Highlights
- •
A novel technique for the encapsulation of plant-derived extracts was developed.
- •
Polynuclear microcapsules with core loading of up to 38 wt% extract were manufactured.
- •
Bioactivity of extracts was preserved after encapsulation for one year.
Abstract
Objective
To sustain the bioactivity of proanthocyanidins-rich plant-derived extracts via encapsulation within biodegradable polymer microcapsules.
Methods
Polylactide microcapsules containing grape seed extract (GSE) were manufactured using a combination of double emulsion and solvent evaporation techniques. Microcapsule morphology, size distribution, and cross-section were examined via scanning electron microscopy. UV–vis measurements were carried out to evaluate the core loading and encapsulation efficiency of microcapsules. The bioactivity of extracts was evaluated after extraction from capsules via solvent partitioning one week or one year post-encapsulation process. Fifteen human molars were cut into 7 mm × 1.7 mm × 0.5 mm thick mid-coronal dentin beams, demineralized, and treated with either encapsulated GSE, pristine GSE, or left untreated. The elastic modulus of dentin specimens was measured based on three-point bending experiments as an indirect assessment of the bioactivity of grape seed extracts. The effects of the encapsulation process and storage time on the bioactivity of extracts were analyzed.
Results
Polynuclear microcapsules with average diameter of 1.38 μm and core loading of up to 38 wt% were successfully manufactured. There were no statistically significant differences in the mean fold increase of elastic modulus values among the samples treated with encapsulated or pristine GSE (p = 0.333), or the storage time (one week versus one year storage at room temperature, p = 0.967).
Significance
Polynuclear microcapsules containing proanthocyanidins-rich plant-derived extracts were prepared. The bioactivity of extracts was preserved after microencapsulation.
1
Introduction
Dental caries is the most prevalent chronic disease worldwide. Resin composites have been widely used as restorative materials to manage tissue loss due to caries, yet, their service life is limited to only a few years (e.g. average 7 years in posterior teeth) .
The predominant reason for the replacement of composite restorations is the gradual loss of adhesion at the dentin–resin interface, likely due to the enzymatic degradation of collagen fibrils , and the hydrolytic degradation of resin components at the interface . There are two ongoing efforts to improve the long-term stability of the interface materials, and hence, the longevity of composite restorations: advancements in resin composite chemistry for increased stability in the oral environment, and strengthening and stabilization of dentin matrix at the dentin–resin interface. The latter can be achieved by an increase in the number of inter- and intra-microfibrillar cross-links within type I collagen fibrils through the use of exogenous natural and synthetic cross-linkers . The use of plant-derived extracts as collagen cross-linkers is more attractive and promising due to their biocompatibility and wide source availability of these compounds compared to their synthetic counterparts .
Recently, the use of plant-derived extracts rich in proanthocyanidins (PA), such as cocoa, pine bark, and grape seed extracts has been reported to effectively improve the mechanical properties and biostability of the dentin matrix as well as the dentin–resin bond strength . Simple addition of bioactives into the dental adhesive may prove challenging due to stability of the polyphenols and reactivity with the dental resin chemistry. On-demand release of bioactives at the adhesive interface is an attractive way to deliver and sustain activity over the long-term, or to localize activity where potential flaws are present at the interface.
Herein, we propose an approach for sustaining the long-term bioactivity of natural extracts and protection from potential environmental degradation via sequestration within polymer microcapsules. Microencapsulation is a versatile technique commonly used for isolation, protection, and controlled delivery of active core materials in a wide range of applications and industries including drug delivery , self-healing materials , nutrient preservation , and perfumery . The versatility of microcapsules is exemplified by the ability to release their core material in response to a variety of environmental stimuli including chemical triggers (e.g. change in pH), light irradiation, mechanical rupture, temperature, and time . For dental materials microencapsulation of bioactive or reactive agents has enabled strategies for enhancing the performance of dental tissues, materials, or treatments. For example, self-healing resin composites were demonstrated using core–shell microcapsules that release a healing agent in response to mechanical damage . Microcapsules have also been used for the time-release (also known as sustained release) of useful agents for antimicrobial activities , remineralization , and regeneration . Time-release is generally achieved via the degradation of the encapsulating wall material, diffusion through the shell wall, or a combination of these two mechanisms . Microencapsulation has also been used to enhance the stability of resinous components prior to clinical treatments via the sequestration of the reactive and sensitive components (e.g. polymerization initiators), and releasing them when required for optimal polymerization reactivity .
