of caries were stored in physiological serum after having been extracted as part of the routine orthodontic treatment of young healthy adolescent patients (aged < 18). Five sets of two samples each (one natural tooth and one prosthetic tooth) were amassed. Right and left premolars from the same patient were used. One was kept intact, and the other was prepared to receive the prosthetic crown.
2.2
Prosthetic crowns
We employed the Cerec 3D ® (Sirona Dental System ® , Bensheim, Germany) CAD/CAM (computer aided design/computer aided manufacturing) unit to manufacture each prosthetic crown as a clone of the opposite tooth using the reproduction capability of the Cerec ® software V2.80. This CAD/CAM system is composed of two distinct units: the optical imprint recording also allowing the CAD, and the milling unit using the CAD data to manufacture the sample out of a ceramic block. The software was set to give a dento-prosthetic spacing of 100 μm and a peripheral joint of 40 μm for a total thickness of 800 μm. Optical imprints, of the prepared tooth and of the opposite tooth, were recorded, and the Cerec MC ® machine milled the prosthetic crowns . The whole cloning process is presented in Fig. 1 .
The Vita MarkII ® (Vita Zahnfabrik, Bad Säckingen, Switzerland) ceramic blocks of albite-enriched feldspathic ceramic were used. Their abrasion coefficient is close to that of natural dental enamel. After milling, the extrados were glazed (Azkent ® , Vita Zahnfabrik, Bad Säckingen, Switzerland).
The crowns were cemented onto the prepared teeth using Relyx Unicem ® adhesive cement (3MESPE Dental Division, St. Paul, MN, USA) following the standard clinical protocol of illumination of each side of the crown for 4 s at 3000 mW/cm 2 with a Swissmaster Light ® lamp (E.M.S., Nyons, Switzerland).
2.3
Specimen preparation
After extraction, the teeth were disinfected and stored in physiological serum with traces of chloroform. The teeth were longitudinally cut in the vestibular–lingual orientation, and one of the two resulting parts was removed with a diamond disc. Longitudinal cuts have been used to allow planar observation and to appreciate the different behaviors inside the tooth of the natural DEJ and of the DCJ interfaces. The tooth was then glued into the sample holder with a layer of Araldite ® (Hunstman Advanced Materials, The Woodlands, Texas, USA). The sample holders have been cast in chromium cobalt using the imprint of a root. The mechanical stability of the specimen holders was validated by a speckle interferometry (SI) experiment.
2.4
Loading system
The compression test device fulfills the high sensitivity of speckle interferometry and copes with the rigid body motions of the whole system. The sample tooth cemented in the sample holder was placed under the force transducer (Model 31, Honeywell International, Morriston, NJ, USA). This mid-range precision miniature load cell is slowly translated vertically by the motor (M-235 ® , PI. Karlsruhe, Germany). The system can generate a force-driven displacement (C-862 Mercury PI, Karlsruhe, Germany) or simply a user’s displacement. The compression apparatus communicates with the computer through a NI USB-6251 port (National Instruments, Austin, TX, USA) and is interfaced with an in-house LabView program. The entire mechanical system was bolted onto the holographic table top (Newport, Irvine, CA, USA). Very small displacement steps, as small as 1.6 nm, can theoretically be achieved. The force can be applied to the tooth directly with the force transducer or through a relay rod. Force has always been applied to the same part of the lingual cusp. Preliminary testing by SI showed good performances of the mechanical set-up and no spurious displacements.
2.5
SI apparatus
The optical set-up was previously presented in detail . The frequency-doubled YAG laser emits 50 mW at 532 nm wavelength in the green range. The laser beam is then injected in a COTS (commercially off-the-shelf) system (Canadian Instruments, Nottingham, UK) offering injection, variable intensity coupling in the output fibers, and phase shifting.
There are two input fibers: one for injection and one for detection of the reflected signal at the output fiber interfaces. Parts of the output fibers are bared and wrapped around piezoelectric transducers. The phase shift is applied on either or both of the two output fibers by applying a voltage at the PZT and thus generating a tiny extension of the fiber. The system is protected from thermal and mechanical effects by a plastic box and is easily breadboardable. Phase shifts were calibrated using common procedures .
