Abstract
Objective
Bioactive glasses and surface pre-reacted glass-ionomer (sPRG) filler possess cariostatic properties owing to ion release. Many studies investigated potential cariostatic effects; few studies evaluated the surface stability and the structural changes their surfaces undergo in acidic conditions.
Methods
The surface resistance against acid attack and the surface receptiveness for bacterial adhesion and biofilm formation of a sPRG-filled (Beautifil ll, Shofu) and conventional glass-filled (Herculite XRV Ultra, Kerr) resin-based composite (RBC), and a conventional glass-ionomer cement (GIC; Fuji IX GP Extra, GC) were examined. Specimens (n = 3) were immersed in distilled water or lactic acid (pH 4.0) for 3 days. Bacterial growth and biofilm formation were recorded using optical density and SEM.
Results
Upon 3-day immersion in lactic acid, the surface of the sPRG-filled RBC revealed multiple holes, while virtually no change in surface integrity was observed for the conventional RBC and GIC. Bacterial growth measurements revealed that none of the materials inhibited Streptococcus mutans (p < 0.05). Remarkably, cross-sectional SEM revealed that S. mutans had penetrated the etch pits induced by lactic acid in/around the sPRG filler. Ion-release measurements revealed that sPRG-filled RBC released boron and fluoride, while GIC only released fluoride. However, the concentration of ions released by both materials appeared not sufficient to inhibit bacterial growth. Moreover, the structural surface change and resultant increased surface roughness appeared to have promoted biofilm formation.
Significance
While having bioactive potential through ion release, the stability of surface integrity of bioactive materials is a key-parameter to be assessed with regard to their cariostatic potential.
1
Introduction
The continuous evolvement in dental adhesive technology, such as the development of novel resin-based composites (RBCs) and adhesives, has allowed the size of tooth preparations to be minimized. The dentist can now remove carious tissue selectively and fill the resultant cavity with adhesive materials. Concurrently, dental materials can today be bonded better to tooth tissue. However, secondary caries, which occurs after treatment of a primary caries lesion, could still not be ruled out . Several researchers have therefore investigated materials with antibacterial potential to prevent or treat dental caries . Silver compounds, such as silver nitrate, silver fluoride and silver diamine fluoride, have been used in restorative dentistry for many years . Also, silver nanoparticles recently appeared advantageous , as they have a large surface area and so exhibit a stronger antibacterial effect than does bulk or ionic silver. In addition, nanoparticles are widely applicable, because they can be mixed with conventional dental materials such as adhesives and RBCs . Other antibacterial substances, such as quaternary ammonium dimethacrylate monomers and 12-methacryloyloxydodecylpyridinium bromide, have been copolymerized in resin-based materials to render them antibacterial . Unfortunately, the actual cariostatic effect of bioactive restorative materials is often found to be limited. Also glass-ionomer cements (GICs) have been attributed antibacterial effects . They can release several types of ions; especially fluoride (F) is detrimental for bacteria . A low pH before setting also kills bacteria . Overall, also the ability of GICs to prevent secondary caries remains minor .
Few bioactive glasses have been suggested to release antibacterial ions . Bioactive glass has an amorphous structure, whereas glass–ceramics are crystallized glasses and composites of a crystalline phase in a residual glassy phase. Bioactive glass consists solely of the elements found in the body material, mainly being silicon (Si), calcium (Ca), sodium (Na), phosphorous (P), and oxygen (O) . Among the diverse kinds of bioactive glass filler, surface pre-reacted glass-ionomer (sPRG) filler has already been used for a relatively long time in some specific commercial RBCs. Most studies investigating this material assortment have focused on ion-release degree and potentially associated antibacterial effects .
However, only few studies have investigated the surface integrity of ion-releasing bioactive glass filler; the resultant surface receptiveness for bacterial adhesion has also scarcely been studied . Nevertheless, the restoration’s surface integrity may be a key factor determining the eventual clinical beneficial effect of the alleged antibacterial properties. Therefore, in order to evaluate the surface integrity of RBCs, containing bioactive glass filler, and its antibacterial efficacy, we compared in this study the bacterial growth and ion-release properties of a sPRG-filled RBC, as compared to that of a contemporary conventional RBC and GIC, this upon prior immersion in distilled water and lactic acid. The null hypotheses tested in this study were that (1) the surface integrity of the sPRG-filled RBC is stable, (2) the sPRG-filled RBC shows a stronger antibacterial effect than the conventional RBC and GIC, and (3) the sPRG-filled RBC releases more ions.