In this study, grape seed extract (GSE) was used as a core material for microencapsulation because of its high PA content (up to 97%, ) and proven biomodification of the dentin matrix. Polylactide (PLA), a biodegradable and biocompatible polymer, was used as the shell wall material since the encapsulation by PLA does not involve in-situ chemical reactions which could adversely affect the bioactivity of natural extracts, and the biodegradation of PLA offers the ability to control and tailor the sustained release of core materials for optimal delivery to the dentin matrix. The goals of this study were to develop a facile microencapsulation technique for the encapsulation of natural extracts (e.g. GSE) and to demonstrate the sustained bioactivity of the core material after the encapsulation process. Microcapsules containing natural extracts are ultimately envisioned to be embedded in the adhesive dental resin that anchors the bulk composite to the dentin substrate. We hypothesize that the biodegradation of the microcapsule shell wall will enable the sustained delivery of bioactives to the dentin matrix for continued stabilization of collagen fibrils, and consequently, prolonged service life of dental restorations. Consequently, the size of microcapsules is constrained by the maximum thickness of the adhesive layer (ca. 20 μm) for practical clinical applications.
A novel double emulsion-solvent evaporation microencapsulation technique was used to encapsulate GSE within PLA microcapsules. Encapsulation parameters were optimized to produce microcapsules with acceptable morphology, desired size range, and high core loading. Bioactivity of GSE, both pre- and post-encapsulation, was assessed by the pretreatment of demineralized dentin specimens with GSE and the measurement of elastic modulus from three-point bend experiments.
2
Materials and methods
2.1
Materials
Unless otherwise specified, all chemicals and reagents were purchased from Sigma Aldrich and used as received. Polylactide (PLA) pellets were purchased from NatureWorks, LLC (Ingeo 4043D, M n ∼100,000 Da, and M w ∼150,000 Da). Grape seed extract (GSE) was 95% proanthocyanidins-rich Vitis vinifera (MegaNatural™ gold grape seed extract, California, USA).
2.2
Preparation of microcapsules
Microcapsules were fabricated using a combination of water–oil–water (W/O/W) double emulsion and solvent evaporation techniques ( Fig. 1 ). In a typical experiment 600 mg GSE was dissolved in 3 mL of distilled water to produce the inner water solution (W i ). The middle oil solution (O) comprised 20 g of 1.5 wt% Span 85 oil-soluble surfactant and 3 wt% PLA in dichloromethane (DCM), a volatile, water-immiscible organic solvent. The outer aqueous solution (W o ) was 300 mL of 2.5 wt% polyethylene glycol (PEG) and 1 wt% sodium dodecyl sulfate (SDS) surfactants in distilled water. The aqueous surfactant mixture was selected from the experimental trials of various surfactant mixtures in order to obtain microcapsules with good surface morphology and size control.
The first emulsion (i.e. W i /O) was prepared by adding W i solution dropwise to the oil solution in a 50 mL beaker under continuous agitation at 1500 rpm and simultaneous sonication (tapered 3 mm tip sonication horn in 750 W ultrasonic homogenizer, Cole Parmer) for 10 min (20% amplitude, 0.2 s pulse, 0.2 s pause). The beaker was kept in an ice bath to minimize the evaporation of DCM during emulsification. The prepared emulsion was then, in turn, emulsified into the W o solution in a 600 mL beaker using a homogenizer (OMNI GLH-01) for three minutes. After forming a stable W i /O/W o double emulsion, the emulsion was agitated at 800 rpm for five hours to allow the gradual diffusion and evaporation of DCM solvent. Removal of DCM from the middle layer resulted in the consolidation of PLA polymer and the formation of a protective shell wall around the aqueous core (W i ). Microcapsules were then centrifuged (4000 rpm for 10 min) and washed with distilled water four times to remove any residual surfactants. Finally, collected microcapsules were lyophilized for 24 h to remove water from the microcapsules.
2.3
Characterization of microcapsules
Microcapsule morphology, cross-sectional imaging, and size distribution were characterized via scanning electron microscopy (SEM, Quanta 450 FEG ESEM). For cross-sectional imaging, microcapsules were embedded in an epoxy resin, and cured for 24 h at 30 °C. Specimens were then immersed in liquid nitrogen for a few minutes and then immediately fractured using a razor blade. Before imaging, specimens were sputter-coated with Au/Pd to avoid surface charging.
