Biomechanics and Treatment Principles
The treatment modality for each individual with an orthodontic or an orthognathic problem will vary greatly, depending on several factors. Every malocclusion and skeletal discrepancy cannot be treated using the same treatment protocol. Although the biomechanics are the same, they are used in various ways. This chapter focuses on the fundamentals of tooth movement and provides a summary of anchorage.
Biomechanics
Orthodontic biomechanics is the study of the biological systems involved in tooth movement. Control of orthodontic treatment is achieved by understanding the reactions of dental and facial structures to orthodontic forces. Thus, for better decision making and efficiency of care, it is essential to have knowledge of the factors governing tooth movement.
The Mechanics of Tooth Movement
Force, Moment, Couple
Physical properties are categorised as scalars or vectors. Scalars do not have a direction but do have a definite magnitude; for example, weight. Vectors have both magnitude and direction; for example, force. The force applied to teeth with a tendency to cause rotation is known as the moment of force. The size of this component of force is mathematically calculated by multiplying the magnitude of the applied force by the perpendicular distance between the point of application and the centre of resistance (Gill, 2008). This is important in orthodontics as force is applied to the crown of a tooth since it cannot be directly applied to the centre of resistance located on the root. Consequently, rotation of the tooth occurs (Figure 4.1).


Figure 4.1 Force: A) Force (F) must be applied directly to the centre of resistance (CR) of an object to move it in the direction of the force. B) If force (F) does not pass through the centre of resistance (CR), rotation occurs.
Source: Courtesy of Professor Ali Darendeliler.
The term ‘couple’ is given to two parallel forces of the same magnitude in opposing directions to rotate a tooth. The size of this component of force is calculated by multiplying the magnitude of applied forces by the distance between them. To describe a moment or couple, the term torque is used in orthodontics (Gill, 2008).
Centre of Resistance, Centre of Rotation
The centre of resistance is the point of resistance to movement. This point is usually located in the middle of an object in a homogenous environment (Figure 4.1A). In a heterogeneous environment, the centre of resistance is towards the denser side (Figure 4.2). The root of the tooth is embedded in bone; therefore, the centre of gravity or point of balance of the tooth is more apically located due to bone density (Figure 4.3). On single rooted teeth, the centre of resistance is half way between the root apex and the alveolar bone crest. In a multi‐rooted tooth, the centre of resistance is one to two millimetres apical to the furcation. The centre of resistance is influenced by length of the root and height of the alveolar crest. Longer roots have a lower centre of resistance. As bone loss increases, the centre of resistance becomes located further apically. This is because the density of the environment greatly affects the centre of resistance. Bodily movement occurs as the force passes through the centre of resistance. If force does not pass through the centre of resistance, tipping results.

Figure 4.2 The centre of resistance (CR) is towards the denser side and not in the centre of the object.
Source: Courtesy of Professor Ali Darendeliler.

Figure 4.3 The tooth is embedded in bone, therefore the centre of resistance (CR) is not in the centre of the tooth. As the bone is more dense, the centre of resistance is located further apically.
Source: Courtesy of Professor Ali Darendeliler.
The centre of rotation is the rotation of a body from its original position (Figure 4.4). Tooth movement does not alter the centre of resistance; however, the centre of rotation varies depending on the type of tooth movement; for example, the centre of rotation lies at the apex for tilting the crown of the tooth while the centre of rotation lies at infinity if the crown and the root are moved as a unit.

Figure 4.4 Centre of rotation.
Source: Courtesy of Professor Ali Darendeliler.
The Biology of Tooth Movement
Collagenous supporting fibres called periodontal ligaments anchor the tooth in the bony socket by maintaining a strong attachment between the alveolar bone, gingiva and the root cementum. Tooth movement is made possible with the presence of periodontal ligaments and their cellular elements, such as undifferentiated mesenchymal cells and tissue fluid in the periodontal ligament space. There are two theories supporting the biological control of tooth movement, the bioelectric theory and the pressure tension theory.
Bioelectric Theory
The electricity travelling through the periodontal ligaments is piezoelectric. The piezoelectricity initiates a rapid response but, regardless of the force sustainability, it decays or dies away quickly to zero. Another characteristic is reaching equilibrium upon release of force. It is believed that piezoelectric responses are caused by the bending of the alveolar bone. The flex of the bone causes a localised production of prostaglandins, which facilitates tooth movement. This theory supports the fact that tooth movement is carried out by stress on the alveolar bone rather than the periodontal ligaments. Some studies suggest that forces stress the bone, precipitating electrical charges in response to the deformities. Negative polarity on the concavities results in bone deposition and positive polarity of the convex surfaces of the bone results in resorption. These bioelectric variances lead to bone remodelling (Proffit, 2007).
Pressure Tension Theory
The shock of the force is absorbed within the periodontal ligament space and a cascade of chemical reactions occurs to make tooth movement possible (Figure 4.5). The direction of the force is the decisive factor for which part of the supporting structures of the tooth undergo pressure or tension. When force is applied to teeth, the periodontal ligament undergoes pressure on the side towards the movement and tension on the opposite side away from the movement. The side with compression and pressure undergoes resorption by bone resorptive cells (osteoclasts) as the alveolar bone deforms and blood supply to the area increases, resulting in mobilisation of cells such as osteoclasts. On the tension side, an increased blood flow is evident to the area owing to stretching of the ligaments.