A sensitive optical fiber in-plane interferometer has been designed with sensitivity vector Sv. Horizontal sensitivity is achieved. Two symmetrical beams produce an interference signal on the whole object and then illuminate the samples. The objects are optically rough-rendered by white powder. An XC70 CCD camera (Sony, Tokyo, Japan) records the sample surface under loading forces. The images are then stored in live memory or on the computer’s hard disk.
The image processing was performed using the appropriate software in LabView, and the results are presented as vector maps or false color maps. Isodisplacement maps can be visualized in real time during the loading of the sample. The system can handle areas ranging from 5 mm × 5 mm to 1 m × 1 m when using the appropriate type of laser and loading system. The measurement uncertainty is approximately 50 nm, which is common for interferometric measurement in a controlled environment. The displacement resolution is approximately 10 nm, while the spatial resolution is directly linked to the magnification of the object scene on the image sensor (1280 × 900 pixels).
A “4-buckets” phase shifting algorithm leads to phase variations during the compression test . During the test, the initial phase state was memory-resident and real-time subtracted from the current state. Sometimes the reference state was also refreshed because for some loading steps, the number of fringes can be too high, and the resulting noise would interfere with the interpretation of the resulting fringes. Interferograms show the in-plane displacements from phase shifting speckle interferometry. Highest quality images were stored on the hard disk and overlaid with upper jaw position and load value (N).
2.6
Typical experiments
Specimens were white powdered using Eutest 3 Developer Castolin Eutectic powder (Castolin, Lausanne, Switzerland) to generate a uniform diffusing surface and to avoid different modulations between dentin and enamel or ceramic and dentin. The same loads were applied to the natural tooth and the prosthetic crown samples to allow comparisons of their respective compressive behaviors.
The starting load was approximately 0 N. The compression was increased stepwise, performing discrete displacements of the transducer tip (one step is approximately 1.6 nm of Y -displacement). Therefore, a greater number of steps corresponds to a higher compression. The sample can be loaded or unloaded. The loading range applied to the different samples was between 5 N and 120 N. The CCD camera records at the sample surface the interferences of the two illumination beams coming from the two output optical fibers. Live fringes are displayed between a reference state and the current load state. Between minimal and maximal loading, different phase maps are recorded and stored in the memory.
The mechanical deformations are computed from the displacement maps generated from the phase difference maps.
Speckle interferometry is a relative displacement measurement. The maximum range between two successive measurements is approximately 20 μm. In our experiments, we need to record smaller steps as some mechanical noise appears. Therefore, new displacement references (zero displacement reset) are recorded during the test.
2.7
Displacement calculations
Different operation modes of SI are commonly used, e.g., subtraction-mode, time-averaged SI, and double-pulsed SI . In this work, we focus on subtraction-mode SI, or more specifically, on phase-shifting SI, which is mainly used for static deformation measurements.
Combining the primary interference pattern phase changes between the recordings yields new secondary interference fringes (also called correlation fringes).
The variable φ s denotes the start phase (also called speckle phase) at the initial state of the object. The variable Δ φ represents the phase change between two states.
