Introduction
Our objective was to assess the antibacterial and cytotoxic effects of orthodontic stainless steel brackets coated with different phases of photocatalytic titanium oxide.
Methods
From a total sample of 115 brackets, 68 orthodontic stainless steel brackets were coated with titanium oxide using a radiofrequency magnetron sputtering machine. The coated brackets were then converted into 34 each of the anatase and rutile phases of titanium oxide. These brackets were subdivided into 4 groups for antibacterial study and 3 groups for cytotoxicity study. Brackets for the antibacterial study were assessed against the Streptococcus mutans species using microbiologic tests. Three groups for the cytotoxicity study were assessed using the thiazolyl tetrazolium bromide assay.
Results
The antibacterial study showed that both phases were effective, but the rutile phase of photocatalytic titanium oxide had a greater bactericidal effect than did the anatase phase. The cytotoxicity study showed that the rutile phase had a greater decrease in viability of cells compared with the anatase phase.
Conclusions
It is recommended that orthodontic brackets be coated with the anatase phase of titanium oxide since they exhibited a significant antibacterial property and were only slightly cytotoxic.
Highlights
- •
Antibacterial and cytotoxic effects of titanium oxide (TiO 2 ) coating on orthodontic brackets were assessed.
- •
Anatase and rutile phases of photocatalytic TiO 2 were both effective.
- •
Rutile phase of photocatalytic TiO 2 showed more bactericidal effects than the anatase phase.
- •
Rutile phase showed a greater cytotoxic effect than the anatase phase.
- •
Anatase phase of TiO 2 has an antibacterial property and is less cytotoxic.
The oral cavity sustains and provides a habitat for all the complex microbiota, creating an ideal environment for their growth. A disease-free environment is maintained when there is a state of balance between the microorganisms and their substrates. The state of balance may be affected when there is any change in the oral environment. The introduction of orthodontic appliances may bring about a change in the oral cavity. This change may be due to the etching of the enamel surfaces that causes the demineralization of tooth surface, excess composite, or morphologic irregularities on the brackets providing a nidus for plaque accumulation. These changes in the oral cavity lead to increased plaque accumulation and decreased pH of the plaque, which causes enamel demineralization and white spot lesions. With fixed orthodontic appliances, the complex designs of the brackets make them an ideal substrate for plaque accumulation and also hinder oral hygiene.
Bacterial adhesion to orthodontic brackets is the primary step leading to plaque formation and enamel demineralization around orthodontic brackets. Metallic brackets are known to have the highest critical surface tension and surface energy because of the increased tendency for plaque accumulation. Two species that have been often associated with enamel demineralization and plaque are Streptococcus mutans and Lactobacillus acidophilus . Of these, S mutans is the most common pathologic organism. In orthodontically treated patients, elevations in levels of S mutans are seen because of the introduction of new stagnant areas for colonization which in turn lead to a decrease in the pH of plaque, causing demineralization.
Preventing these lesions is a major concern for the orthodontist, since the lesions are unesthetic, unhealthy, and potentially irreversible. The various procedures used for prevention of lesions involve proper mechanical removal of plaque along with daily use of fluoride-containing mouth rinses; the disadvantage of these processes is that they depend mainly on patient cooperation.
In the last decade, the use of photocatalyst oxide films has gained importance, of which titanium oxide (TiO 2 ) is the most commonly used. A photocatalyst is a chemical substance that acts as a catalyst when exposed to light. TiO 2 when exposed to ultraviolet light in the presence of an aqueous medium undergoes oxidation and reduction reactions to form hydroxyl radicals that are used in the decomposition of organic compounds. TiO 2 has been considered a useful substance because of its chemically stable properties, high photoactivity, stability, and relatively low cost and has been used in various industrial and environmental fields. It has been used for water purification, decontamination, and disinfection purposes. The particle sizes can be varied to produce additional beneficial effects. TiO 2 exists in 3 crystalline forms: rutile, anatase, and brookite. These crystalline structures vary in their structure and properties. The anatase crystal structure is formed between 400°C and 500°C and rutile is formed at more than 900°C. Brookite is an amorphous type that can be converted into anatase at low temperatures and into rutile at higher temperatures. It has been used in orthodontics as a coating on archwires and brackets. Various studies have shown that TiO 2 coated brackets and archwires have good antibacterial and antiadherent properties. The antibacterial effect of TiO 2 was shown to be due to the production of hydroxyl radicals that act against the bacteria in aqueous solution on illumination with ultraviolet light.
