Introduction
Our objective was to evaluate the effect of the diameter of the archwire on tooth alignment with different bracket-archwire combinations.
Methods
The materials included 2 categories of orthodontic brackets (1) conventional ligating brackets (Victory Series [3M Unitek, Monrovia, Calif], Mini-Taurus [Rocky Mountain Orthodontics, Denver, Colo], and Synergy [Rocky Mountain Orthodontics]) and (2) self-ligating brackets (SmartClip [3M Unitek], a passive self-ligating bracket; Time3 [American Orthodontics, Sheboygan, Wis], an active self-ligating bracket; and SPEED [Strite Industries, Cambridge, Ontario, Canada], an active self-ligating bracket). All brackets had a nominal 0.022-in slot size. The brackets were combined with Therma-Ti 0.014-in and Therma-Ti 0.016-in titanium memory archwires (American Orthodontics). The archwires were tied to the conventional brackets with both stainless steel ligatures (0.010 in) and elastomeric rings. Each bracket-archwire combination was tested 20 times with the orthodontic measurement and simulation system built in a temperature-controlled chamber where the temperature was kept at 37°C (±1°C) during testing. The malocclusion simulated in the study represented a maxillary central incisor displaced 2 mm gingivally (x-axis) and 2 mm labially (z-axis).
Results
The incisogingival corrections achieved by the 0.014-in archwire combined with the brackets used ranged from 40% to 95%; the corrections by the 0.016-in wire were 55% to 95%. The labiolingual corrections achieved by the 0.014-in archwire combined with the brackets used ranged from 10% to 100%, and the corrections of the 0.016-in archwires ranged from 15% to 100%.
Conclusions
Increasing the diameter from 0.014 to 0.016 in increased the correction achieved by up to 15% in certain bracket-archwire combinations, but it decreased the correction by up to 25% in other combinations.
Highlights
- •
We studied the effects of changes in archwire diameter on tooth alignment.
- •
Displacements of a maxillary central incisor 2 mm gingivally and labially were simulated.
- •
We combined 0.014- and 0.016-in titanium memory wires with 6 types of brackets.
- •
Changing from 0.014 to 0.016 in increased the correction at best by 15%.
- •
Smaller archwires are recommended in leveling and alignment to avoid high forces.
The ultimate goal of the new introductions in orthodontics is to improve patient care, including diagnosis, treatment planning, treatment mechanics, and treatment results, by making processes more effective and efficient. The orthodontic market receives new products every day; consequently, a wide variety of choices is available for orthodontists.
The selected bracket-archwire combination has a determining effect on the resultant orthodontic outcome. Although self-ligating brackets have been developed to overcome the disadvantages of conventional ligation, until now there is no evidence-based support of such a claim. Systematic reviews of clinical studies did not show evidence of differences between the efficiency or the effectiveness of self-ligating and conventional bracket systems. Nickel-titanium archwires gained rapid popularity for use in orthodontics because of their mechanical properties, including low modulus of elasticity, high springback, and wide force-delivery ranges; so, clinicians were especially interested in these wires during leveling and alignment. Accordingly, nickel-titanium archwires of larger diameters were sometimes used by clinicians early in treatment because they can be easily engaged to even the most severely malaligned teeth. As attention to wire size and shape retreated in favor of the structural properties of the new wires, the composition of nickel-titanium archwires invited researchers to study friction between these archwires and different bracket combinations.
When planning orthodontic mechanics, orthodontists should consider not only the amount of tooth movement, but also the biologic effect of tooth movement. The bottom line when planning orthodontic tooth movement is that effective tooth movement requires an optimum orthodontic force that would produce maximum tooth movement, maximum biologic response, and maximum patient comfort, thus achieving the optimum force requirements and also supporting the orthodontic paradigm shift from the heavy forces of the past to the appropriate gentle forces that are optimum for cellular response.
The objective of this study was to evaluate the effect of archwire diameter change on the amount of alignment achieved during correction of complex tooth malalignment with different bracket-archwire combinations using the orthodontic measurement and simulation system (OMSS).