2
Materials and methods
2.1
Material disk preparation ( Fig. 1 )
The sPRG-filled RBC Beautifil ll (Shofu, Kyoto, Japan), the conventional RBC Herculite XRV Ultra (Kerr, Orange, CA, USA), and the conventional GIC Fuji IX GP EXTRA (GC, Tokyo, Japan) were used in this study. These materials were filled in a 10-mm (diameter) × 2-mm (height) silicone molds (LADD Research, Williston, VT, USA) and covered with a microscope slide-glass plate. In the case of RBC specimens, they were light-cured for 40 s from both sides (for a total curing time of 80 s) using a LED light-curing unit (G-Light Prima II Plus, GC). The GIC specimens were allowed to set for 5 min. The specimens were then polished using a 15-μm diamond lapping film (3 M, St. Paul, MN, USA). A total of 33 disks per material were prepared along with another set of 3 disks for the sPRG-filled RBC ( Fig. 1 ). After being polished, 3 disks were examined by Feg-SEM (see further) without any further treatment (control), while all other disks were either immersed in distilled water (pH 5.8) or in lactic acid (pH 4.0) for 3 days. After storage, the specimens were thoroughly washed with distilled water and air-dried.
2.2
Surface integrity examined by Feg-SEM
Three specimen surfaces per material (n = 3) were coated with a thin layer of osmium (Neo Osmium Coater, Meiwa, Tokyo, Japan), upon which the specimen surfaces were examined using field-emission-gun SEM (Feg-SEM; JSM-6701F, JEOL, Tokyo, Japan) operated at 5 kV and employing an annular semiconductor detector.
2.3
Bacterial strains and culture conditions
Streptococcus mutans (ATCC25175), stored at −80 °C, were subcultured on blood-agar plates (37 °C, 5% CO 2 ). Colonies from these blood-agar plates were cultivated overnight in brain heart infusion (BHI, Becton Dickinson and Company, Franklin Lakes, NJ, USA) broth; the obtained liquid cultures were used for the experiments.
2.4
Bacterial growth spectrophotometry
Bacterial growth was assessed by spectrophotometry. The liquid cultures were centrifuged and the bacteria were re-suspended in fresh BHI broth. The concentration was adjusted to 1 × 10 5 CFU/ml spectrophotometrically at a wavelength of 600 nm (BioSpectrometer Basic, Eppendorf, Hamburg, Germany). Three disks per material, which were stored for 3 days in distilled water or lactic acid, were placed in a 24-well plate (Costar 24 well, Corning, NY, USA), upon which 2 ml bacterial suspension was added. As positive and negative control, 3 wells did not receive a material disk and another three wells did not receive a bacterial suspension. The absorbance was measured for 30 h (6 h intervals) at 600 nm and 37 °C using a microplate reader (POLARstar Omega, BMG LABTECH, Ortenberg, Germany). This procedure was repeated three times (n = 3 sites on 3 disks). The bacterial growth data for each group were statistically compared using two-way analysis of variance (ANOVA) and Scheffé post hoc test with 5% error limits (p < 0.05) using IBM SPSS Statistics (version 19; IBM, Armonk, NY, USA).
2.5
Biofilm formation examined by Feg-SEM
Three disks per material, which were stored for 3 days in distilled water or lactic acid, were placed in a 24-well plate. Next, 2 ml of a S. mutans suspension in BHI broth, containing 1% sucrose, was added to each well. After incubation at 37 °C for 24 h, the adherent S. mutans on the disks was observed using Feg-SEM (Topcon DC-720, Topcon, Tokyo, Japan). This measurement was conducted in triplicate (n = 3 sites on 3 disks).
For cross-sectional observations, the abovementioned procedure was repeated using another set of 3 disks, after which the specimens were fixed using 2.5 wt% glutaraldehyde (Nacalai Tesque, Kyoto, Japan), stained with 2% osmium (TAAB Laboratories Equipment, Aldermaston, UK), and gradually dehydrated in ascending ethanol concentrations prior to being embedded in epoxy resin (TAAB Laboratories Equipment). Specimen cross-sections were made using a cross-section polisher (SM-090101 Cross Section Polisher, JEOL). Subsequently, a thin layer of osmium was deposited on their surfaces (Neo Osmium coater, Meiwa), upon which the specimens were examined using Feg-SEM (JSM-6701F, JEOL) operated at 5 kV and employing an annular semiconductor detector. The procedure was repeated three times (n = 3 sites on 3 disks).