Despite proven antibacterial properties, concerns have been expressed about the biocompatibility of the TiO 2 film. In the oral cavity, there is a tendency for the TiO 2 coating on the brackets to become corroded from various iatrogenic causes. Moreover, these antibacterial coatings may exhibit cytotoxicity, causing safety issues. Although several in-vitro studies have evaluated the usefulness of TiO 2 in controlling S mutans , there is little information in the literature with regard to the cytotoxicity of TiO 2 coated orthodontic products. Moreover, no study to the best of our knowledge has assessed and compared the antibacterial effects of the rutile and anatase phases of TiO 2 on orthodontic products. Therefore, this study was designed to evaluate and compare the antibacterial efficacy and cytotoxic effects of stainless steel brackets coated with the rutile and anatase phases of photocatalytic TiO 2 .
Material and methods
This in-vitro study was conducted in 2 parts. The first part involved assessment and comparison of the antibacterial properties of TiO 2 coated brackets, and the second part evaluated the cytotoxicity of TiO 2 coated brackets under controlled laboratory conditions.
The brackets used for the study were stainless steel premolar brackets, prescription 0.022 × 0.028-in slot size (Mini 2000; Ormco, Orange, Calif). A total sample size of 115 brackets was taken. All were autoclaved, and 68 of these brackets underwent the TiO 2 coating procedures.
Surface coating of the brackets with TiO 2 was carried out by the magnetron sputtering method (Anelva RF sputtering unit, SPF-332H; ARML, Bangalore, India).
The TiO 2 target is used as the cathode, and the stainless steel brackets to be coated are used as the substrate. The procedure is carried out in a vacuum where no other reaction occurs. This sputtering process removes surface atoms from the target which is the TiO 2 , and a layer of thin film is coated onto the surface of the brackets. The whole coating procedure took 4 hours at room temperature, and a 50-to-80 nm TiO 2 coating was obtained.
Then the brackets were treated with heat (calcination). The 68 brackets coated with TiO 2 were oxidized in a muffle furnace. For conversion into the anatase phase, half of the coated brackets (34) were subjected to a temperature of 450°C for 1 hour. For conversion into the rutile phase, the other half of the brackets (34) were subjected to a temperature of 1000°C for 4 hours.
The sample size for the study was determined with G*Power software (version 3.1.3; University of Kiel, Germany) with 0.80 statistical power at the 0.05 significance level.
For the antibacterial study, there were 4 groups: group 1, 13 stainless brackets coated with the anatase phase of TiO 2 ; group 2, 13 stainless steel brackets coated with the rutile phase of TiO 2 ; group 3, 13 uncoated stainless steel brackets (positive control); and group 4, 13 uncoated stainless steel brackets (negative control).
For the cytotoxicity study, the 3 groups were group 1, 21 stainless brackets coated with the anatase phase of TiO 2 ; group 2, 21 stainless steel brackets coated with the rutile phase of TiO 2 ; and group 3, 21 uncoated stainless steel brackets (positive control).
To determine the antibacterial activity of the TiO 2 coated brackets, we used a lyophilized culture of S mutans MTCC-497 (Microbial Type Culture Collection and Gene Bank, Chandigarh, India) that was procured commercially. The bacterial strains were then reconstituted according to the manufacturer’s instruction in the brain-heart infusion broth.
