Highlights
- •
Pulp canal sealer exerted the highest antibacterial activity.
- •
Chlorhexidine increased the antibacterial activity of all sealers investigated.
- •
BioRoot was the most affected sealer by CHX in terms of physicochemical properties.
- •
Chlorhexidine did not affect AH Plus and BioRoot as shown by surface characterization.
- •
Two extra phases were observed for Pulp Canal Sealer under chlorhexidine irrigation.
Abstract
Objectives
Assess the antibacterial, physical and chemical properties of AH Plus, BioRoot RCS and Pulp Canal Sealer (PCS) in contact with 2% chlorhexidine digluconate (CHX) used as final irrigant prior to root canal obturation.
Methods
The antimicrobial properties were investigated by direct contact tests for planktonic and biofilm growth of E. faecalis , S. mutans , S.epidermidis and S.aureus in vitro . The setting time, wettability, microhardness and surface roughness were also assessed. The sealers were studied in no contact, 1-minute (short-term) and continuous contact (long-term) with CHX. Chemical characterization of sealers was performed by scanning electron microscopy, X-ray diffraction analysis and Fourier-transform infrared spectroscopy after CHX or saline used as the last irrigant in an ex vivo tooth model and in endo training blocks.
Results
CHX increased the antibacterial activity of all the sealers investigated against planktonic bacteria and biofilms with PCS exerting the highest antimicrobial activity with and without the presence of CHX. The setting of AH Plus and BioRoot RCS was retarded, while for PCS accelerated in the presence of CHX. AH Plus and PCS were more hydrophilic after contact with CHX, whilst BioRoot RCS was hydrophobic in a time-dependent manner. The microhardness of sealers was compromised and the surface roughness increased after CHX exposure for AH Plus and BioRoot RCS, and decreased for PCS. CHX did not affect the sealers’ chemistry, but PCS that exhibited two extra phases.
Significance
CHX improved the antibacterial efficacy of endodontic sealers but further evidence is needed to confirm its suitability as a final irrigant prior to root canal obturation.
1
Introduction
The primary aim of root canal treatment of teeth with apical periodontitis is to eliminate the microbial load from the root canal system and promote periapical healing [ , ]. Meticulous mechanical debridement of the root canal system significantly reduces the bacterial load and is considered important in canal disinfection [ ]. However, complete elimination of all microorganisms is challenging, as viable bacteria potentially remain on the dentin walls and inside dentinal tubules, both in planktonic forms and biofilms [ , ]. About 35% of the root canal area is left untouched when conventional rotary and hand instruments are used [ ]. Therefore, disinfection with irrigation solutions during root-canal treatment and thereafter obturation of the root canal are important factors to reduce the amount and growth of residual bacteria [ ].
Different irrigation solutions such as sodium hypochlorite (NaOCl), chlorhexidine digluconate (CHX), 17% ethylene diamine tetracetic acid (EDTA), citric acid and MTAD are used in endodontic treatments [ , ]. NaOCl is widely used, as it dissolves organic material and has antibacterial properties [ ]. CHX has antibacterial properties but does not dissolve organic tissue. Unlike NaOCl, it has the ability to be absorbed, bind to dentin and be gradually released (substantivity), which may contribute to a prolonged antibacterial effect [ , ]. CHX is a cationic substance that kills the bacteria by acting at the microbial cell wall or outer membrane [ ].
Endodontic sealers play an important role in obturation of the root canal system as they may provide a seal, which prevents the penetration of bacteria. Sealers are meant to entomb residual bacteria, prevent leakage of nutrients and ideally possess antibacterial properties [ , ]. Sealers with numerous chemical compositions are used in endodontics, such as zinc oxide eugenol- (ZOE), resin-, silicone- and calcium silicate- based materials [ ]. AH Plus (Dentsply Maillefer, Ballaigues, Switzerland) is a resin-based root canal sealer that is frequently used as a benchmark for comparisons [ , ]. BioRoot RCS (Septodont, Saint-Maur-des-Fossés, France) is a hydraulic calcium-silicate based sealer and it possesses both antibacterial [ ] and biological properties [ ]. Its hydraulic nature and the formation of calcium hydroxide as part of the hydration process makes the sealer very susceptible to the environment it is placed in [ ]. Pulp Canal Sealer (Kerr Corporation, Romulus, MI, USA) is a traditional eugenol containing sealer that has been in clinical use for decades possessing antibacterial properties, but controversial biocompatibility [ ].
