Abstract
Objective
The aim of this study was to evaluate the calcium release, pH, flow, solubility, water absorption, setting and working time of three experimental root canal sealers based on mineral trioxide aggregate (MTA) and two forms of calcium phosphates (CaP).
Methods
The materials were composed of a base and a catalyst pastes mixed in a 1:1. The base paste was made by 60% bismuth oxide and 40% butyl ethylene glycol disalicylate. Three different catalyst pastes were formulated containing 60% MTA or 40% MTA + 20% CaP (hydroxyapatite HA or dibasic calcium phosphate dehydrate DCPD), 39% Resimpol 8% and 1% titanium dioxide. MTA Fillapex was used as control. The release of calcium and hydroxyl ions, solubility and water absorption were measured on regular intervals for 28 days. The working time and flow were tested according to ISO 6876:2001 and the setting time according to ASTM C266. The data were analyzed using 1-way ANOVA with Tukey’s test ( p < .01).
Results
All the cements showed basifying activity and released calcium ions. MTA Fillapex showed the highest values of flow ( p < .01) and working/setting times ( p < .01) and the smallest values of solubility ( p < .01) and water absorption ( p < .01).
Significance
All experimental materials showed satisfactory physical–chemical properties to be used as endodontic sealers in clinical practice.
1
Introduction
One of the aims of root canal treatment is to fill the root canal system with an impervious, biocompatible and dimensionally stable sealer . The material is placed on the vital tissues and the tissue response may influence the outcome of treatment . Some materials have been used as root canal sealers as zinc oxide–eugenol, calcium hydroxide, calcium phosphate and calcium silicate cements . New root canal sealers are constantly being developed in attempts to improve the physical, chemical and biological properties.
Calcium-silicate cements, such as mineral trioxide aggregate (MTA) cements, demonstrated a wide range of dental clinical applications as root-end filling materials , root perforation repair, pulp capping, root canal sealers , and dentin hypersensitivity . MTA cements consist of a powder with fine hydrophilic calcium silicates particles and are able to set in contact with water, blood or other fluids and release calcium . MTA-based cements are bioactive and biocompatibile materials. It has been shown to induce the differentiation of osteoblasts , to improve proliferation of periodontal fibroblasts and to stimulate mineralization of dental pulp cells .
A root canal sealer must be able to set when is placed in contact with oral fluids and wet tissues to avoid the fast dissolution and removal (wash-out) from the surgical site due fluid contamination . Conventional MTA cements showed some clinical limitation when used as endodontic materials due to their poor handling and extended setting time . The composition of MTA-based cements can be properly modified to improve physicochemical properties as well as handling characteristics, to reduce the setting time and the solubility by including a resin, as previously demonstrated by Gandolfi et al. or the apatite-forming ability . It has been recently demonstrated that MTA cements doped with alpha-tricalcium phosphate support the survival and differentiation of human orofacial bone mesenchymal stem cells and that calcium phosphate have a stimulatory effect on cementoblasts .
Root canal sealers based on MTA have been studied and new endodontic sealers are now marketed as Tech Biosealer endo (Isasan, Italy) and MTA Fillapex (Angelus, Londrina, Brazil). MTA Fillapex is trade secret, but it is known that tha basic composition contains synthetic Portland cement clinkers and butyl ethylene glycol disalicylate as salicylate resin. Other endodontic sealers contain salicylate resin as Sealapex (Sybronendo, Glendora, USA). The salicylate is a chemical compound frequently used in the composition of anti-inflammatory creams and oils . Several derivatives of salicylates resins with differences in molecular structure and size of carbon chain can be used to obtain resins/polymers with different physical characteristics as rheological characteristics and handling properties .
MTA Fillapex has been recently developed (2010) in attempt to combine the biological and sealing properties of MTA cements with the physicochemical properties required by a root sealer . MTA Fillapex showed to be a biocompatible material . The manufacturer claims that MTA Fillapex has great working time and flow, excellent radiopacity and solubility and is easy to handle. A recent study showed that MTA Fillapex has physicochemical properties suitable to be used in an endodontic treatment. However, there is a lack of knowledge regarding the effects of butyl ethylene glycol disalicylate on the physical–chemical properties.
Calcium phosphates (CPC) are materials that can promote the dissolution and precipitation of calcium and phosphate ions . Some studies have demonstrated that CPC is useful in the composition of sealers used in root canal treatment . There are several types of calcium phosphates which can be classified by the molar ratio between the calcium and phosphorus atoms (Ca/P). Most of them used in vivo are calcium orthophosphate .