Figure 4.5 Tension and compression.
In response to force, osteoblasts produce prostaglandin E2 and leukotrienes, owing to the deformities developed in the periodontal ligaments and the bone. These cells are responsive to mechanical loading and are found in areas of inflammation. Prostaglandin E2 is a mediator in bone resorption and leukotrienes increase intracellular messengers to the site. Examples of these intracellular messengers are interleukin‐1 and macrophage colony‐stimulating factor (MCSF). Interleukin‐1, produced by osteoblasts and macrophages, stimulates bone resorption and inhibit bone formation by increasing prostaglandin synthesis. MCSF is also produced by osteoblasts to coordinate osteoclasts proliferation and differentiation. As these messengers are recruited to the site, an increased production of osteoblasts and receptor activator of nuclear factor kappa‐B ligand (RANKL) is evident. RANKL is secreted by osteoblasts as a stimulating factor for differentiation, fusion and activation of osteoclastic cells. This factor is essential in osteoclast survival. As a result of these events, macrophages produce more interleukin‐1, leading to an increased level of RANKL. RANKL and MCSF attract additional blood monocytes to the site. The blood monocytes fuse to form osteoclasts and become activated by RANKL.
Osteoid is exposed to allow access to the underlying mineralised bone. Osteoid is a newly formed layer of bone as a result of osteoblastic activity; it matures within about ten days (Foster, 1990). Matrix metalloproteinases are produced by osteoblasts to break down the osteoid and permit access to the underlying mature bone. Osteoclasts are recruited to the site and secrete hydrogen ions into the matrix. These ions soften the hydroxyapatite crystals and degrade the extracellular matrix with proteases. These events lead to bone resorption. The activity of bone resorptive cells are inhibited by the production of enzymes and cytokines. Bone resorption is strictly controlled by osteoblasts, as it produces cells such as osteoprotegerin to block the effect of RANKL. Osteoblasts are vital in the coordination of bone homeostasis.
