Introduction
The purposes of this study were to quantify bone damage associated with insertion of 2 types of miniscrew implants and to relate the amount of bone damage to monocortical plate thickness.
Methods
Nondrilling (n = 28) and self-drilling (n = 28) miniscrew implants (6 × 1.6 mm, Dentaurum, Newtown, Pa), and pilot holes (n = 26) were placed bilaterally in the maxillae and the mandibles of 5 adult dogs immediately after death. Bone blocks were cut, bulk stained with 1% basic fuchsin, embedded in methyl methacrylate, sectioned, and mounted. Monocortical plate thickness was measured adjacent to the miniscrew implant insertion site. Damage amounts were quantified at distances of 0 to 0.5 mm (adjacent region) and 0.5 to 1 mm (distant region) from the bone-implant interface. Total fractional damaged area (%), fractional microcracked area (%), and fractional diffuse damaged area (%) were quantified by using standard histomorphometric methods.
Results
The mean monocortical plate thickness of the specimens from the mandible (2.2 mm) was significantly ( P <0.001) greater than that of the maxillary specimens (0.9 mm). In the mandible, the 3 damage parameters were greater with self-drilling miniscrew implants than with nondrilling miniscrew implants; however, there were no differences in the damage parameters in the maxilla.
Conclusions
Bone damage accumulation is related to the type of miniscrew implant and the thickness of the bone.
The use of skeletal anchorage in orthodontics is not a new concept, but its routine application and acceptance in orthodontic practice has been more recent. Like other advances in orthodontics, the clinical application of skeletal anchorage has preceded a thorough understanding of the biologic and biomechanical bases. Survival rates of miniscrew implants used for orthodontic anchorage range from 57% to 95.3%, with most studies reporting survival rates around 84%.
Several studies have attempted to address factors responsible for the success of miniscrew implants. Primary stability is generally accepted to be important and can be measured by evaluation of insertion torque, removal torque, and pull-out strength. Variables that result in higher primary stability include smaller pilot hole diameters, increased cortical bone thickness, increased bone density, and use of self-drilling miniscrew implants. Unlike nondrilling miniscrew implants, self-drilling miniscrew implants have a sharp cutting tip and do not require a pilot hole before insertion. Miniscrew implants placed in the mandibles of beagles had greater primary stability than those placed in the maxillae. However, in humans, the success rates of miniscrew implants placed in the maxilla are consistently greater than those in the mandible. This suggests that other factors beside primary stability may be important in determining the success of miniscrew implants.
Although primary stability is generally associated with increased success, it is possible that factors that increase primary stability also increase the amount of bone damage associated with miniscrew implant insertion. For example, the sequence of microdamage and extensive remodeling may decrease bone-implant contact and lead to miniscrew implant failure.
There is a need to understand how implant-related and bone-related factors influence the level of bone damage. A few studies have investigated factors that alter the amount of bone damage associated with miniscrew implant insertion. For example, greater microdamage was associated with asymmetric thread profiles of implants, increased diameter and conical shape of miniscrew implants, and overtightening of miniscrew implants. A recent qualitative study demonstrated that self-drilling miniscrew implants created greater bone damage than did nondrilling miniscrew implants. However, quantitative data from the jaw bone are lacking. The objective of this study was to quantify the amount of bone damage associated with the insertion of self-drilling and nondrilling miniscrew implants in bone of different monocortical thicknesses in nonvital tissues.
Material and methods
Five mixed-breed dogs, ages 2 to 2.5 years, were obtained immediately after euthanasia. These animals had been used as the healthy controls in an unrelated study that had Institutional Animal Care and Use Committee approval. Each dog was killed by intravenous administration of pentobarbital, as recommended by the American Veterinary Medical Association Panel on Euthanasia. Immediately after death, orthodontic miniscrews (6 × 1.6 mm; Dentaurum, Newtown, Pa) were placed at predetermined locations, bilaterally, in the jaws. A horizontal incision was made in the soft tissues to expose the underlying maxillary and mandibular bones. With the intent of sampling bone with a range of thicknesses, we inserted miniscrew implants bilaterally in the posterior maxilla, middle mandible, and posterior mandible of each dog ( Fig 1 ). In each selected location, a triad of a pilot hole, a pilot hole followed by a nondrilling miniscrew implant, and a self-drilling miniscrew implant without a pilot hole was placed in the bone about 2 to 3 mm apical to the roots and about 7 to 10 mm apart from each other ( Fig 1 ). Totals of 30 miniscrew implants of each type and 30 pilot holes were placed. The order of placement of the miniscrew implants and pilot holes was randomized for each dog, and this order was used for all triads in a dog. A slow-speed hand piece and a short pilot drill bit (TOMAS, 4 × 1.1 mm, Dentaurum) were used for placement of the pilot holes. Miniscrew implants were placed with a screwdriver (TOMAS; Dentaurum) according to the manufacturer’s instructions.
