Abstract
Objective
The dynamic bone-periodontal ligament (PDL)-tooth fibrous joint consists of two adaptive functionally graded interfaces (FGI), the PDL-bone and PDL-cementum that respond to mechanical strain transmitted during mastication. In general, from a materials and mechanics perspective, FGI prevent catastrophic failure during prolonged cyclic loading. This review is a discourse of results gathered from literature to illustrate the dynamic adaptive nature of the fibrous joint in response to physiologic and pathologic simulated functions, and experimental tooth movement.
Methods
Historically, studies have investigated soft to hard tissue transitions through analytical techniques that provided insights into structural, biochemical, and mechanical characterization methods. Experimental approaches included two dimensional to three dimensional advanced in situ imaging and analytical techniques. These techniques allowed mapping and correlation of deformations to physicochemical and mechanobiological changes within volumes of the complex subjected to concentric and eccentric loading regimes respectively.
Results
Tooth movement is facilitated by mechanobiological activity at the interfaces of the fibrous joint and generates elastic discontinuities at these interfaces in response to eccentric loading. Both concentric and eccentric loads mediated cellular responses to strains, and prompted self-regulating mineral forming and resorbing zones that in turn altered the functional space of the joint.
Significance
A multiscale biomechanics and mechanobiology approach is important for correlating joint function to tissue-level strain-adaptive properties with overall effects on joint form as related to physiologic and pathologic functions. Elucidating the shift in localization of biomolecules specifically at interfaces during development, function, and therapeutic loading of the joint is critical for developing “functional regeneration and adaptation” strategies with an emphasis on restoring physiologic joint function.
1
Introduction
The bone-periodontal ligament (PDL)-tooth complex of the oral and craniofacial masticatory complex is a dynamic and biomechanically active fibrous joint . The primary function of the joint is to sustain cyclic chewing forces of varying magnitudes and frequencies. It is categorized as a fibrous joint by virtue of the PDL that (1) serves to attach teeth to the alveolar bone , a type of bone that is distinctly different from skeletal bone; (2) serves to consequently facilitate tooth displacement within the alveolar bony socket; (3) serves to distribute and dampen masticatory forces through the vascularized and innervated PDL; (4) contains differentiating zones at the ligament-cementum and ligament-bone entheses (attachment sites) ; (5) adjoins and interacts with cementum and alveolar bone through ligament-cementum and ligament-bone interfaces; (6) sustains and permits load-based reactionary forces from the tissues (enamel, dentin, cementum) and interfaces (enamel-dentin and cementum-dentin junctions) that makeup teeth exclusively; and (7) subsequently induce mechanical strain not limited to alveolar bone . A multitude of structural components and tissues (categorized as biomaterials) join to form this nature’s well-lubricated load-bearing joint. Conceivably, this fibrous joint undergoes mechanical strain-mediated adaptation where the measured physical and chemical properties of load-bearing tissues per se and their interfaces are appreciated in the context of overall function. Understanding how tooth motion is guided within the alveolar socket in the presence of the PDL, and how the attachment process accommodates cyclic functional loads is critical. This would allow extracting strain-adaptive information that promotes tissue regeneration/remodeling to maintain biomechanical function of a joint.
To date, the biomechanical aspects of the bone-PDL-tooth fibrous joint have been investigated at discrete length-scales, that is, at the levels of the joint , tissues , and cells . Investigations at a joint level have provided insights into the “coupled” nature of joint form and its masticatory function . At a tissue level, biological processes identified as interactions between the soft organic meshwork of the PDL and adjoining hard bone and cementum matrices, and subsequent strain-mediated mineralization of the inorganic hard tissue through active modeling and remodeling , have provided insights into joint adaptation in response to physiologic and pathologic forces . Through a reductionist approach, at a tissue and cellular-level, immunohistological approaches led to mapping of cell behavior and related matrix protein expressions to perturbations placed on tissues and cultured scaffolds . This also implies that cell and related tissue mechanics are seldom evaluated within the context of organ function. While probing using reductionist approach answers questions specific to tissues and cells respectively, it minimally addresses the importance of the measured physical and chemical properties, and thereby biological processes within the realm of function. In this manuscript, insights into plasticity and thereby adaptive features within a complex in the context of function will be extracted using principles from biomechanics and mechanobiology. As a result, the manuscript will also highlight an interdisciplinary yet holistic approach (as opposed to reductionist) through the use of various imaging modalities to detail the effect of form and function relationship on strain adaptive properties of human alveolar bone, the PDL, cementum, and PDL-bone and PDL-cementum interfaces. Additionally, results will be discussed in the context of clinically relevant problems specifically related to orthodontics.
