Abstract
Objective
Dentin remineralization is of considerable clinical interest for dentin hypersensitivity and developing biomimetic analogs that can regulate hydroxyapatite (HAp) nucleation and growth remains a challenge. This study aimed to evaluate in vitro the potential for dentin remineralization using the following biomimetic in situ prepared poly(catechols): poly(dopamine), poly(DOPA), poly(caffeic acid) and a synthesized DOPA-peptide possessing collagen and calcium-binding domains (DOPA-Ahx-(Gly) 3 -(Glu) 5 ).
Methods
Dentin samples were immersed in a freshly prepared phosphate-buffered saline (PBS) containing the respective catechol and laccase. After the reaction, they were immersed in calcium and phosphate remineralization solution, which was changed every day for 10 days. Samples of intact and demineralized dentin were used as control groups and kept in deionized water under the same experimental conditions. The remineralized dentin was characterized by scanning electron microscopy (SEM), Micro-energy dispersion X-ray fluorescence spectroscopy (μEDX) and X-ray diffraction (XRD).
Results
The application of different poly(catechols) and DOPA-peptide promoted crystal nucleation and the formation of HAp, which partially covered both the dentin surface and dentinal tubules walls.
Significance
By mimicking the role of charged non-collagenous proteins in vivo , polymers consisting of catechol groups showed the ability to modify demineralized dentin surface properties, promoting mineral formation. The use of poly(catechols) may be encouraged for the development of a therapeutic technique for dentin hypersensitivity.
1
Introduction
Dentin hypersensitivity (DH) is an increasing clinical manifestation that affects a large part of the world’s population (11.5%) [ ]. Many etiological and predisposing factors have been identified, but all of these are related to changes in dental structure that result in the exposure of the dentinal tubules to the oral environment [ ]. Once exposed, the rapid displacement of the fluid located inside the dentinal tubules over different stimuli causes a deformation of the pulp nerve fibers (type A-δ, A-β or C), resulting in painful symptomatology [ ] that can affect quality of life [ ].
Despite the extensive number of therapeutic agents manufactured for self or professional administration [ ], all are based on two objectives: obliterating the dentinal tubule to prevent movement of fluid, or a neural blockade of the pulp receptors, or both simultaneously [ ]. However, the pulp receptors’ neural blockade is reversible, and as the daily acid challenge negatively affects the maintenance of the plugs that obliterate the dentinal tubules, the pain relief is compromised and leads the patient to require constant treatment in both situations [ ]. Thus, there is currently no effective long-term treatment, and for that reason, DH is still considered challenging [ ]. Markowitz and Pasheley [ ] proposed that new treatments for DH should make dentin more resistant to both mechanical and chemical attack and should mimic the natural desensitizing process, leading to a spontaneous occlusion of the dentinal tubules over time; an ideal treatment would, therefore, produce non-sensitive and sclerotic dentin.
Within this context, a promising method is the induction of mineral formation by dentin remineralization methods. By definition, it is a process that replaces the minerals of demineralized dentin. However, due to its hierarchical structure in the form of tubules and its high organic matter content (20%), the dentin remineralization process is very complex and difficult to obtain [ ]. Although they represent less than 10% of the organic content of dentin, studying the structures and functions of non-collagenous proteins (NCPs) becomes a general strategy because these proteins are responsible for regulating the processes of nucleation and growth of HAp crystals through their amino acid domains (AA) on natural biomineralization [ ]. Considering their properties, researchers try to synthesize or find in nature analogs capable of mimicking the role of NCPs in dentin remineralization.
Inspired by the chemistry of mussel adhesive proteins, catechol-containing compounds have shown the ability to self-assemble over both organic and inorganic materials, serving as a template for surface biomodification [ ]. Zhou et al. [ ] evaluated the potential of remineralization through the use of poly(dopamine) on demineralized dentin and demonstrated that the coating formed over the dentin promoted remarkable remineralization, in which all the tubules were occluded by densely compacted HAp crystals. Despite the theoretical potential, their study was only found to be evaluating the remineralization potential through the use of catechol-containing compounds on demineralized dentin, and for this reason, we decided to extend the knowledge and also evaluate poly(DOPA) (DOPA = 3,4-dihydroxyphenylalanine) and poly(caffeic acid). Moreover, Zhou et al. [ ] kept the dentin samples on the biomimetic solution for 24 h. Although catechols are able to self-polymerize under oxygen exposure [ ], it is necessary to accelerate this reaction for clinical applications. Therefore, we included the Trametes versicolor laccase on the solution due to its ability to catalyze the oxidation reaction of various aromatic compounds [ ] and to achieve the highest redox potential among laccases [ ].