Hydroxyapatite (HA) is a type of calcium orthophosphate and the main mineral component of bone and it has a similarity structural and chemical with the mineral phase present in the teeth . HA is the most biocompatible and stable in aqueous solution and nowadays, the main biomaterial used in the bone tissue repair due to its biological properties. It shows apparent adhesion to dental tissue and excellent biocompatibility and osteoconductivity properties . HA may be added in the composition of root canal sealers to improve the biological properties of the materials . Synthetic HA has been introduced as main component in root canal sealers as Bioseal (Ogna Laboratori Farmaceutici, Muggiò, Italy) and Apatite Root Sealer I, II and III (Dentsply-Sankin, Tokyo, Japan).
Dibasic calcium phosphate dihydrate (DCPD) is an other type of calcium orthophosphate used as active component in root canal sealer. It has a high solubility and reach the stability by the conversion in other calcium phosphate as HA . DCPD possess good osteoconductivity . Some recent studies reported satisfactory results of sealing ability and cell response to root canal sealers containing DCPD .
The aim of this study was to investigate the physical–chemical properties of experimental MTA-based root canal sealers containing butyl ethylene glycol disalicylate with or without addition of a calcium phosphate component (HA or DCPD).
2
Materials and methods
2.1
Synthesis and characterization of butyl ethylene glycol disalicylate
The 1,3-butyl ethylene glycol disalicylate was synthesized by the transesterification reaction of methyl salicylate (Synth Laboratory, São Paulo, Brazil) in two different alcohols in a molar ratio of 1:3. The isopropoxide titanium (Sigma–Aldrich, St. Louis, USA) was used as catalyst. The reaction was maintained at 200 °C for about 2 h. After the product was purified by vacuum distillation to remove the excess of methyl salicylate. Then the butyl ethylene glycoldisalicylate was characterized by Nuclear Magnetic Resonance Spectroscopy (NMR) and by Fourier Transform Infrared Spectroscopy (FTIR) and showed the peak narrow of the hydroxyls in the spectral range from 3300 cm −1 .
2.2
Calcium phosphate-based cements formulation
Three experimental ( e ) root canal sealers (MTA e , MTA e -HA, MTA e -DCPD) were prepared by mixing a base paste and a catalyst paste. The composition is shown in Table 1 . The catalyst paste contained MTA with or without addition of calcium phosphate, Resimpol 8 resin and titanium dioxide. Three different catalyst pastes were formulated by changing the amount of MTA (60% or 40% in weight) and the type of calcium phosphate (HA or DCPD 20% in weight). The base paste contained the synthesized component 1,3 butyl ethylene glycol disalicylate and the bismuth oxide. MTA Fillapex (Angelus, Londrina, Brazil) was used as control.
Materials | Composition | Manufacturers and batch numbers |
---|---|---|
MTA e | Base paste | |
Bismuth oxide (60%) | Vetec, Duque de Caxias, Brazil, 010127-TB | |
Butyl ethylene glycol disalicylate (40%) | Prepared at Federal University of Pelotas, Brazil | |
Catalyst paste | ||
MTA (60%) | Angelus, Londrina, Brazil, batch number 090605 | |
Resimpol 8 (39%) | Bandeirante Brazmo, São Paulo, Brazil, 81319 | |
Titanium dioxide (1%) | Vetec, Duque de Caxias, Brazil, 06092005 | |
MTA e -HA | Base paste | |
Bismuth oxide (60%) | Vetec, Duque de Caxias, Brazil, 010127-TB | |
Butyl ethylene glycol disalicylate (40%) | Prepared at Federal University of Pelotas, Brazil | |
Catalyst paste | ||
MTA (40%) | Angelus, Londrina, Brazil, 090605 | |
Resimpol 8 (39%) | Bandeirante Brazmo, São Paulo, Brazil, 81319 | |
Hydroxyapatite (20%) | Sigma–Aldrich, St. Louis, USA, MKBC4763V | |
Titanium dioxide (1%) | Vetec, Duque de Caxias, Brazil, 06092005 | |
MTA e -DCPD | Base paste | |
Bismuth oxide (60%) | Vetec, Duque de Caxias, Brazil, 010127-TB | |
Butyl ethylene glycol disalicylate (40%) | Prepared at Federal University of Pelotas, Brazil | |
Catalyst paste | ||
MTA (40%) | Angelus, Londrina, Brazil, 090605 | |
Resimpol 8 (39%) | Bandeirante Brazmo, São Paulo, Brazil, 81319 | |
Dibasic calcium phosphate dihydrate (20%) | Sigma–Aldrich, St. Louis, USA, 1305078 | |
Titanium dioxide (1%) | Vetec, Duque de Caxias, Brazil, 06092005 | |
MTA Fillapex | (MSDS data) salicylate resin, natural resin, diluting resin, bismuth oxide, nanoparticulated silica, MTA and pigments | Angelus, Londrina, Brazil, 19595 |
2.3
Flow evaluation
The flow and working time tests were performed following the International Organization for Standardization (ISO) 6876:2001 . After the material’s handled 0.05 mL of the sealer was dropped on the center of one glass plate (40 m × 40 m and 5 mm in thickness). At 180 s after initiating the mixing the second glass plate with same dimensions and weighing 20 g was placed centrally on top of the material followed by a 100 g weight to make a total mass of 120 g. Seven minutes later the weight was removed and the maximum and minimum diameters of compressed disk were measured using a digital paquimeter. Three determinations were made for each sealer tested ( n = 3). The test was repeated when the two diameters were different from each other more than 1 mm. The mean values and standard deviation (expressed in mm) were calculated and recorded (mm) to obtain the flow rates.