The two central objectives to highlight the critical importance of regenerative capacity of PDL-bone and PDL-cementum interfaces within the context of multi-scale biomechanics of the bone-PDL-tooth fibrous joint will be as follows. Within this objective the following salient points will be discussed.
- 1.
The changes in form of a human tooth relative to the socket-shape and vice versa can prompt local adaptations through strain concentrations within the PDL, PDL-bone and PDL-cementum interfaces that facilitate the dynamic function of the bone-PDL-tooth fibrous joint .
- a
The structural integration in tandem with the gradual variation in physical and chemical characteristics at the PDL-bone and PDL-cementum interfaces exist within a bone-PDL-cementum complex .
- b
Deviation from normal PDL-space (150–380 μm) to adapted regions of (5–50 μm) at the original ligament-bone and ligament-cementum attachment are to resist functional demands .
- c
Over time, these constriction sites within the PDL-space are new load-bearing sites and could eventually impair joint function .
- a
- 2.
In vivo models can be used to manipulate and help understand the adaptive capacities of entheses/interfaces. “Natural intelligence” of interfaces can be determined by extracting mechanoresponsive signals from cells within local regions specifically within the context of a clinical setting such as orthodontic tooth movement (OTM).
- d
The semiautonomous events at interfaces guide the joint within physiologic limits, but significant mineral formation and resorption at the PDL-interfaces may result in closing or widening of the PDL-space and can guide the complex to a nonphysiologic regime .
- d
In all cases, insights into the functional information for the PDL-bone and PDL-cementum interfaces will be extracted from in situ experiments , and mapping of physical and chemical characteristics of interfaces will be performed through high resolution complementary and correlative microscopy techniques.
- 1.
The changes in form of a human tooth relative to the socket-shape and viceversa can prompt local adaptations through strain concentrations within the PDL, PDL-bone and PDL-cementum interfaces that facilitate the dynamic nature of the bone-PDL-tooth fibrous joint function .
The mammalian tooth attachment is a part and parcel of the oral and craniofacial masticatory complex, possesses the unique characteristic of short range motion compared to long range motion observed in diarthrodial joints of the musculoskeletal system. Such short-range motion is provided by a soft, fibrous PDL that attaches cementum to the alveolar bone, and acts as a shock absorber to distribute occlusal loads during mastication . Absorption of functional loads decreases hard tooth-tooth impact and enamel wear, alleviates stress/strain concentrations across PDL-cementum, PDL-bone attachment sites and interfaces, and allows for decades of cyclical loading . Tooth movement due to chewing within physiological limits drives joint function through maintenance of the functional PDL-space via mechanobiological processes . The goal of this section is to elucidate tooth movement within the alveolar socket and to gain insights into the contribution and adaptive capacity of the constitutive properties of soft/hard tissues and interfaces to maintain joint biomechanics. Experimental approaches included a validated holistic method using noninvasive imaging technique through micro-X-ray computed tomography (μ-XCT) coupled to a mechanical loading device . Ex vivo experimentation enabled visualization and evaluation of the effect of physical shifts within the intact complex of a loaded fibrous joint .
1.1
Entheses, Entheses Organs and Interfaces: Materials Science, Biomechanics, and Mechanobiology Perspectives
Similar to other diarthrodial joints in the body, the load-bearing bone-PDL-tooth fibrous joint has the ability to react and sustain cyclic load through self-regulated biological processes. Under loaded conditions, mechanical strains can stimulate cells at various sites of the complex ( Fig. 1 a and b), and promote strain-adaptive properties within periodontal tissues and their interfaces ( Fig. 1 c and d). From a materials perspective, the strain-adaptive properties are termed as physicochemical properties.