Considering that both positively and negatively charged NCPs are necessary for HAp precipitation [ ], we decided to synthesize a peptide based on charge potential. For this purpose, a simple peptide with a domain of glutamic acid for calcium binding and a short rigid spacer was synthesized, herein called the remineralization peptide. For the immobilization on the dentin surface, a DOPA-amino acid was attached to the designed peptide by a flexible aminohexanoic acid (Ahx)-linker. The peptide DOPA-Ahx-(Gly) 3 -(Glu) 5 was designed to interact with the collagen through a bond between the catechol groups (DOPA) and the organic surface [ ], while the multiple glutamic acid (L-Glu) residues attract calcium(II) ions for the dentin remineralization process [ ] and even di-glutamate possesses a strong binding for calcium(II) [ ]. The three glycine residues are used as a further spacer in the peptide. Furthermore, the combination of glycine with glutamic acid increases the calcium(II)-binding affinity [ ].
Hence, the aim of this study was to evaluate the dentin remineralization potential in vitro of poly(dopamine), poly(DOPA), poly(caffeic acid) and a DOPA-containing remineralization peptide (DOPA-RP). The null hypothesis tested is that the proposed remineralization methods would not be able to produce a remineralization of dentin.
2
Materials and methods
2.1
Dentin samples preparation
This study was reviewed and approved by an Ethics Committee in Human Research under protocol #80645317.9.0000.5418. Extracted sound human third molars were collected, and the soft tissue attached to them was removed. The teeth were stored in a 0.5% thymol solution for at least 1 month prior to the experiment. Each tooth was sliced perpendicular to its longitudinal axis using an IsoMet Low Speed diamond saw (Buehler, Lake Bluff, IL, USA). The dentin surface exposed was polished on 600-, 1200- and 2000-grit silicon carbide papers, respectively, under running water (Buehler EcoMet 5, Lake Bluff, IL, USA) and ultrasonically cleaned for 10 min between each grit (Ultrasound Ultrason 1440 D-Odontobrás Ind. E Com. Med. Odont. Ltda, Rio Preto, SP, Brazil). A second slice, also perpendicular to the longitudinal axis, was made in order to obtain a 1.5 mm dentin slice. Each slice was then sectioned in half using a surgical chisel and hammer, with exception for the samples analyzed by SEM, which were fractured only after the incubation period. Dentine samples without defects on dental structure as, staining, cracks or other anomalies were selected for use in this study. They were stored in deionized water in a refrigerator at 4 °C prior to their use.
2.2
Peptide synthesis
The peptide DOPA-Ahx-(Gly) 3 -(Glu) 5 (DOPA-RP) was obtained by solid phase peptide synthesis (SPPS) on a 433A peptide synthesizer (Applied Biosystems, Foster City, CA, USA). All applied amino acids were Fmoc-protected at the α-amino group. In the case of DOPA, the orthogonal protecting group was acetonide (Merck Bioscience, Darmstadt, Germany) and for glutamic acid tert .-butyl (Iris Biotech GmbH, Marktredwitz, Germany). A glutamic acid preloaded TCP resin was applied with a size of 200–400 mesh (Intavis Bioanalytical Instruments AG, Tübingen, Germany). The synthesis was performed in NMP using the FastMoc™ protocol (HBTU (2-(1 H -benzotriazol-1-yl)-1,1,3,3-tetramethyluronium hexafluorophosphate) in a solution of HOBt (1-hydroxybenzotriazole) in DMF (dimethylformamide) in the presence of DIEA (diisopropylethylamine) for coupling and a 20% solution of Piperidine in NMP for the Fmoc removal before coupling (all Iris Biotech GmbH, Marktredwitz, Germany)). The peptide was cleaved from the resin by a mixture of 95% TFA (trifluoroacetic acid)/2.5% water/2.5% triisopropylsilane. After the cleavage, the peptide was obtained by precipitation in ice-cold methyl tert .-butyl ether. The precipitated peptide was centrifuged, and the supernatant was discarded. The remaining peptide was solubilized in UHQ water and freeze-dried in a lyophilisator (Martin Christ Alpha 1–4, Osterode am Harz, Germany). The obtained peptide was characterized by MALDI-ToF MS (Autoflex speed, Bruker Daltonik, Bremen, Germany) using α-CHCA (α-cyano-4-hydroxycinnamic acid) as a matrix.