2.6
HRTEM and STEM-EDS analyses of sPRG-filled RBC
High-resolution transmission electron microscopy (HRTEM) and scanning transmission electron microscopy (STEM)/energy-dispersive X-ray spectroscopy (EDS) were employed to determine the elemental distribution of the sPRG-filled RBC. Three disks (n = 3) were polished using an argon-ion-milling ion slicer (EM-09100IS, JEOL) prior to HRTEM and STEM-EDS analyses using a 200 kV TEM/STEM system (JEM-2100F, JEOL), which was equipped with a Cs-corrector probe (CEOS, Heidelberg, Germany). The system was operated at 200 kV with a current density of 40 pA/cm 2 . STEM bright-field (BF), low-angle annular dark-field (LAADF) and EDS (JED-2300T, JEOL) spectrometer attachments were used. The probe forming Cs correction allowed for STEM imaging with sub-Å resolution (minimum probe size: 0.09 nm). For EDS, an electron spot with a diameter of 0.3 nm was used. Furthermore, drift correction was performed each minute in order to account for the drift that may occur at nanoscale during the acquisition of STEM-EDS multi-element mapping.
2.7
Ion release
Two sets of 3 disks per material, which were before stored in distilled water or lactic acid, were placed in 2 ml of distilled water for 24 h.
Material solution samples, originating from the first set of three material disks (n = 3), were subsequently collected for elemental analyses of boron (B) and strontium (Sr) using inductivity coupled plasma atomic emission spectroscopy (ICP-AES; VISTA Pro, SII, Chiba, Japan).
In order to measure fluoride release, another approach was needed, because ICP-AES cannot measure fluorine. Therefore, ultraviolet spectroscopy was employed, for which first a calibration curve needed to be prepared on basis of standard sodium-fluoride solutions with different concentrations. Therefore, 0, 1, 2, 3, 4, and 5 ml of a sodium-fluoride solution, containing 0.01 mg F − /ml (Kanto Chemical, Tokyo, Japan), were added to 50-ml volumetric flasks. Next, 5.0 ml of 5% Alfusone (Dojindo, Tokyo, Japan) and 20 ml acetone were added to each flask, after which the flasks were further filled with distilled water to reach a total volume of 50 ml. These solutions were left to stand for 1 h at ambient temperature in order to subsequently characterize them at a wavelength of 620 nm using ultraviolet spectroscopy (BioSpectrometer Basic, Eppendorf) following the method decribed by Noguchi et al. . In order to measure fluoride release from the material disks, 2 ml of the respective material solution samples, originating from the second set of material disks, was added to 50-ml volumetric flasks, after which 5.0 ml of 5% Alfusone and 20 ml acetone were added, and each flask was finally filled with distilled water to reach a total volume of 50 ml. These experimental solutions were subjected to ultraviolet spectroscopy, as was mentioned above to determine the calibration curve. Three independent measurements were performed (n = 3).
2.8
Antibacterial efficacy
In order to determine the minimum concentration of B or F needed to inhibit bacterial growth, 100, 1000, 2500, 5000 and 10000 ppm boric acid (Sigma Aldrich, St. Louis, MO, USA) and 10, 100, 250, 500 and 900 ppm sodium fluoride (Sigma Aldrich) solutions were prepared. Next, 200 μl of each solution was placed in a 24-well plate, to which 1.0 × 10 5 CFU/ml S. mutans in BHI was added. The plate was then incubated for 24 h. The optical density of each well was measured using a plate reader (POLARstar Omega, BMG LABTECH) as measure of bacterial growth. Three independent measurements were performed (n = 3). The bacterial growth data for each group were statistically compared using one-way analysis of variance (ANOVA) and Scheffé post hoc test with 5% error limits (p < 0.05) using IBM SPSS Statistics (version 19; IBM, Armonk, NY, USA).