Functional aspects related to interfaces within the context of biomechanics and mechanobiology were eloquently stated by Darcy Thompson and were adapted to fit within manuscript’s content. Thompson wrote “…and the beauty and strength of the mechanical construction (joint) lie not in one part or in another (tissues per se), but in the harmonious concatenation (interfaces) which all the parts, soft and hard, rigid and flexible, tension-bearing and pressure-bearing, make up together .” An interface is a “concatenation” of dissimilar materials. Similar to materials science, various analytical techniques have taught that a natural interface can be converted to a homophone; interphase. An interphase is a region that encapsulates changes in physical and chemical characteristics that permit transition of one material to another (soft to hard ( vice versa ), hard to hard, soft to soft – all of which are dissimilar tissues) in a seamless fashion. This implies that an interphase is a transition zone and challenges identification of the end and beginning of two dissimilar tissues.
In biology, a term analogous to an interface is an enthesis organ. This term was first used in the musculoskeletal system to define interfaces between dissimilar tissues such as bone and tendon (osteotendinous), or bone and ligament (osteoligamentous) . There is yet another term known as the “junction”. However, when used in the context of an interface, it is limited in meaning as it alludes to a physical demarcation between two different materials/tissues as seen by the naked eye, or limited to a low resolution light microscope. Several such junctions known as the dentin-enamel, cementum-dentin, and cementum-enamel junctions were also observed . From mechanics and materials science perspectives, the topic of particular interest to researchers is “fracture toughness” of a seemingly discontinuous system, that is, that which can be defined as an abrupt change from one material to another. However, interdisciplinary approaches using analytical techniques and histological analyses indicated natural interfaces to consist of gradients. Gradients with gradual changes in structure, elemental and molecular compositions, and elastic modulus within a finite width exist between dissimilar tissues including the cementum-dentin, cementum-enamel, and enamel-dentin regions . In a similar fashion, fibrocartilaginous joints showed that as tendons inserted into bone, they transitioned through zones containing gradual gradients in organic and inorganic concentrations interspersed with hygroscopic regions ( Fig. 1 ). Consequently, it is thought that these gradual transitions in elemental and biochemical compositions provide resistance to functional demands including fracture . The importance of a gradual transition permitted by multiple zones is highlighted specifically when it is contrasted with a single zone. Single zone transition/gradient is indicated by a sharp increase or decrease in organic to inorganic ratio . These single zone regions are observed as elastic discontinuities within load-bearing systems with impaired function ( Fig. 2 ) .
- a
The structural integration in tandem with the gradual variation in physical and chemical characteristics at the PDL-bone and PDL-cementum interfaces exist within a bone-PDL-cementum complex ( Fig. 1 ) .

Interfaces continue to be characterized to bioengineer templates upon which tissues can be regenerated to replace injured and/or chronically inflamed regions that impair locomotion. Within joints, interfaces are localized regions and act as “functional machines” due to crosstalk between a multitude of cells and cell-extracellular matrix structural components that work in concert to accommodate functional forces and permit joint motion . Within musculoskeletal and oral and craniofacial systems, these interfaces are grossly categorized as (1) indirect fibrocartilaginous, in which tendons ligaments insert into bone through an intermediate tissue type such as cartilage, and (2) direct fibrous entheses, in which tendons/ligaments insert into bone and cementum (in case of a tooth) with no identifiable cartilaginous tissue . Studies guided by the principles from biomechanics and mechanobiology illustrated PDL-bone and PDL-cementum interfaces of the bone-tooth fibrous joint to express characteristics analogous to direct fibrous entheses. Regardless, applying principles of mechanics of materials, amplification of strains within the cells at the soft-hard tissue interfaces compared to adjacent bulk tissues per se renders the tethered ends of the softer tissues to be susceptible to increasing shear and flexural moments that are commonly identified in load-bearing joints with short and long-range motions. However, from a mechanics perspective to prevent catastrophic joint failure through fatigue, cells interspersed with increased concentrations of matrix molecules including the adhesive proteoglycans (PGs) at these tethered ends can promote cell migration and permit their adhesion and matrix interactions needed for tissue regeneration and remodeling .
PGs are hydrophilic macromolecules that are responsible for resisting the compressive forces through their water-retention characteristics, and sustaining functional loads in the periodontium . PGs are secreted by various cells in the PDL to sequester biomolecules and directly and indirectly modulate extracellular matrix formation. PGs have varied function in that their concentration and arrangement within the 5–10 μm region adjoining cementum and alveolar bone is distinctly different from that which is observed at the PDL-cementum and PDL-bone attachment sites ( Fig. 1 ). Functions of PG include regulating adhesion of fibroblasts, cementoblasts, and osteoblasts to adjacent tissues to repair both degraded tissues and the attachment mechanism, and acting as potential modulators of biominerals . Hence, it is conceivable that the inherent characteristics of the PG-decorated PDL-cementum and PDL-bone interfaces and partial swelling at the adjoining hypomineralized regions consisting of hygroscopic PDL-inserts are vital for tooth attachment and modulating physiological loads ( Fig. 1 c and d). These identifiable gradual transitions from softer to harder tissues (and vice versa ) are used as a standard “blue print” to engineer functionally graded interfaces (FGI).