Material and methods
The study design and the materials we used are summarized in Table I . The materials included 6 orthodontic bracket types of 2 categories: (1) conventional ligating brackets (Victory Series, 3M Unitek, Monrovia, Calif; Mini-Taurus, Rocky Mountain Orthodontics, Denver, Colo; and Synergy, Rocky Mountain Orthodontics) and (2) self-ligating brackets (SmartClip, a passive self-ligating bracket, 3M Unitek; Time3, an active self-ligating bracket, American Orthodontics, Sheboygan, Wis; and SPEED, an active self-ligating bracket, Strite Industries, Cambridge, Ontario, Canada). Synergy is a specially designed conventional low-friction bracket with 6 tie wings. All brackets had a nominal 0.022-in slot size. Two heat-activated archwires were used in combination with the 6 brackets. These archwires were preformed maxillary Therma-Ti 0.014-in and Therma-Ti 0.016-in titanium memory wires (American Orthodontics). To control confounders arising from the archwires’ composition and manufacturing treatments, the archwires were chosen from the same type; the only difference was the diameter. Also, a new archwire was used for each test, and the temperature of the surrounding environment was kept at 37°C ± 1°C. The archwires were tied to the conventional brackets with both stainless steel ligatures of size 0.010 in (Advanced Orthodontics, Näpflein, Düsseldorf, Germany) and elastomeric rings with a 2.94-mm outer diameter (3M Unitek). Combining the 6 types of brackets with the 2 archwire sizes and the 2 types of ligation for conventional brackets resulted in 18 groups. Each combination was tested 20 times, for a total of 360 tests, to measure the amount of alignment of a maxillary central incisor adjusted in a simulated malocclusion with a 2-mm gingival displacement represented on the x-axis and a 2-mm labial displacement represented on the z-axis.
Bracket type | Bracket width at central incisor (mm) | Bracket width at lateral incisor (mm) | Type of ligation | Type of wire | Group observations | Total observations |
---|---|---|---|---|---|---|
Mini-Taurus | 3.6 | 3.1 | Stainless steel ligation Elastic ligation |
|||
Victory Series | 3.6 | 2.9 | Stainless steel ligation Elastic ligation |
|||
Synergy | 3.4 | 3.4 | Stainless steel ligation Elastic ligation |
0.014-in titanium memory 0.016-in titanium memory |
20 | 360 |
Smart-Clip | 3.8 | 3.3 | Passive self-ligation | |||
Time3 | 2.5 | 2.4 | Active self-ligation | |||
SPEED | 2.5 | 2.3 | Active self-ligation |
The OMSS comprises 2 force-moment sensors capable of registering forces and moments in the 3 planes of space simultaneously ( Fig 1 ). The 2 sensors are mounted on motor-driven positioning tables that can move freely in the 3 planes of space. Commands regarding the conditions of the experiment are given to the OMSS through a personal computer. Two microcomputer-based sensor electronics deliver the digital output of the force moment vectors to a personal computer where the resultant force-deflection curves are recorded, thus facilitating a means to study the loads from the simulated orthodontic tooth movement. The whole mechanical assembly of the OMSS is built in a temperature-controlled chamber that is especially important when testing temperature-dependant alloys. The OMSS was programmed to move the maxillary right central incisor bracket from its initial position (representing a well-aligned maxillary right central incisor) 2 mm gingivally and 2 mm labially (the simulated malocclusion) and then to move it back to the initial position in 0.01-mm increments. The center of resistance of the simulated maxillary central incisor was positioned 10 mm apical and 4.5 mm lingual to the point of force application based on a mathematical model that calculates a vector of tooth movement taking into account the center of resistance of the simulated tooth.
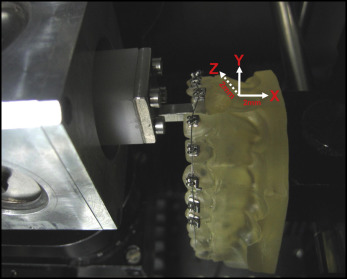
A mold (Frasaco, Greenville, NC) of a normally aligned maxillary arch was used to prepare resin replicas (Palavit G 4004; Heraeus Kulzer, Hanau, Germany) that were later fixed to the OMSS. The right central incisor was removed from each resin replica to allow for placement of the sensor for testing in the OMSS. On each resin replica, the orthodontic brackets were bonded from second premolar to second premolar with a cyanoacrylate adhesive. A jig was used to standardize the bonding process of the right central incisor bracket to a bracket holder that would be attached to the first sensor of the OMSS. The archwires were tied to the conventional brackets with both stainless steel ligatures and elastomeric rings. With the stainless steel ligatures, the ligature was tied around the bracket wings using a needle holder; the ligature was first tightened and then loosened by 1 turn to allow free movement of the archwire. The elastomeric ligatures were positioned using a needle holder, and a 3-minute waiting period was allocated to allow a reproducible amount of stress relaxation to occur as recommended by Henao and Kusy. On the other hand, with self-ligating brackets, the archwire was inserted into the brackets, and the clips were closed.