Several studies have addressed the effect of irrigation solutions on sealers’ properties such as sealing ability, microleakage, and wettability [ , ]. However, there is scant information regarding the effect of irrigation solutions on sealers’ antibacterial properties. To the best of our knowledge only one study has investigated the effect of final irrigation with water, EDTA and phosphate buffered saline (PBS) on antibacterial activity of BioRoot RCS, MTA Fillapex (Angelus, Londrina, Brazil) and AH Plus. The irrigation solution affected the antibacterial properties of all three sealers as they exhibited the highest antibacterial activity after irrigation with EDTA followed by water [ ].
Remnants of irrigation solutions are present in the root canal system after completion of chemo-mechanical root canal preparation [ , ]. CHX in 2% concentration is often used in endodontics as a final irrigant before placement of endodontic sealers [ ]. Due to binding to dentin and subsequent release of CHX, this may influence the sealers’ properties. Many different procedures for debridement and irrigation of the root canal are described in the literature. Debridement and irrigation are important to reduce the amount of bacteria in the root canal, however the most efficacious combination of irrigation solutions and obturating materials is not known.
The primary aim of this in vitro study was to assess the antibacterial, physical (physicomechanical) and chemical properties of AH Plus, BioRoot RCS and Pulp Canal Sealer after exposure to 2% chlorhexidine digluconate. An ex vivo tooth model and endo training blocks were used to simulate irrigation procedures. The null hypothesis tested was that sealers’ antibacterial and physicochemical properties would not be affected after contact with CHX.
2
Materials and methods
An epoxy resin-based sealer, AH Plus (Dentsply Maillefer, Ballaigues, Switzerland), a calcium silicate-based sealer, BioRoot™ RCS (Septodont, Saint-Maur-des-Fossés, France), and a zinc oxide eugenol-based sealer, Pulp Canal Sealer (PCS) (Kerr Corporation, Romulus, MI, USA) were tested. The materials were mixed according to manufacturer’s instructions. Chlorhexidine digluconate, 20% in water solution, (Lot # BCBS7878V, Sigma-Aldrich, St.Louis, MO, USA) was diluted in sterile distilled water (water) and standardized to 2%. Regarding physical and antibacterial testing, standard CHX volumes were used with the ultimate aim to sufficiently cover the surface area of the sealers. Taking into account the different levels of hydrophilicity of sealers, the guiding principle was to apply as less CHX was possible to achieve full coverage of the tested materials in order to imitate the clinical scenario. CHX was used as a last irrigant in both tooth model and endo training blocks for assessing chemical properties.
2.1
Antibacterial assays
All experiments were conducted in triplicate and with three independent parallels for each material investigated. Enterococcus faecalis American Type Cell Culture Collection (ATCC) 19434, Streptococcus mutans ATCC 700610, Staphylococcus epidermidis ATCC 35984, Staphylococcus aureus Newman were grown overnight for 18 hours in Tryptone Soya Broth (TSB) at 37° C, 5% CO 2 supplemented atmosphere. The bacteria were suspended in Phosphate Buffered Saline to an optical density at 600 nanometers (OD 600 ) of 1.0, corresponding to approximately 2 × 10 8 Colony Forming Units (CFUs)/ml. The sealers were tested against both planktonic bacteria and bacteria in biofilms for three different groups of exposure to CHX: group 1, no CHX (no contact with CHX); group 2, short CHX (1-minute contact with CHX); group 3, long CHX (continuous contact with CHX along with setting process).
A direct contact test (DCT) was used to investigate the antibacterial activity of sealer surfaces against planktonic bacteria. Briefly, the bottoms of a 96-well microtiter plate (Costar, Flat bottom, Ultra low attachment, Corning Inc, Corning, NY, USA) were coated with each sealer by using a small size round ended dental instrument. A fixed amount of 15 μl CHX was applied on the sealer surfaces (≈ 0.53 μl CHX/mm 2 ). The same amount of CHX within the same application times was also transferred on uncoated wells serving as negative controls. The CHX drop was applied upon the sealers with a pipette and evenly spread with a sterile plastic inoculation loop. In short-term exposure groups, after 1 minute of contact with CHX, the drop was removed with a pipette and the sealers were placed in a dry incubator at 37 °C for 20 minutes to let any liquid excess dry out, before allowed to set. Subsequently, half of the samples were covered with 300 μl saline. All the materials were stored in humidified atmosphere at 37 °C and incubated for 24 hours. After incubation, the supernatant saline solution was removed from the wells and all surfaces proceeded for testing. The sealer surfaces were tested either with or without saline application, constituting two experimental conditions. An amount of 10 μl from each bacterial suspension was carefully placed on the surface of the materials. Another 10 μl from the same bacterial suspension was transferred to uncoated wells serving as positive control. The specimens were incubated at 37 °C for 1 hour, while complete evaporation of the suspension’s liquid was inspected. Colonies of surviving bacteria were calculated after serial dilution in PBS and plating on TSB agar plates incubated overnight at 37 °C, 5% CO 2 supplemented atmosphere (Supplementary Figure S1).