2
Materials and methods
2.1
Material disk preparation ( Fig. 1 )
The sPRG-filled RBC Beautifil ll (Shofu, Kyoto, Japan), the conventional RBC Herculite XRV Ultra (Kerr, Orange, CA, USA), and the conventional GIC Fuji IX GP EXTRA (GC, Tokyo, Japan) were used in this study. These materials were filled in a 10-mm (diameter) × 2-mm (height) silicone molds (LADD Research, Williston, VT, USA) and covered with a microscope slide-glass plate. In the case of RBC specimens, they were light-cured for 40 s from both sides (for a total curing time of 80 s) using a LED light-curing unit (G-Light Prima II Plus, GC). The GIC specimens were allowed to set for 5 min. The specimens were then polished using a 15-μm diamond lapping film (3 M, St. Paul, MN, USA). A total of 33 disks per material were prepared along with another set of 3 disks for the sPRG-filled RBC ( Fig. 1 ). After being polished, 3 disks were examined by Feg-SEM (see further) without any further treatment (control), while all other disks were either immersed in distilled water (pH 5.8) or in lactic acid (pH 4.0) for 3 days. After storage, the specimens were thoroughly washed with distilled water and air-dried.
2.2
Surface integrity examined by Feg-SEM
Three specimen surfaces per material (n = 3) were coated with a thin layer of osmium (Neo Osmium Coater, Meiwa, Tokyo, Japan), upon which the specimen surfaces were examined using field-emission-gun SEM (Feg-SEM; JSM-6701F, JEOL, Tokyo, Japan) operated at 5 kV and employing an annular semiconductor detector.
2.3
Bacterial strains and culture conditions
Streptococcus mutans (ATCC25175), stored at −80 °C, were subcultured on blood-agar plates (37 °C, 5% CO 2 ). Colonies from these blood-agar plates were cultivated overnight in brain heart infusion (BHI, Becton Dickinson and Company, Franklin Lakes, NJ, USA) broth; the obtained liquid cultures were used for the experiments.
2.4
Bacterial growth spectrophotometry
Bacterial growth was assessed by spectrophotometry. The liquid cultures were centrifuged and the bacteria were re-suspended in fresh BHI broth. The concentration was adjusted to 1 × 10 5 CFU/ml spectrophotometrically at a wavelength of 600 nm (BioSpectrometer Basic, Eppendorf, Hamburg, Germany). Three disks per material, which were stored for 3 days in distilled water or lactic acid, were placed in a 24-well plate (Costar 24 well, Corning, NY, USA), upon which 2 ml bacterial suspension was added. As positive and negative control, 3 wells did not receive a material disk and another three wells did not receive a bacterial suspension. The absorbance was measured for 30 h (6 h intervals) at 600 nm and 37 °C using a microplate reader (POLARstar Omega, BMG LABTECH, Ortenberg, Germany). This procedure was repeated three times (n = 3 sites on 3 disks). The bacterial growth data for each group were statistically compared using two-way analysis of variance (ANOVA) and Scheffé post hoc test with 5% error limits (p < 0.05) using IBM SPSS Statistics (version 19; IBM, Armonk, NY, USA).
2.5
Biofilm formation examined by Feg-SEM
Three disks per material, which were stored for 3 days in distilled water or lactic acid, were placed in a 24-well plate. Next, 2 ml of a S. mutans suspension in BHI broth, containing 1% sucrose, was added to each well. After incubation at 37 °C for 24 h, the adherent S. mutans on the disks was observed using Feg-SEM (Topcon DC-720, Topcon, Tokyo, Japan). This measurement was conducted in triplicate (n = 3 sites on 3 disks).
For cross-sectional observations, the abovementioned procedure was repeated using another set of 3 disks, after which the specimens were fixed using 2.5 wt% glutaraldehyde (Nacalai Tesque, Kyoto, Japan), stained with 2% osmium (TAAB Laboratories Equipment, Aldermaston, UK), and gradually dehydrated in ascending ethanol concentrations prior to being embedded in epoxy resin (TAAB Laboratories Equipment). Specimen cross-sections were made using a cross-section polisher (SM-090101 Cross Section Polisher, JEOL). Subsequently, a thin layer of osmium was deposited on their surfaces (Neo Osmium coater, Meiwa), upon which the specimens were examined using Feg-SEM (JSM-6701F, JEOL) operated at 5 kV and employing an annular semiconductor detector. The procedure was repeated three times (n = 3 sites on 3 disks).