2.3
Phase 1
To evaluate the influence of collagen fibers on dentin remineralization, the intact and demineralized dentin group were immersed on the remineralizing solution for 10 days and the surface morphology was analyzed by SEM. For this test, eight dentin samples were randomly divided into the two groups ( n = 4). 37% of phosphoric acid was used as the conditioning agent to promote demineralization of the dentin surface. It was applied with a disposable dripper on the polished dentin surface of the demineralized dentin group so as to completely cover it for 2 min. After, they were abundantly rinsed with deionized water and samples of both groups were placed at the bottom of sealed falcon tubes filled with 10 mL of the remineralizing solution, which was prepared according to the procedure described by Fan et al. [ ]. The solution contained 2.58 mM calcium (CaCl 2 ·2H 2 O) (Dinâmica, Indaiatuba, SP, Brazil), 1.55 M phosphate (KH 2 PO 4 ) (Dinâmica, Indaiatuba, SP, Brazil), 1 mg/L fluoride (NaF) (Thermo Scientific Orion, Waltham, Massachusetts, USA), and 180 mM NaCl (Dinâmica, Indaiatuba, SP, Brazil) and was buffered by 50 mM of tris(hydroxymethyl)-aminomethane (Tris)-hydrochloric acid (Dinâmica, Indaiatuba, SP, Brazil). The remineralizing solution pH was adjusted using 0.1 M HCl and 0.1 M NaOH to 7.6 and was stored at 4 °C prior to use. The remineralizing solution was replaced every day for 10 days.
2.4
Phase 2: biomimetic treatment
Sixty dentin samples were randomly divided into the following groups: poly(dopamine), poly(DOPA), poly(caffeic acid), DOPA-RP (experimental groups), intact dentin and demineralized dentin (control groups) ( n = 10). After being demineralized as described on phase 1, all dentin samples from the experimental groups were immersed in a freshly prepared solution containing 1 mg/mL of the respective biomimetic analog (dopamine, l -DOPA, caffeic acid [all from Sigma-Aldrich, Schnelldorf, Germany] and the synthesized peptide, DOPA-Ahx-(Gly) 3 -(Glu) 5 (DOPA-RP)) in phosphate-buffered saline (PBS) (pH 6) with 1 mg/10 mL of laccase from Trametes versicolor (ASA Spezialenzyme GmbH, Wolfenbüttel, Germany) for 12 h at 37 °C. After that, the dentin samples were rinsed with PBS solution (pH 6) to remove the unbound biomimetic analog. Then, the dentine samples were placed at the bottom of sealed falcon tubes filled with 10 mL of the remineralizing solution with the same composition used on phase 1 and stored at 37 °C. The remineralizing solution was replaced every day for 10 days. The dentin samples of the control groups were stored under the same conditions as the experimental ones but immersed in deionized water.
After the incubation period, the dentin samples were analyzed by scanning electron microscopy (SEM) ( n = 4), micro-energy-dispersive X-ray fluorescence (μEDX) ( n = 5) and X-ray diffraction (XRD) ( n = 1).