2.4
Setting time test
Setting time test was performed following the specification no. C266-03 of American Society for Testing and Materials (ASTM) . The different sealers ( n = 3) were compacted to excess into PVC molds (10 mm in diameter and 2 mm thick) placed on flat glass plate (1 mm thick). After 120 ± 10 s from the end of mixing, the sample was placed in the cabinet at 37 °C and 95% relative humidity. At 150 ± 10 s after initiating the mixing the Gilmore needle weighing 100 ± 0.5 g with 2.1 mm diameter was placed carefully on the material surface. This operation was repeated until the indentations could not be observed on the sealer surface. The elapsed time between the end of mixing and the failure of the indentation was recorded as initial setting time. Then, the Gilmore needle weighing 456 ± 0.5 g with 1 mm diameter was used as previously described and the time recorded as final setting time. The initial and final setting times of each specimen ( n = 3) were recorded.
2.5
Working time test
Working test was performed following the same procedure used in flow test (ISO 6876:2001) , with the difference of the increase in the intervals between the initial mixing and the setting time. Freshly mixed material was used on each time. The working time was determined when the specimen diameter was 10% less than flow value. Three samples for each experimental sealer were made and the mean was taken as sample working time.
2.6
Solubility
The solubility test was carried out as described by Gandolfi et al. . All materials were placed into PVC molds with 8 mm of internal diameter and 1.6 mm of height and then weighed using an analytical balance with 0.001 g accuracy (Bel Engineering series M, Monza, Italy). Each weight measurement was repeated three times and the mean recorded as Initial weight. PVC matrices were made to serve as support for each sample and to avoid that any surface of the specimen came into contact with the container. So, the entire surface of the sample was in contact with the water. Each sample ( n = 10) was placed in a cylindrical polystyrene-sealed container with 20 mL of deionized water at 37 °C. The solubility of the materials was calculated according to ISO 6876:2001 . At 1, 7, 14 and 28 days the samples were removed from the solutions, blotted dry at 37 °C for 48 h to constant weight (dry weight) and discarded.
The solubility (percentage weight variation) at each t time was calculated according to the following equation:
Solubility = Dry weight at time t − Initial weight Initial weight × 100
Any specimen that showed evidence of disintegration was discarded and the test repeated .
2.7
Water absorption
The water uptake was determined gravimetrically and was carried out as described by Gandolfi et al. . The procedure was the same used in solubility test. The samples were handled and placed into PVC molds. Each sample ( n = 10) was placed in a cylindrical polystyrene-sealed container with 20 mL of deionized water at 37 °C. There was not contact between the sample and container. The water absorption of the materials was calculated according to ISO 6876:2001 . At 1, 7, 14 and 28 days the specimens were carefully removed from container, wiped free from any visible surface moisture (blotted dry on filter paper for 3 s to remove the surface water)and weighed. Each weight measurement was repeated three times and the mean recorded as Wet weight. Then the samples were blotted dry at 37 °C for 48 h until a weight stabilization (Dry weight).