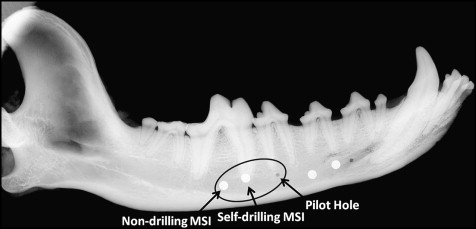
Once all miniscrew implants were placed in a dog, the maxilla and the mandible were harvested. The jaws were cut into left and right halves with a band saw (Mar-Med, Cleveland, Ohio). Images (Faxitron X-Ray, Lincolnshire, Ill) were obtained to orient the jaws for sectioning and also to evaluate the miniscrew implants for root proximity or direct root contact ( Fig 1 ). Two pilot holes in the posterior maxilla, 2 nondrilling miniscrew implants in the posterior mandible, and 4 self-drilling miniscrew implants in the posterior mandible were replaced when it was apparent that root contact had occurred.
The miniscrew implants were removed by reversing the screws. The jaws were then sectioned into blocks with the band saw. Blocks were stored in 70% ethyl alcohol for 24 hours and then bulk stained with 1% basic fuchsin (B660-03; J. T. Baker, Phillipsburg, NJ) in a graded series of alcohol under vacuum. After staining, the blocks were washed twice in 100% ethyl alcohol for 5 minutes. The blocks were sequentially infiltrated in methyl methacrylate, and then methyl methacrylate and 4% dibutyl phthalate under vacuum for 3 days each. All blocks were then embedded in catalyzed methyl methacrylate and sectioned buccolingually perpendicular to the bone surface and to simulate the longitudinal axis of the implant with a Diamond Wire Histo-Saw (model 3241; Delaware Diamond Knives, Wilmington, Del) with a 0.3-mm diamond blade into sections about 80 to 100 μm in thickness. The sections were mounted on microscope slides.
Eight specimens could not be successfully sectioned; thus only 82 (26 pilot holes, 28 self-drilling, and 28 nondrilling) of the 90 possible specimens were quantified. By location, the final numbers of specimens were 9 posterior maxillary pilot holes, 10 posterior maxillary nondrilling miniscrew implants, 10 posterior maxillary self-drilling miniscrew implants, 8 middle mandibular pilot holes, 9 middle mandibular nondrilling miniscrew implants, 9 middle mandibular self-drilling miniscrew implants, 9 posterior mandibular pilot holes, 9 posterior mandibular nondrilling miniscrew implants, and 9 posterior mandibular self-drilling miniscrew implants. Each slide was examined to identify the section located closest to the center of the implant or pilot hole defect. This section was selected for quantification of bone damage associated with each type of miniscrew implant or pilot hole drill insertion. The slides were blinded to conceal jaw location and type of miniscrew implant. All data were collected by 1 investigator (S.B.S.). Each specimen was examined by using an epifluorescent microscope (BX 51; Olympus, Tokyo, Japan) at 200× magnification. The first millimeter of buccal cortical bone adjacent to the insertion site was examined ( Fig 2 ). Bone at 0 to 0.5 mm from the implant interface was defined as the “adjacent region,” and bone 0.5 to 1 mm from the implant interface was called the “distant region.” The rationale for division into these regions was based on our observation that most damage was limited to the first 1 mm from the interface, and a general decrease was noted in the damage from the adjacent to the distant regions. By examining at a high magnification (200×), we were able to clearly visualize the damaged bone ( Fig 3 ). Amounts of bone damage on both the apical and coronal sides of the insertion site were quantified by using standard histomorphometric methods. Two features of microdamage were quantified for the cortical bone specimens: microcracks defined as small linear defects typically 100 μm in length, and diffuse damage: patches of intensely stained mineralized matrix that have been disrupted by local intense deformation. Other forms of microdamage such as crosshatching and microcallus fractures are more frequently observed in trabecular bone and thus were not included in our study of cortical bone. Using a Merz grid, the following histomorphometric variables were quantified: bone hits, hits on microcracks, and hits on diffuse damage. The thickness of the buccal plate of cortical bone was also measured on both the apical and coronal sides of the insertion site by using a calibrated computer program (MicroSuite; Olympus, Center Valley, Pa). Six randomly chosen specimens were requantified 3 weeks later to evaluate intrarater reliability.