The guiding principle of an FGI is to alleviate mechanical strains by distributing them over a wider interface thereby decreasing susceptibility to failure through tears and ruptures with an increased resistance to fracture. This signature of nature’s FGI has been consistently demonstrated across diverse species that include the squid beak, bamboo stem, and vascular bundle of the Washingtonia robusta palm to name a few. Within each of these species, gradients in mechanical stiffness have developed as an adaptive response in an attempt to reinforce underlying organ structure to resist the external stimulus. As such, interfaces can then be defined as a collection of related tissues at and near the attachment site (enthesis), and develop in response to environmental cues, such as shifts in mechanical strains. Consequently, the stimulated cells attempt to reinforce regions as they react to the biomechanical signals (mechanical strains related to tension, compression, and shear) that stem as function for any organism. The reinforcement can be identified as a multi-zone interface exhibiting a gradual transition, or a single-zone interface exhibiting an abrupt transition from soft to hard tissues and vice versa . These single zone regions are identified as elastic discontinuities within load-bearing systems and could perpetuate pathologic function ( Fig. 2 ).
- b
Deviation from measured normal PDL-space (150–380 μm) to adapted regions of (5–50 μm) at the original ligament-bone and ligament-cementum attachment are to resist functional demands ( Fig. 2 ) .
When external stimuli that modulate functional homeostasis are altered and include abnormal regimes, irreversible modeling occurs and physicochemical properties change until a new functional homeostasis within a joint is reached . Under these conditions, the question is; what is the functional relevance of these locally measured shifts in physicochemical gradients specifically at the PDL-bone and PDL-cementum and bone-PDL-cementum complex? That is, how does this shift in stiffness gradient affect overall joint function?
Under normal conditions, the graded stiffness from PDL to bone and PDL to cementum could vary due to natural physiological tooth movement with age known as active eruption, as well as by biomechanical function such as; masticatory forces due to normal and malocclusion. This normal adaptive role of the PDL-bone and PDL-cementum interfaces is amplified with the addition of external perturbations such as therapeutic load caused by orthodontic forces or disease as is the case with periodontitis. In support of the mechanics of materials related principle, a higher incidence of inflammation and failure in the form of tears at soft-hard tissue interfaces is commonly observed when PDL to bone and PDL to cementum interfaces are subjected to nonphysiologic loading. Due to the presence of cells, the increasingly strained interfaces become local regulators and form self-governing zones, and are ultimately observed as chronically inflamed sites. These injuries occur with repeated insults/nonphysiologic stimuli or high impact sports, exemplified by development of insertional tendinopathies at the elbow, the Achilles tendon, and the knee . Such injuries are also known as enthesopathies, gradually formed at the soft-hard tissue entheses organs/interfaces with continuous nonphysiologic cyclic loads which over time can impede joint mobility and cause joint impairment .
In a human bone-PDL-tooth fibrous joint, these elastic gradients at entheses were found to be caused by a gradual increase in inorganic to organic ratio as the softer PDL transits either into stiffer bone or cementum ( Fig. 1 ). Partial swelling at the hypomineralized regions within the attachment sites between PDL and bone or cementum could establish the interface width over which the mechanical properties vary from the lower PDL (10–50 MPa) to higher values observed in alveolar bone (0.2–9.6 GPa) and cementum (1.1–8.3 GPa). As a result, under normal function, such interfaces can protect against excessive shear, torsional, bending, rotational, tensile, and compressive forces accompanied with joint movement , ensuring optimum load distribution and transmission of cyclic loads ( i.e. chewing forces) that prevent wear and tear due to fatigue. However, the following questions are invoked; what would happen should this gradual change in stiffness undergo into an abrupt change as observed in Fig. 2 ? What would this shift in strain-adaptive properties (in this case the property in Fig. 2 is reduced elastic modulus) mean in the context of joint function? And under what external stimuli including clinical scenarios would such a shift in graded properties arise? These questions will be addressed in the following sections.