2.6
HRTEM and STEM-EDS analyses of sPRG-filled RBC
High-resolution transmission electron microscopy (HRTEM) and scanning transmission electron microscopy (STEM)/energy-dispersive X-ray spectroscopy (EDS) were employed to determine the elemental distribution of the sPRG-filled RBC. Three disks (n = 3) were polished using an argon-ion-milling ion slicer (EM-09100IS, JEOL) prior to HRTEM and STEM-EDS analyses using a 200 kV TEM/STEM system (JEM-2100F, JEOL), which was equipped with a Cs-corrector probe (CEOS, Heidelberg, Germany). The system was operated at 200 kV with a current density of 40 pA/cm 2 . STEM bright-field (BF), low-angle annular dark-field (LAADF) and EDS (JED-2300T, JEOL) spectrometer attachments were used. The probe forming Cs correction allowed for STEM imaging with sub-Å resolution (minimum probe size: 0.09 nm). For EDS, an electron spot with a diameter of 0.3 nm was used. Furthermore, drift correction was performed each minute in order to account for the drift that may occur at nanoscale during the acquisition of STEM-EDS multi-element mapping.
2.7
Ion release
Two sets of 3 disks per material, which were before stored in distilled water or lactic acid, were placed in 2 ml of distilled water for 24 h.
Material solution samples, originating from the first set of three material disks (n = 3), were subsequently collected for elemental analyses of boron (B) and strontium (Sr) using inductivity coupled plasma atomic emission spectroscopy (ICP-AES; VISTA Pro, SII, Chiba, Japan).
In order to measure fluoride release, another approach was needed, because ICP-AES cannot measure fluorine. Therefore, ultraviolet spectroscopy was employed, for which first a calibration curve needed to be prepared on basis of standard sodium-fluoride solutions with different concentrations. Therefore, 0, 1, 2, 3, 4, and 5 ml of a sodium-fluoride solution, containing 0.01 mg F − /ml (Kanto Chemical, Tokyo, Japan), were added to 50-ml volumetric flasks. Next, 5.0 ml of 5% Alfusone (Dojindo, Tokyo, Japan) and 20 ml acetone were added to each flask, after which the flasks were further filled with distilled water to reach a total volume of 50 ml. These solutions were left to stand for 1 h at ambient temperature in order to subsequently characterize them at a wavelength of 620 nm using ultraviolet spectroscopy (BioSpectrometer Basic, Eppendorf) following the method decribed by Noguchi et al. . In order to measure fluoride release from the material disks, 2 ml of the respective material solution samples, originating from the second set of material disks, was added to 50-ml volumetric flasks, after which 5.0 ml of 5% Alfusone and 20 ml acetone were added, and each flask was finally filled with distilled water to reach a total volume of 50 ml. These experimental solutions were subjected to ultraviolet spectroscopy, as was mentioned above to determine the calibration curve. Three independent measurements were performed (n = 3).
2.8
Antibacterial efficacy
In order to determine the minimum concentration of B or F needed to inhibit bacterial growth, 100, 1000, 2500, 5000 and 10000 ppm boric acid (Sigma Aldrich, St. Louis, MO, USA) and 10, 100, 250, 500 and 900 ppm sodium fluoride (Sigma Aldrich) solutions were prepared. Next, 200 μl of each solution was placed in a 24-well plate, to which 1.0 × 10 5 CFU/ml S. mutans in BHI was added. The plate was then incubated for 24 h. The optical density of each well was measured using a plate reader (POLARstar Omega, BMG LABTECH) as measure of bacterial growth. Three independent measurements were performed (n = 3). The bacterial growth data for each group were statistically compared using one-way analysis of variance (ANOVA) and Scheffé post hoc test with 5% error limits (p < 0.05) using IBM SPSS Statistics (version 19; IBM, Armonk, NY, USA).