The antibacterial activities of the 2 types of TiO 2 coated brackets were tested against S mutans .
Group 1 brackets were put into a test tube containing 1 mL of brain-heart infusion broth to which 10 8 colony forming units of MTCC-497 S mutans were added.
Similarly in groups 2 and 3, the S mutans strains were added to the brackets.
In group 4, only the brackets were put into the brain-heart infusion broth, without S mutans . Before adding the S mutans in groups 1 and 2, the test tubes with brackets were activated with an ultraviolet light (TL-D/08 [RS] Blacklight Blue; Philips Electronics, Chennai, India) with an intensity of 1.0 mW/cm 2 for 60 minutes.
After inoculation, all test tubes belonging to the 4 groups were incubated at 37°C for 48 hours. After 48 hours, the tubes were visualized for turbidity. All brackets were removed from the test tubes aseptically. A test tube dilution of 1:10 broth was made using sterile brain-heart infusion, and the broth was subcultured into brain-heart infusion agar for colony quantification. The plates were then incubated for 37°C overnight. Readings of the plate showing bacterial growth were taken, and growth was confirmed as S mutans by performing gram stain reaction and biochemical reaction.
For the assessment of cytotoxicity, mouse subcutaneous connective tissue fibroblast (L929) cells were grown in Dulbecco’s Modified Eagles Medium (NCCS, Pune, Lonza, Switzerland) containing 10% fetal bovine serum, 10,000 IU per milliliter of penicillin, 10,000 μg per milliliter of streptomycin, and 250 μg per milliliter of amphotericin-B in a 25 cm 2 culture flask in an incubator at 37°C with 5% carbon dioxide in a controlled humidified atmosphere. Once the cells reached about 85% confluency, they were trypsinized using trypsin (0.05%)-EDTA (0.54 mmol/L) solution, washed thoroughly with media, and subcultured into a 75-cm 2 culture flask for expansion. This process was repeated twice until the cells attained a consistent growth phase. After that, they were trypsinized at 70% to 80% confluency and then used for the assay.
For the assessment of cytotoxicity of the TiO 2 coated metal brackets by thiazolyl tetrazolium bromide assay, the cells were trypsinized when they were at about 80% confluence and seeded in a 96-well plate at the density of 7 × 10 3 cells per well. The cells were incubated in an incubator at 37°C with 5% carbon dioxide under a controlled humidified atmosphere overnight to allow them to attach to the plate. After overnight incubation, the cells were exposed to TiO 2 coated metal brackets (7 brackets each coated with anatase and rutile) for 1, 3, and 6 days, along with a control group in which cells were exposed to metal brackets with no Ti O 2 coating. The brackets were activated using an ultraviolet light with an intensity of 1.0 mW/cm 2 for 60 minutes before exposing the cells. At the end of days 1, 3, and 6 of exposure, 50 mL of thiazolyl tetrazolium bromide (5 mg/mL stock) was added to the cells and further incubated for 3 hours at 37°C. At the end of the incubation period, the contents of the plate were discarded by simple decantation, and the plates were dried overnight at room temperature. The purple-colored formazon crystals formed were dissolved in 100 mL of dimethyl sulfoxide by shaking at 400 rpm for 15 minutes at room temperature in a thermo shaker. The intensity of the color developed was absorbed at 570 nm in a multimode microplate reader. The percentage viability of the cells was calculated using the following formula.
Percentage viability = Optical density of test ×100/Optical density of control.
Cell viability was scored according to the method of Sjogren et al : more than 90% cell viability, noncytotoxic; 60% to 90% cell viability, slightly cytotoxic; 30% to 59% cell viability, moderately cytotoxic; and less than 30% viability, severely cytotoxic.