The adaptive nature of the PDL and its interfaces is thought to be due to vascular elements (blood vessels – BV, Fig. 2 ) and nerves that are continuous with the extracellular matrix of the PDL that consists of fibrous proteins, including the dominant type I collagen, and trace type III and V collagens, elastin and oxytalan fibers, and globular proteins, including proteoglycans and other noncollagenous proteins . Progenitor cells and their differentiated lineages of osteoblasts, fibroblasts, and cementoblasts, reside within the matrix, but are localized in strain-specific regions of the complex . The surface layer of alveolar bone adjacent to the PDL consists of an underlying osteoid matrix with maturing inorganic minerals in the predominant forms of hydroxyapatite and carboxyapatite . Typically, the general structure of an alveolar bone consists of an outer dense shell of compact bone known as the lamina dura to which a heavily vascularized, spongy cancellous bone is attached. The thickness of the compact bone as well as size and number of trabeculae in cancellous bone can increase and/or decrease in density in response to hyperfunction and hypofunction, respectively , and is controlled by magnitude and duration of loading. These remodeling aspects of bone occur through an orchestrated effort of osteoblasts of mesenchymal and osteoclasts of hematopoetic origins, creating reversal lines caused by deposition and resorption activities, respectively . Hence, from a functional perspective, one can ask, do these protrusions in bone ( Fig. 2 g) with a higher elastic modulus cause an elastic discontinuity in bone despite dominance in hygroscopic PDL-inserts? And does this discontinuity within the tissue shift joint biomechanics from physiologic to pathologic or maladapted function?
Alveolar bone along with its bundle bone protrusion works in concert with another mineralized tissue, cementum within a bone-PDL-tooth fibrous joint. Opposite to the PDL-bone attachment site of the complex, a mineralizing matrix known as the cementoid also exists at the PDL-cementum interface . Cementum is thought to be similar in structure to bone; however, it possesses an acellular zone in the anatomical coronal half to two-thirds of the tooth root . Deposition and resorption of mineral also occur in cementum as they do in bone; however, these changes are usually in the form of modeling as opposed to remodeling . Most often it is thought that cementum is lamellar, does not adapt, and has minimal adaptive response. While this could be true when compared to vascularized bone, results from our laboratory have indicated that cementum could have a delayed response. If so, could this be the reason why bone grows into the PDL-space ( Fig. 2 b, d and f), while cementum could subsequently make way to accommodate bone growth? Both these events need not be seen in the same sectioned two-dimensional (2D) plane, and indeed occur in three-dimensional (3D) volumes of the bone-PDL-cementum complex . And could this be the reason why secondary events such as conforming or nonconforming forms between the bony alveolar socket and cementum of the tooth can occur?
1.2
Physiologic and Pathologic Strain Amplifications at the PDL-bone and PDL-cementum Interfaces: Conforming and Nonconforming Alveolar Bone-Tooth Surfaces
In musculoskeletal and dental orthopedics, conforming and nonconforming surfaces can define the form-function behavior/adaptation of a joint, and provide insights into the local behavior at a tissue level and resulting cellular responses and subsequent genetic and molecular expression. These hierarchical length-scale events provide a continuum-like effect to address functional homeostasis of a joint. Based on the fundamental seminal concept by Julius Wolff, the long-term effect of functional aberrations at the macroscale could result in pathological deformations to maintain function. Over time pathological deformations of mineralized tissues change the internal architecture, as the joint continues to function. Extending this to bone-PDL-tooth fibrous joint, the relative motions between members through a combination of soft and hard tissues and their interfaces will continue to adapt tissues locally. This adaptation is guided by the principles of joint biomechanics intertwined with mechanobiology of tissues. Adaptation is identified by spatiotemporal changes in physical and chemical properties of soft and mineralized tissues including their interfaces which in turn can result in an overall change in a form-function relationship, commonly termed as functional or biomechanical adaptation.
This section will elucidate the effect of eccentric loads, loads which do not align with the anatomical axis of the joint as opposed to concentric loads. Eccentric loads are those imposed by parafunctions with varying magnitudes and frequencies in which the principle vector is converted into a moment, and can exacerbate functional adaptation between two nonconforming surfaces in motion, specifically at the tethered ends of the PDL-bone and PDL-cementum attachment sites. These adaptations over time can be observed as physical constrictions, and when investigated in the context of function can lead to stress concentrations and impending failure of the once biomechanically efficient joint. Other significant load-induced perturbations include traumatic and therapeutic loads from orthodontic braces all of which can change form-function relationship and decreased functional efficacy of the bone-PDL-tooth fibrous joint which will be discussed in subsection 2 .