The measurement procedure started with mounting the resin replica on the OMSS table and fixing the bracket holder, with the bracket of the maxillary right central incisor bonded to it, to the first sensor. The sensor was then adjusted so that the bracket was in the right position in the prepared space in the resin model, and the whole assembly simulated a well-aligned dental arch, and the bracket was not subjected to forces and moments because the exerted forces and moments were adjusted as close to zero as possible. At this point, the measurement started by moving the bracket attached to the sensor from the initial position 2 mm gingivally (x-axis) and 2 mm labially (z-axis). The correctional movement then took place automatically, the brackets moved toward the correct position, and the malocclusion correction was achieved in incremental steps of 0.01 mm, which means that the measurements were made on the unloading path. The quotient of the amount of achieved correction and the amount of initial displacement multiplied by 100 gave the percentage of correction of the bracket-archwire combination; ie, a final position of 1 mm of gingival displacement equals a correction of 50%. To evaluate the effect of archwire diameter on the amount of correction achieved, the amount of correction with the 0.014-in archwire was subtracted from that of the 0.016-in archwire combined with the same bracket and ligation. When the amount of correction achieved was greater with the 0.016-in archwire, the difference was given a plus sign, and when the amount of correction was smaller, a minus sign was given to the difference.
Statistical analysis
The maximum absolute values of the correction of malalignment achieved in the 2 directions of the x- and z-axes were calculated from the recordings of the OMSS software for each sample. Descriptive statistics including means, standard deviations, and maximum and minimum values were calculated for each bracket-archwire combination and were then statistically analyzed with 2-way analysis of variance (ANOVA) followed by least significant tests. The data were analyzed using SAS/STAT software (SAS, Cary, NC) for statistical analysis.
Results
Descriptive statistics of the corrections in the x-axis (incisogingival) and the z-axis (labiolingual) directions are presented in Tables II and III and in Figure 2 .
Wire size (in) | n | Bracket type | ||||||||
---|---|---|---|---|---|---|---|---|---|---|
Mini-Taurus Steel ligation |
Mini-Taurus Elastic ligation |
Victory Series Steel ligation |
Victory Series Elastic ligation |
Synergy Steel ligation |
Synergy Elastic ligation |
Smart-Clip | Time3 | SPEED | ||
Correction (x-axis) | ||||||||||
0.014 | 20 | 1.8 ± 0.2 a | 0.8 ± 0.2 c | 1.8 ± 0.1 a | 1.2 ± 0.4 b | 1.8 ± 0.1 a | 1.9 ± 0.1 a | 1.9 ± 0.1 a | 1.8 ± 0.2 a | 1.9 ± 0.1 a |
90% ± 10% | 40% ± 10% | 90% ± 5% | 60% ± 20% | 90% ± 5% | 95% ± 5% | 95% ± 5% | 90% ± 10% | 95% ± 5% | ||
0.016 | 20 | 1.5 ± 0.3 c | 1.1 ± 0.4 d | 1.5 ± 0.3 c | 1.2 ± 0.2 d | 1.8 ± 0.1 ab | 1.9 ± 0.0 a | 1.9 ± 0.2 ab | 1.9 ± 0.1 ab | 1.8 ± 0.3 b |
75% ± 15% | 55% ± 20% | 75% ± 15% | 60% ± 10% | 90% ± 5% | 95% ± 0% | 95% ± 10% | 95% ± 5% | 90% ± 10% | ||
Correction (z-axis) | ||||||||||
0.014 | 20 | 1.4 ± 0.4 c | 0.2 ± 0.1 d | 1.8 ± 0.2 b | 0.3 ± 0.1 d | 1.9 ± 0.1 ab | 2.0 ± 0.1 a | 1.9 ± 0.2 b | 1.8 ± 0.3 b | 1.5 ± 0.4 c |
70% ± 20% | 10% ± 5% | 90% ± 10% | 15% ± 5% | 95% ± 5% | 100% ± 5% | 95% ± 10% | 90% ± 15% | 75% ± 20% | ||
0.016 | 20 | 1.1 ± 0.7 d | 0.3 ± 0.2 e | 1.3 ± 0. 6 d | 0.4 ± 0.1 e | 1.8 ± 0.2 ab | 1.9 ± 0.3 a | 1.7 ± 0.4 b | 2.0 ± 0.2 a | 1.5 ± 0.3 c |