On the side with tension, a series of events takes place leading to bone formation. Owing to a tensile force, the osteoblasts flatten and allow access to osteoid. The periodontal ligaments respond to these changes by increasing the levels of specific secondary messengers (extracellular signal‐regulated kinase, ERK). ERK increases the activity of osteoblasts, and thus bone formation, by inducing expression of runt‐related transcription factor 2 (RUNX2). The fibroblasts of periodontal ligament differentiate into osteoblasts induced by RUNX‐2 (Mitchell, 2001).
These cascades of events lead to bone remodelling, which is responsible for tooth movement. These alterations occur for the bone to accommodate movement of the bony socket from one place to another and to make orthodontic tooth movement possible.
Orthodontic Forces
Light continuous forces permit tooth movement as hyperaemia (increased blood flow) recruits osteoblasts and osteoclasts to the site, causing frontal resorption. This type of resorption takes place in the inner wall of the alveolar bone between the root and the lamina dura. Constant gentle forces resorb the bone on the side of the socket, which undergoes pressure as bone deposition takes place on the side of the socket with tension. The tensile pressure increases vascularity and osteoid develops at the site, which is more resistant to resorption in comparison with alveolar bone.
Strong continuous forces result in ischaemia (inadequate blood flow) of the periodontal ligaments due to extreme compression and pressure. This leads to undermining resorption of the socket wall. Excessive heavy forces crush and occlude the periodontal ligaments, resulting in tissue necrosis (cell death). This may lead to unwanted tooth resorption or ankylosis (the fusion of tooth and bone). Ankylosis occurs as the tooth contacts the bone from compression of the ligaments.
Heavy forces cause hyalinisation of the periodontal ligaments. Since hyaline tissue degenerates, the tissues appears translucent and smooth as they lose their cellular elements. This zone disappears with resorption by osteoclasts. During compression, cells from periphery tissues can also invade the hyalinised tissue and obliterate the layer.
The force magnitude and duration is responsible for the type of resorption that takes place. Frontal resorption (Figure 4.6) is ideal for tooth movement and less painful for the patient, although undermining resorption (Figure 4.7
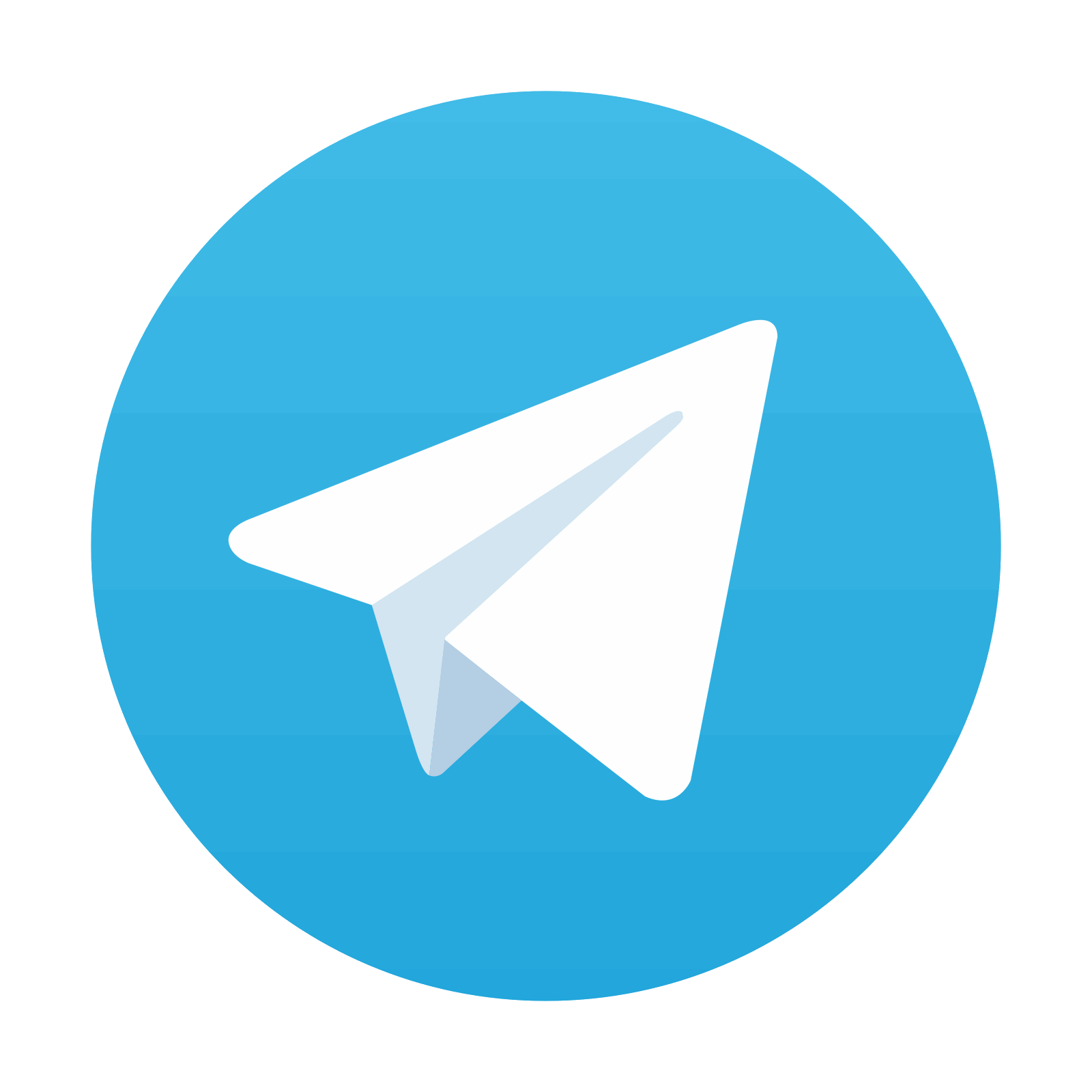
Stay updated, free dental videos. Join our Telegram channel

VIDEdental - Online dental courses
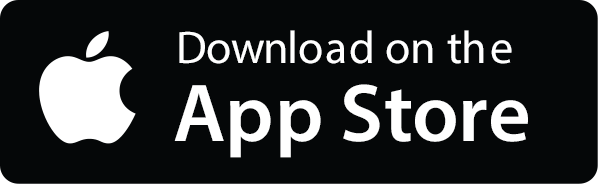
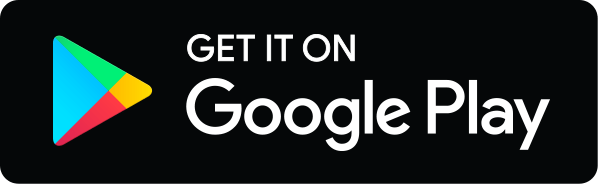