For biofilm assay, membrane filters (MF-Millipore™ Membrane Filter, 0.45 μm pore, Merck, Darmstadt, Germany) were cut in circular 3-mm diameter pieces and placed upon TSB agar plates. A droplet of 2 μl of each bacterial inoculum OD 600 1.0 was applied upon the outer surface of membranes. The agar plates were incubated at 37 °C in a 5% CO 2 supplemented atmosphere for 48 hours and monospecies biofilms were established and verified with the use of confocal laser scanning microscopy (CLSM; Olympus FluoView FV1200, Olympus Corp, Tokyo, Japan) ( Fig. 1 ). The Syto-9/Propidium iodide (PI) staining (FilmTracer™ LIVE/ DEAD Biofilm Viability kit, Thermo Fisher Scientific Inc., Waltham, MA, USA) was used to color the biofilms upon membranes. A diode laser emitting at 473 nanometres (nm) was used. The scanning was performed from the top of the biofilm to the membrane surface using a 60× water lens, 0.5 μm step size, and a format of 512 × 512 pixels corresponding to an area of 88 × 88 μm (Figure S2). Caps of 0.2-mL polypropylene thin wall PCR tubes (Axygen, Corning, NY) were cut and filled with the mixed sealers, and a glass microscope slide was applied on the cap to obtain smooth surfaces. A fixed amount of 10 μl CHX was applied on the sealer surfaces with a pipette and evenly spread with a sterile plastic inoculation loop (≈ 0.79 μl CHX/mm 2 ). After incubation period, the filter membranes were positioned upon the sealers with the established biofilms facing their surfaces. Membranes also covered uncoated bottoms, serving as positive controls. Parafilm M (Bemis Inc, Neenah, WI, USA) was applied around the caps to ensure tight contact between membranes and sealer surfaces. The specimens were placed at 37 °C in a 5% CO 2 supplemented atmosphere for 24 hours. After 24 hours, a droplet of 10 μl water was transferred upon the membranes to enable gentle detachment from the sealer and the caps’ bottoms. Each membrane with its corresponding cap was transferred to vials containing 5 ml PBS and vigorously vortexed with glass beads. After serial dilutions in PBS, CFUs were counted after overnight incubation at 37 °C in a 5% CO 2 supplemented atmosphere (Figure S3). Carry over effect of the method was also assessed. Filter membranes with established biofilms served as positive controls and were placed in vials containing 5 ml PBS. Sealer specimens inside caps were allowed to set independently, as it was aforementioned, for 24 hours at 37 °C in a humidified chamber and then put in the same vial. These samples were vigorously vibrated with glass beads. Possible carryover effect was measured after serial dilutions and CFUs were calculated as described previously. Experiments for potential carryover effect were performed in triplicate.

2.2
Assessment of Physical Properties
The physical properties were assessed by testing the setting time, wettability (contact angle measurements), microhardness, and surface roughness of sealers. The experiments were performed in triplicates with at least three parallel samples.
Cylindrical specimens, measuring 10 mm in diameter and 2 mm in height, were prepared into molds for each sealer. For setting time, wettability and microhardness tests, after preparation the sealers were incubated in 100% humidified atmosphere at 37 °C and allowed to set for 24 hours with and without contact with 2% CHX or water. Five groups were formed according to exposure to CHX or water: group 1, no CHX/water (no contact with CHX or water); group 2, short CHX (1 minute contact time with CHX); group 3, long CHX (continuous contact with CHX along with the setting process); group 4, short water (1 minute contact time with water); group 5, long water (continuous contact with water along with the setting process). For both CHX and water exposure groups, a drop of 25 μl was applied upon the sealers with a pipette and evenly spread with a sterile plastic inoculation loop (≈0.32 μl CHX or water/mm 2 ). In short-term exposure groups, after 1 minute of contact either with CHX or water, the drop was removed with a pipette and the sealers were placed in a dry incubator at 37 °C for 20 minutes to liquid excess dry out, before allowed to set for 24 hours in a humidified atmosphere. For surface roughness test, in addition to no contact and CHX groups, measurements of the samples were also taken for 24 hours contact with Hanks’ Balanced Salt Solution (HBSS; Sigma Aldrich, Gillingham, UK) (Figure S4).
2.2.1
Assessment of setting time
The setting times of the sealers were analyzed in compliance to ISO 6876 (2012) [ ]. A stopwatch was started after the preparation of the sealers and the placement of CHX or water upon them in the short-term and long-term exposure groups. The specimens were placed in an incubator at 37 °C and 100% humidity until the end of setting. Setting of the sealers was assessed using an indentation technique with a Gilmore-type metric indenter, having a mass of 100.0 ± 0.5 g and a flat end of diameter 2.0 ± 0.1 mm. The sealers were considered as set when the indenter was lowered gently onto the material surface and did not leave any visible round indentation on it.