55% ± 35% | 15% ± 10% | 65% ± 30% | 20% ± 5% | 90% ± 10% | 95% ± 15% | 85% ± 20% | 100% ± 10% | 75% ± 15% |
Wire size (in) | n | Bracket type | ||||||||
---|---|---|---|---|---|---|---|---|---|---|
Mini-Taurus Steel ligation |
Mini-Taurus Elastic ligation |
Victory Series Steel ligation |
Victory Series Elastic ligation |
Synergy Steel ligation |
Synergy Elastic ligation |
Smart-clip | Time3 | SPEED | ||
Correction (x-axis) | ||||||||||
0.014 | 20 | 1.8 ± 0.2 a | 0.8 ± 0.2 a | 1.8 ± 0.1 a | 1.2 ± 0.4 a | 1.8 ± 0.1 a | 1.9 ± 0.1 a | 1.9 ± 0.1 a | 1.8 ± 0.2 a | 1.9 ± 0.1 a |
90% ± 10% | 40% ± 10% | 90% ± 5% | 60% ± 20% | 90% ± 5% | 95% ± 5% | 95% ± 5% | 90% ± 10% | 95% ± 5% | ||
0.016 | 20 | 1.5 ± 0.3 b | 1.1 ± 0.4 b | 1.5 ± 0.3 b | 1.2 ± 0.2 a | 1.8 ± 0.1 a | 1.9 ± 0.0 b | 1.9 ± 0.2 a | 1.9 ± 0.1 a | 1.8 ± 0.3 a |
75% ± 15% | 55% ± 20% | 75% ± 15% | 60% ± 10% | 90% ± 5% | 95% ± 0% | 95% ± 10% | 95% ± 5% | 90% ± 10% | ||
Correction (z-axis) | ||||||||||
0.014 | 20 | 1.4 ± 0.4 a | 0.2 ± 0.1 a | 1.8 ± 0.2 a | 0.3 ± 0.1 a | 1.9 ± 0.1 a | 2.0 ± 0.1 a | 1.9 ± 0.2 a | 1.8 ± 0.3 a | 1.5 ± 0.4 a |
70% ± 20% | 10% ± 5% | 90% ± 10% | 15% ± 5% | 95% ± 5% | 100% ± 5% | 95% ± 10% | 90% ± 15% | 75% ± 20% | ||
0.016 | 20 | 1.1 ± 0.7 a | 0.3 ± 0.2 a | 1.3 ± 0.6 b | 0.4 ± 0.1 b | 1.8 ± 0.2 b | 1.9 ± 0.3 a | 1.7 ± 0.4 a | 2.0 ± 0.2 b | 1.5 ± 0.3 a |
55% ± 35% | 15% ± 10% | 65% ± 30% | 20% ± 5% | 90% ± 10% | 95% ± 15% | 85% ± 20% | 100% ± 10% | 75% ± 15% |
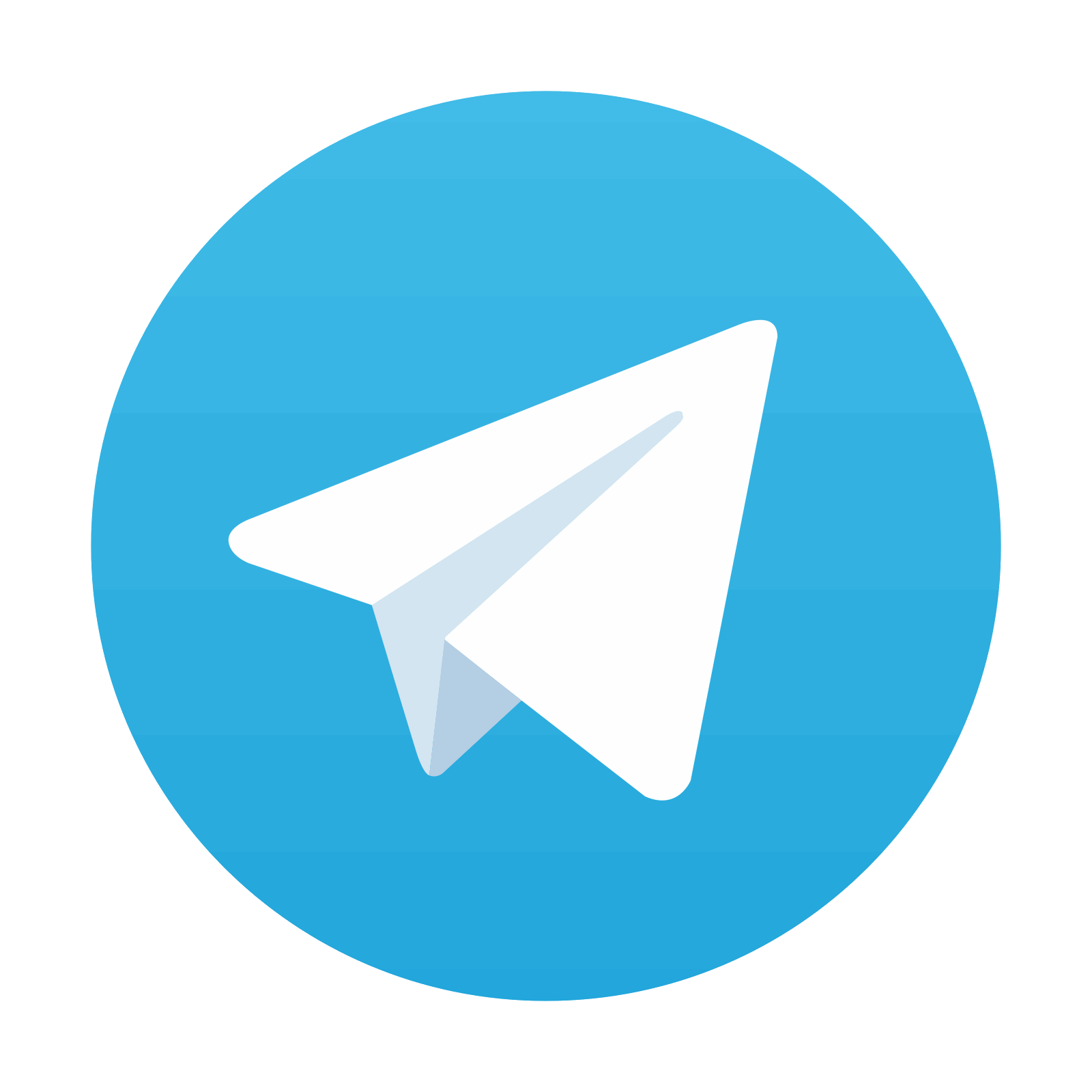
Stay updated, free dental videos. Join our Telegram channel

VIDEdental - Online dental courses
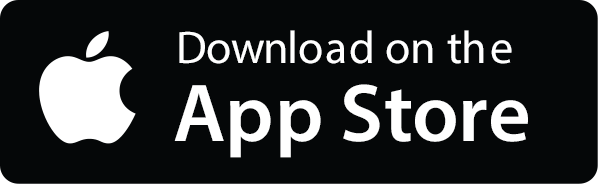
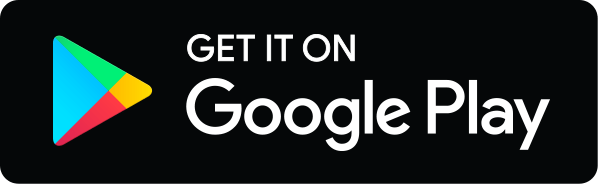
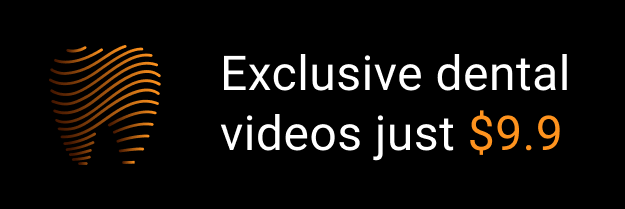