The water absorption at each time t was calculated as follows:
Water absorption = Wet weight at time t − Dry weight Dry weight × 100
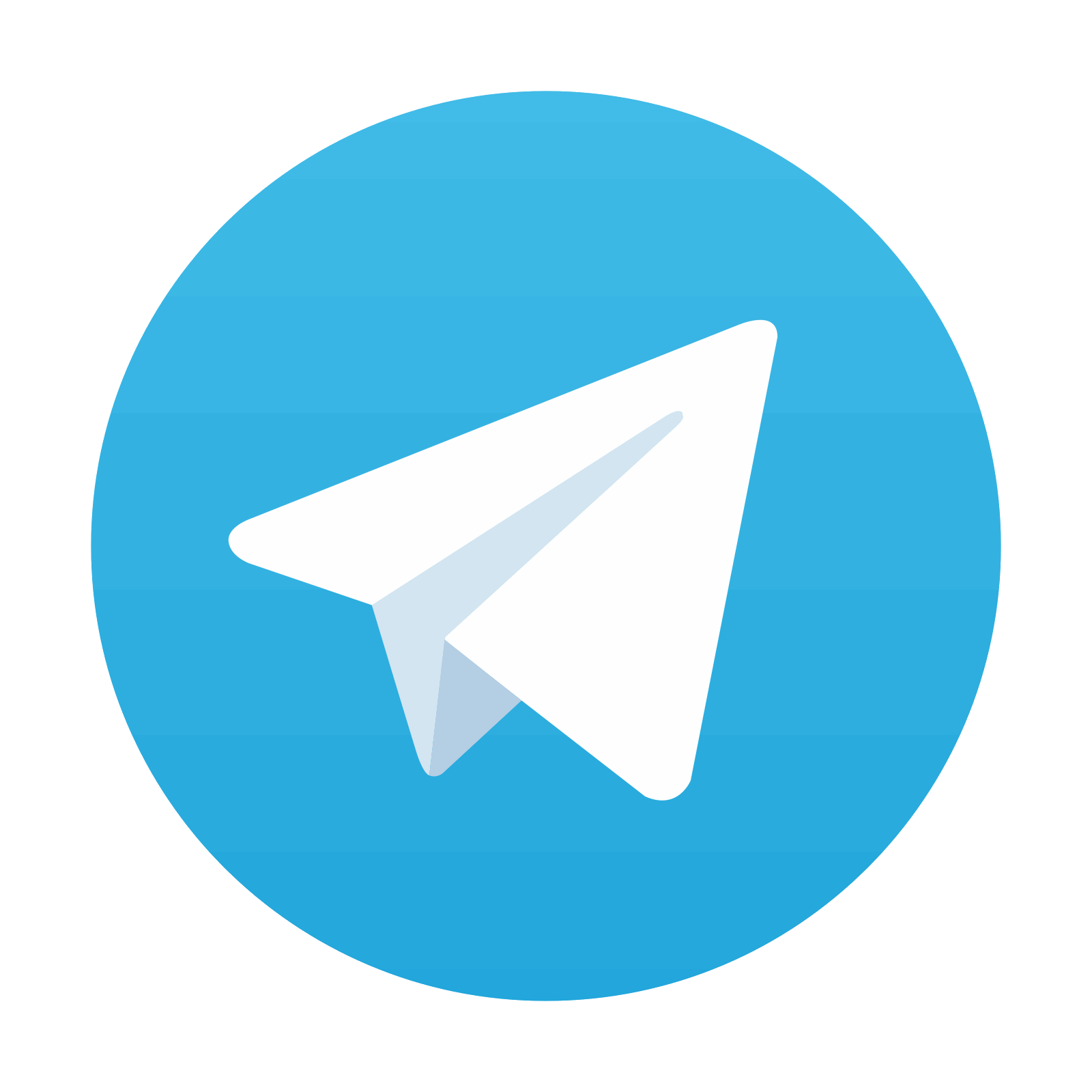
Stay updated, free dental videos. Join our Telegram channel

VIDEdental - Online dental courses
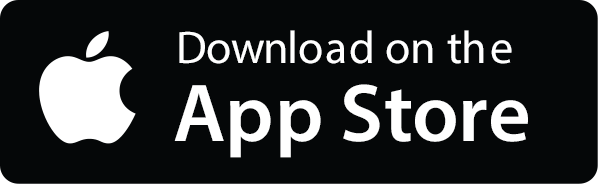
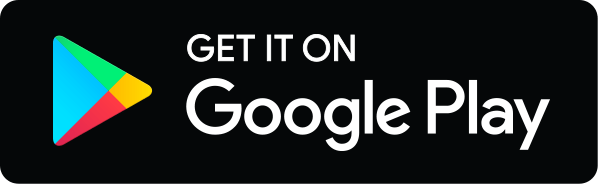