These speckle interferograms can be subtracted and lead to the following equation for the secondary interference fringe pattern, assuming perfect spatial correlation between the two primary speckle patterns:
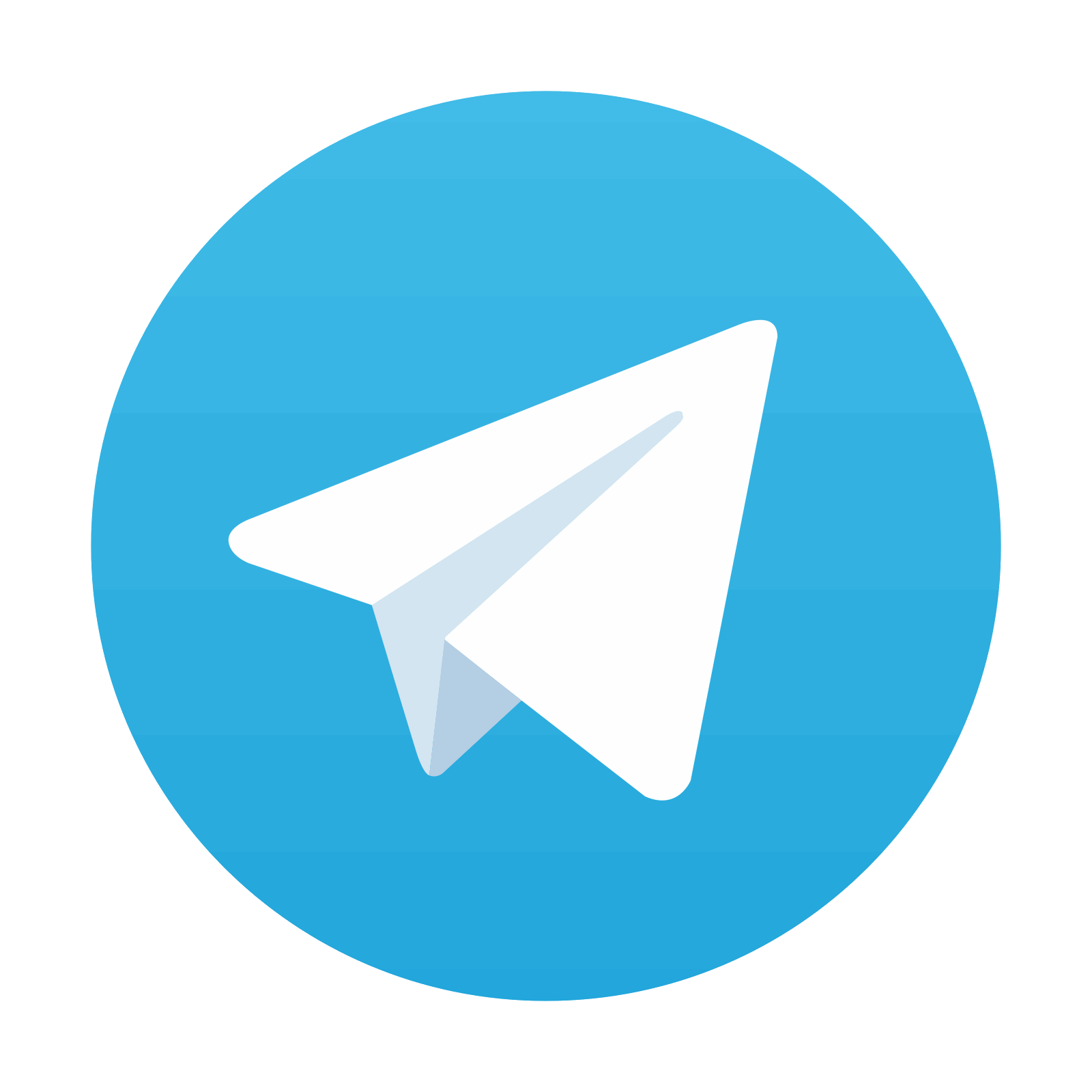
Stay updated, free dental videos. Join our Telegram channel

VIDEdental - Online dental courses
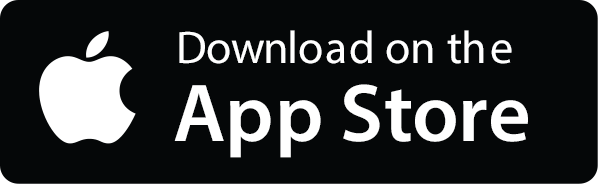
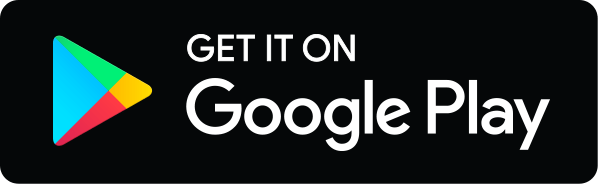