2.5
Characterization of the remineralization precipitates
The surface morphology of the samples was observed by SEM (JEM 1400, JEOL, Tokyo, Japan) with a beam voltage at 15 kV and a working distance of 10 mm. They were fixed with 2.5% glutaraldehyde for 12 h, gradually dehydrated using ethanol (at a gradient concentration of 25%, 50%, 75%, 95% and 100%) until a critical point (Critical Point Drying, CPD 030, Balzers, Liechtenstein) as the final dehydration step, and then sputter-coated with gold (Sputter Coater, SCD 050, Balzers Union Aktiengesellschaft, Liechtenstein).
The quantitative element analysis of Ca, P, and O as well as the Ca/P ratio was conducted by μEDX analysis (μEDX-1300, Shimadzu, Japan). The spectrometer used is equipped with a rhodium X-ray tube and a Si (Li) semiconductor detector cooled by liquid nitrogen (N 2 ) and was adjusted using a certified commercial reagent of stoichiometric hydroxyapatite (Aldrich synthetic, Ca 10 (PO 4 ) 6 (OH) 2 , 99.999 % purity, Lot 10818HA/SIGMA 2008) as a reference. The tension in the tube was set to 15 kV and measurements were collected under basic parameters for X-ray emissions of Ca and P elements, while OH was used for chemical balance. The incident beam diameter was adjusted automatically to 50 μm and three points (spot size 2 nm) per sample were collected for 100 s per point. The mean of the three points was used for statistical analyses. Bartlett’s test and the Shapiro-Wilk test were used to verify the homogeneity of the data, and the Kruskal-Wallis and Dunn post hoc tests were used to assess and compare the statistical differences between the groups, respectively ( p < 0.05).
The mineral phase and crystallinity were carried out by XRD using a diffractometer (D8 Advance, Bruker, Germany) with Cu Kα radiation. The data were collected in the 2θ range of 10–60° at a scan rate of 2° per min. The diffractogram’s software treatment (OriginLab, Northampton, USA) consisted of a subtraction of the baseline and normalization. The Rietveld method was performed in order to measure the size of the crystallites formed.
3
Results
3.1
SEM analysis
3.1.1
Phase 1
Phase 1 was performed to evaluate the surface of intact and demineralized dentin after immersion in the remineralizing solution for 10 days. In the group with intact dentin, it was not possible to observe the dentin tubules because the mineral deposited on its surface covered all of them ( Fig. 1 A and C). The group with demineralized dentin, on the other hand, presented surfaces with completely opened dentinal tubules, with little mineral content deposited on its surface, and with an absence of peritubular and intertubular dentin ( Fig. 1 B and D).

On the transverse sections, there was a crystal deposition on the lumen of the dentinal tubules of demineralized dentin group that was only observed for this group ( Fig. 1 E). This is probably due to the mineral layer formed over the surface, which covered the dentinal tubules’ entrance of the intact dentin group after 10 days of immersion on the remineralizing solution ( Fig. 1 F).
3.1.2
Phase 2
The control groups of phase 2 (intact and demineralized dentin) were stored in deionized water during the incubation time (10 days). The control group of intact dentin presented surfaces with opened dentinal tubules, with no mineral content deposited on the surface, and with peritubular and intertubular dentin present ( Fig. 2 A and C). On the transverse section, it was possible to observe natural characteristic of dentinal tubules, such as the presence of peritubular and intertubular dentin ( Fig. 4 A). In contrast, the control group that had been acid-etched with 37% phosphoric acid for 2 min presented surfaces with completely opened dentinal tubules, with no mineral content deposited on the surface, and with the presence of a demineralized collagen fiber matrix ( Fig. 2 B and D). On the transverse section it was possible to observe the effects of the superficial demineralization treatment on the dentinal tubules, such as the exposition of the demineralized collagen matrix (arrow), absence of peritubular dentin, and presence of accessory channels ( Fig. 4 B).
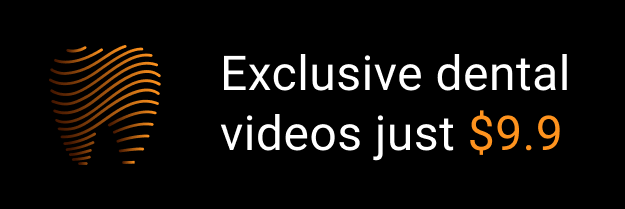