- c
These constriction sites within the PDL-space over time are new load-bearing sites and could eventually impair joint function ( Fig. 2 ) .
Form modulates biomechanical response of individual structural components of the fibrous joint including its interfaces to facilitate optimal tooth movement under physiologic and nonphysiologic conditions, including therapeautic loads. The contextual information of interfacial biomechanics is best highlighted by performing in situ experiments through visualization of interfaces at no load and under loaded conditions. Following image registration/analyses, the deformation within the PDL as a result of concentric loading (vertical load aligned with the anatomical axis of the bone-PDL-tooth fibrous joint) was mapped as shown in Fig. 1 . A normal PDL-space of 150–380 μm as observed is mechanically strained under uniformly loaded conditions. However, in situ eccentric (off centered) loading of the joint illustrated that tilting of the root in the bony socket can occur and induce tissue adaptations, not only at the interfaces, but within periodontal tissues per se by converting strain-induced deformation within respective matrices (mechanical energy) into biological processes (chemical energy). This conversion predominantly occurs intracellularly, and is facilitated by matrix–cell, and cell–cell interactions . Specifically, mechanical strains can be localized in regions where nonconformity between the surfaces of the tooth and the alveolar socket is appreciated ( Fig. 1 a – red patches, b – block arrows and white arrows). At these regions it is likely that strains at the tendon/ligament-bone entheses could be up to four times the deformation experienced by the tendon/ligament bulk tissue, with microtears and/or microfractures occurring mainly within the interface . As a result, cells within tissues near entheses are subjected to high incidences of strain amplification, subsequently prompting wear and tear at a tissue level and causing degenerative diseases called enthesopathies .
Enthesopathies can occur as a result of disease and/or load-induced perturbations of higher frequencies and magnitudes of cyclic mechanical forces. Altered strain within the PDL-space simply due to load ( Fig. 1 a and b) and other aberrant inputs, such as bacterial insult, changes in food hardness, and therapeutic loads, can in turn shift the overall displacement of the tooth relative to the socket in response to load, thereby altering the self-regulating biomechanical cycle of the joint and triggering its adaptive nature in perpetuity ( Figs. 1–3 ). In other cases, proinflammatory factor expressions, cellular recruitment, and metaplasia at sites of entheses in response to excessive extraneous loads and/or prolonged inflammatory conditions have been shown to propagate the development of bony spurs that discourage joint mobility . Hence, prolonged perturbations in the form of excessive extraneous loads often cause chronic inflammatory diseases that could alter or break down tissues involved in constructing stress concentration-relieving entheses organs. The observed bony protrusions could occur due to nonphysiological functional demands at the tethered ends of the PDL-bone and PDL-cementum attachment sites, and strengthen the region to accommodate functional demands. This compromised PDL-space will continue upon further loading, as the constriction sites will become the new “load-bearing sites” that eventually cause direct local fusion of bone with cementum. Similarly, it is speculated that altering function of the fibrous joint, i.e. tooth movement, generates adaptation of tissue composition to address changes in biomechanics ( Fig. 2 – differences between normal and a compromised human bone-PDL-tooth complex). Clinically, while a narrowed PDL-space can be considered asymptomatic when superimposed with other aberrant loads and clinical interventions it can cause failure of the fibrous joint.
- 2
In vivo models can be used to manipulate and help understand the adaptive capacities of entheses/interfaces. “Natural intelligence” of interfaces can be determined by extracting mechanoresponsive signals from cells within local regions specifically within the context of a clinical setting such as orthodontic tooth movement (OTM) .
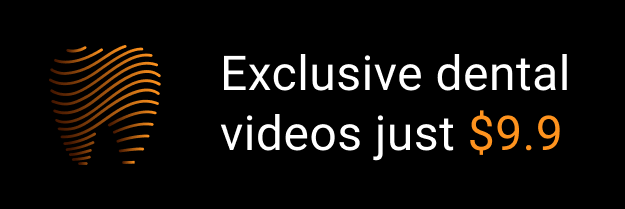