UV–visible spectroscopy (UV-2401 PC, Shimadzu, Japan) measurements were performed to determine the microcapsules core loading as well as the encapsulation efficiency. A 1 g/L solution of microcapsules in a mixture of 60 vol% tetrahydrofuran (THF) and 40 vol% methanol was prepared, and diluted with distilled water at a 1:9 volume ratio to force the polymer precipitate out of the solution. A linear calibration curve of absorbance (at 280 nm) as a function of GSE concentration in the same solvent mixture was also created by measuring the absorbance of GSE (200–700 nm) at known concentrations. The absorbance of GSE in the microcapsule solution was then measured, and GSE concentration was determined from the calibration curve. Microcapsules core loading, L GSE , was calculated as,
L G S E = c G S E V s d M c a p × 100
where c GSE is the concentration of GSE determined from calibration curve, V s is the volume of diluted solution used in the UV–vis measurement, d is the dilution ratio (equal to 10 in this study), and M cap is the original mass of microcapsules dissolved in the solution. Encapsulation efficiency, ξ, of the microencapsulation procedure was calculated by comparing the actual loading of microcapsules with the theoretical loading of GSE,
ξ = L G S E L G S E th × 100
and,
L G S E th = M G S E M G S E + M p × 100
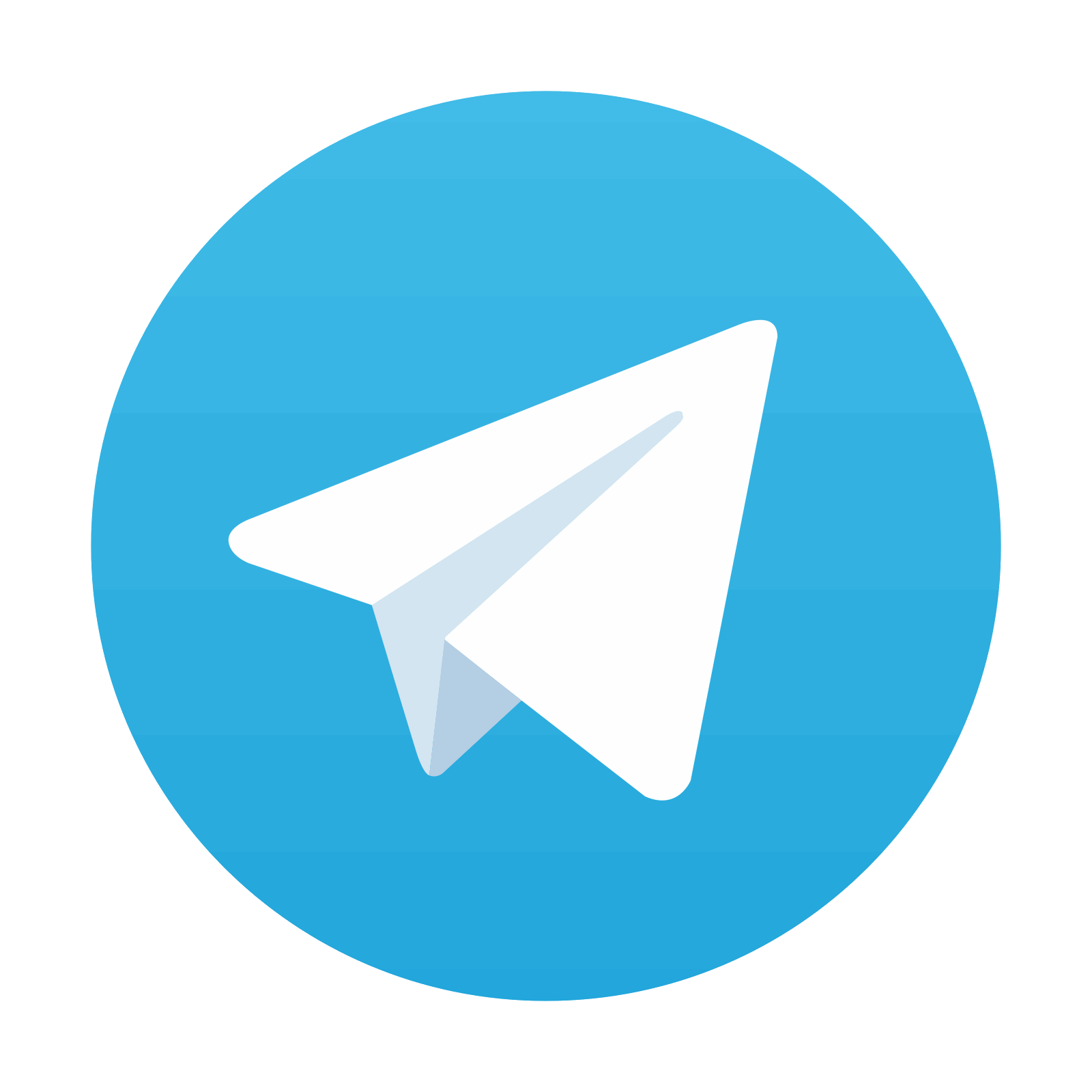
Stay updated, free dental videos. Join our Telegram channel

VIDEdental - Online dental courses
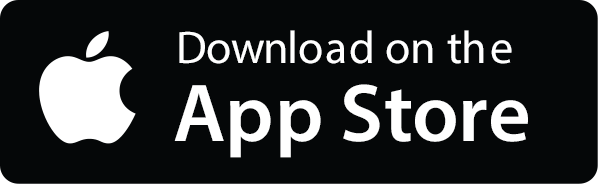
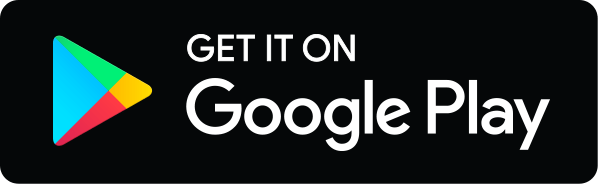