Statistical analysis
The collected data were analyzed with SPSS software for Windows (version 16.0; SPSS, Chicago, Ill). To describe the data, descriptive statistics—means and standard deviations—were used. To find the significant difference between the bivariate samples in paired groups, the Wilcoxon signed rank test was used; for independent groups, the Mann-Whitney U test was used. For the multivariate analysis, the Kruskal-Wallis test was used; for repeated measures, the Friedman test was used. In all these statistical tools, the probability value of 0.05 was considered to be significant. These tests were done for both the antibacterial and cytotoxicity studies.
Results
For the assessment of antibacterial activity, the survival rates of the bacteria were compared in terms of colony forming units. As shown in Tables I and II and Figure 1 , maximum bacterial growth was seen in group 3 followed by groups 1 and 2. This suggested that maximum antibacterial activity was shown by the group 2 brackets. Group 4. a negative control, had no bacterial growth. The Kruskal-Wallis analysis showed a statistically significant difference in bacterial concentration ( P <0.5) in the comparisons of the 3 groups. The intergroup statistical comparisons were significant between groups 1 and 2, groups 1 and 3, and groups 2 and 3 ( Table II ). Both groups 1 and 2 brackets showed significant antibacterial activity, and group 2 had the greatest activity.
Groups | n | Mean | SD | SE |
---|---|---|---|---|
Anatase (group 1) | 13 | 3.31 | 0.947 | 0.263 |
Rutile (group 2) | 13 | 2.31 | 0.751 | 0.208 |
Positive control (group 3) | 13 | 4.69 | 0.480 | 0.133 |
Total | 39 | 3.44 | 1.231 | 0.197 |
∗ Mean = power of 10 |
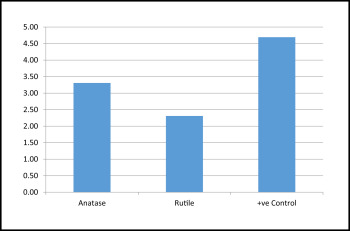
For the assessment of cytotoxicity, the data of the cytotoxicity study were evaluated as percentages of the control. The viability of cells in the control group was maximum at all 3 time intervals as reflected by the optical density data that demonstrated minimum cytotoxicity at all 3 time intervals ( Table III ).
Group | Day 1 | Day 3 | Day 6 | SEM | ||
---|---|---|---|---|---|---|
Anatase (group1) |
89.152 | 77.770 | 89.423 | 7.02 | 15.96 | 14.47 |
Rutile (group2) |
40.018 | 23.287 | 21.694 | 13.65 | 6.05 | 12.63 |
Control (group3) |
100.000 | 100.000 | 100.000 | 0.340 | 0.230 | 0.162 |
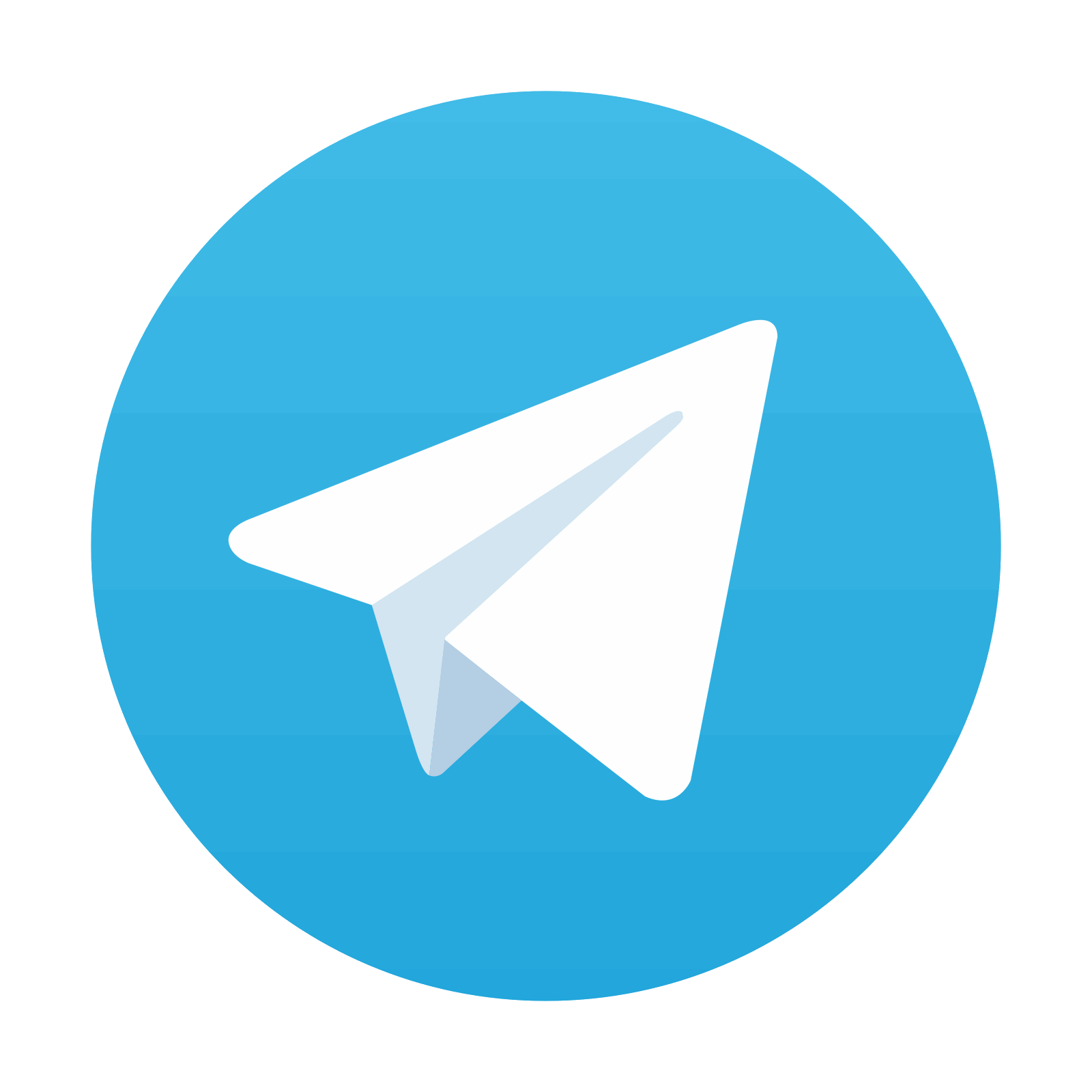
Stay updated, free dental videos. Join our Telegram channel

VIDEdental - Online dental courses
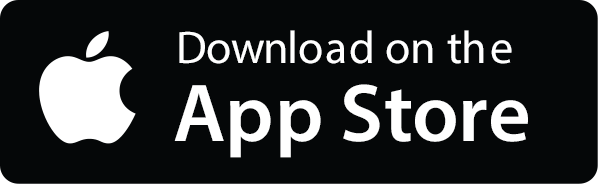
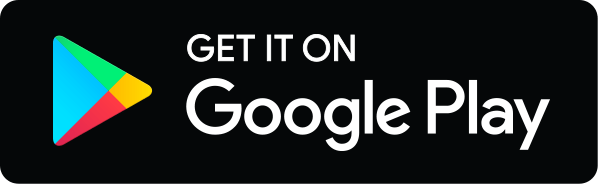
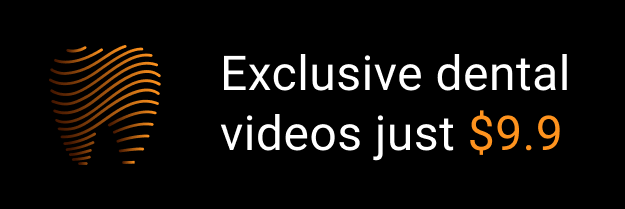