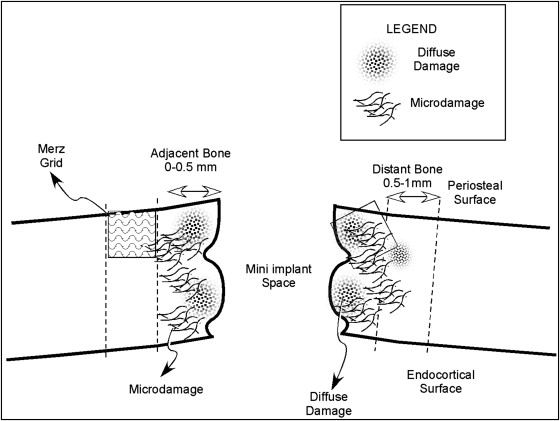
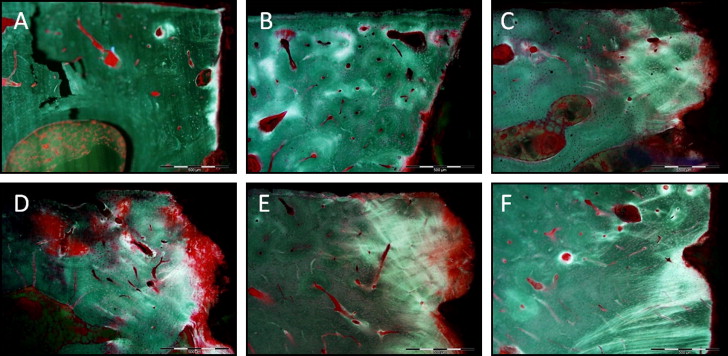
From the primary variables, the following secondary parameters were calculated: total fractional damaged area ([microcrack hits + diffuse damage hits]/bone hits∗100), fractional microcracked area ([microcrack hits/bone hits]∗100), and fractional diffuse damaged area ([diffuse damage hits/bone hits]∗100). The total fractional damaged area represented the percentage of bone with damage, whether diffuse damage or microcracks. Mean values for diffuse damage and microcracks were summed to represent the total amount of damaged bone in each specimen. Although the 2 types of damage are distinct, the addition of these values represented the fraction of bone area that was damaged and, conversely, indicated the amount bone that was undamaged.
Means and standard deviations were calculated for all specimens. Intraclass correlation coefficients were used to describe intrarater reliability. Intraclass correlation coefficients were calculated for monocortical bone thickness, fractional microcracked area, and fractional diffuse damaged area. Repeated measures analysis of variance (ANOVA) with Tukey-Kramer adjustments was used to compare the cortical plate thickness from the 3 regions and to describe the differences in total fractional damaged area, fractional microcracked area, and fractional diffuse damaged area between types of miniscrew implants, between jaws, and between adjacent and distant regions. Significance was set at P <0.05.
Results
The intraclass correlation coefficient values for monocortical thickness, fractional microcracked area, and fractional diffuse damaged area were 1.0, 0.99, and 0.95, respectively. Means (standard deviations) for monocortical bone thickness measurements of specimens from the posterior maxilla, middle mandible, and posterior mandible were 0.92 mm (0.25), 2.27 mm (0.39), and 2.20 mm (0.22), respectively. The monocortical thickness of specimens from the posterior maxilla was significantly different from that of the middle mandible and the posterior mandible ( P <0.001), whereas the middle mandible and the posterior mandible were not significantly different ( P = 0.46). Therefore, specimens from the middle and posterior mandible were combined and compared with the maxillary specimens.
Means and standard deviations of total damage fractional area, microcrack fractional area, and diffuse damage fractional area are presented in the Table . In all specimens, the amount of damage decreased as the distance from the implant-bone interface increased. Self-drilling miniscrew implants had significantly greater total fractional damaged areas ( P <0.005) and fractional microcracked areas ( P <0.02) than did nondrilling miniscrew implants in the distant regions of the mandible. A significantly greater fractional diffuse damaged area was associated with the self-drilling miniscrew implants than with the nondrilling miniscrew implants ( P <0.005) in the mandibular adjacent region. The ratio of microcracks to diffuse damage increased as bone thickness increased and as distance from the bone-implant interface increased in both the self-drilling and nondrilling groups. There were no statistically significant differences in any damage parameter between miniscrew implant types in the maxillary specimens.
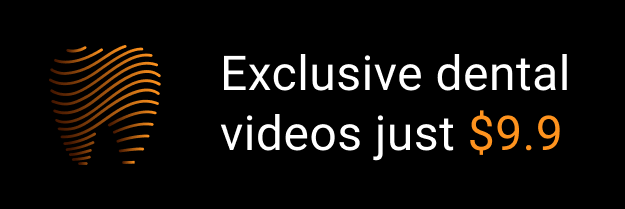