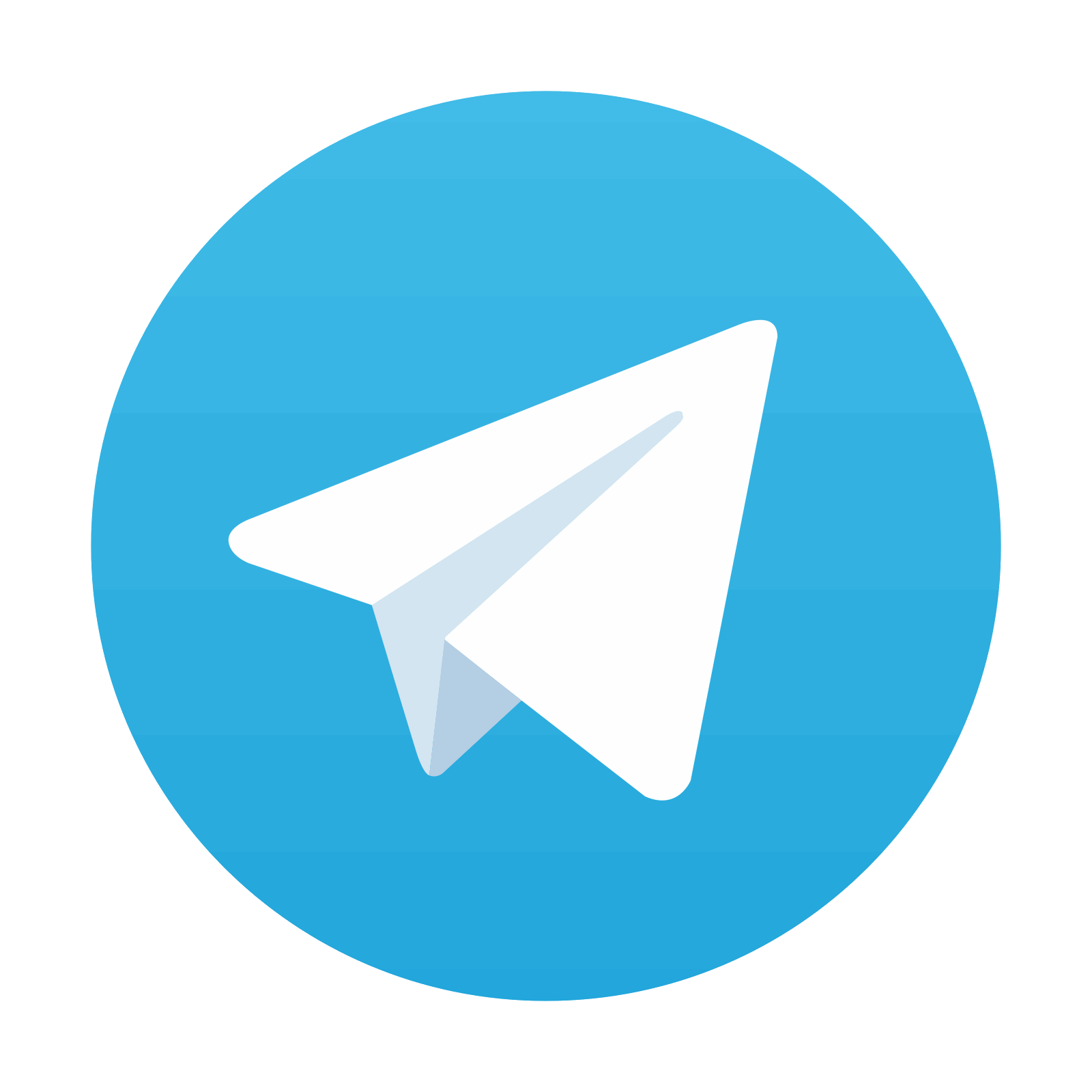
Stay updated, free dental videos. Join our Telegram channel

VIDEdental - Online dental courses
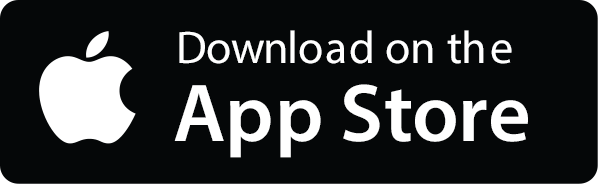
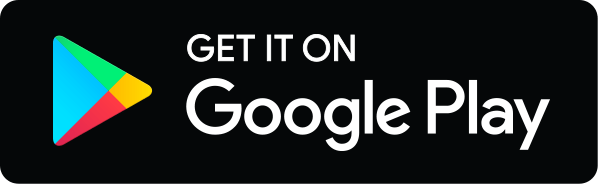