2.2.2
Contact Angle Measurements
Contact angle measurement was used to investigate the wettability of the material surfaces. A 20-μL drop of distilled water was placed with a syringe on the surface of the samples, and images were captured using a color video camera (JVC KY-F55B, JVCKENWOOD Corporation, Yokohama, Japan). The contact angle was measured using an image processing and analysis software (L.P. Optimas 6.5, Media Cybernetics Inc., Rockville, MD, USA).
2.2.3
Microhardness Test
Microhardness testing was carried out by applying an indentation technique, using a hardness-testing instrument (Struers A/S, Rødevre, Denmark). A pyramidal square-based diamond indenter was lowered onto the sealer surfaces and a load ranging up to 100 gf was applied for a dwell time of 5 s. At least two independent indentations at a distance of 5 mm selecting non-overlapping microscopical regions were performed on each sample and the Vickers hardness number (VHN) was recorded.
2.2.4
Assessment of Surface Roughness
Surface analysis of the samples was carried out using mechanical profilometry (Form Talysurf Series 2, Taylor Hobson, Leicester, UK) and scanning electron microscope (SEM) imaging (EVO MA10, Carl Zeiss Ltd, Cambridge, UK). The profiler used a precision motion system and a gauge head to measure the displacement at the sealer surface over a specified area. The region measured was 3 mm in a straight line at the center of each sample with a measurement taken every 2.5 μm. The vertical axis range was set at 1 mm and a resolution of 16 nm was used. The average arithmetic roughness (Ra) was recorded. Representative secondary electron scanning micrographs of the tested materials were made to picture the sealers’ surface microstructure (magnification 100×).
2.3
Sealer characterization in ex vivo tooth model and endo training blocks
A split tooth model and endo training blocks (Endo-Training-Bloc, Dentsply Maillefer, Ballaigues, Switzerland) were used to simulate a clinical setting with irrigation of CHX as the last irrigant.
Twenty four, single-rooted anterior and posterior human teeth with one root canal (Ethical approval REC Ref 14/EM/1128), free of caries, were decoronated and the root length standardized at 15 mm, using a diamond saw (Buehler 11-1280-160 Isomet Low Speed Saw, Buehler, Lake Bluff, IL, USA) and consequently stored at 4 °C in water until use. The root canals were instrumented with ProTaper rotary files (Dentsply Maillefer, Ballaigues, Switzerland) up to size F4, 1 mm shorter than the standardized root length. An irrigation protocol with 2 mL of 2.5% NaOCl between the changes of the rotary files with a 27 gauge Monoject 3cc Endodontic Syringe (CardinalHealth, Dublin, Ireland) was followed. The roots were split longitudinally along their long axis using a diamond saw and the two segments were repositioned and held tightly together by wrapping them up with Parafilm M. The root canals were irrigated with either 2 ml of saline or 2 ml of saline and then 2 ml of 2% CHX for 1 minute as the final irrigant. The root canals were dried with paper points before placement of sealers.
The tested sealers were placed inside the root canals using a lentulo spiral after which they were allowed to set for 24 hours in 37 °C, 100% humidity. After the setting period, each root was unwrapped from the Parafilm M, and the root fragments were gently detached with a use of a scalpel that was applied on the thin space formed between them. The sealers were exposed and gently retrieved intact from the dentin walls. After the separation process, the whole bulk of the sealer was adhered to one segment whilst the other segment was macroscopically free of sealer remnants. Hereafter, the exposed sealer on its half-tooth segment will be referred as sealer-tooth sample. The aforementioned preparation was also applied on endo training resin blocks (Figure S5). The exposed sealer on its resin block segment will be referred as sealer-block sample. The exposed sealers upon their substrates (either tooth or endo training blocks) were characterized by scanning electron microscopy (SEM) (tooth model) and energy dispersive spectroscopy (EDS) (tooth model), X-ray diffraction analysis (XRD)(tooth model and endo training blocks), and Fourier-transform infrared spectroscopy (FTIR) (tooth model and endo training blocks) (Figure S6).
2.3.1
Scanning Electron Microscopic examination-Elemental analysis
SEM examination was performed on sealer-tooth samples. These were mounted on aluminum stubs, carbon coated (Agar Scientific, Stansted, UK), and viewed with the scanning electron microscope (EVO MA10, Carl Zeiss Ltd, Cambridge, UK). Accelerating voltage ranged between 5–15 kV and the probe current between 125–300 pA. High magnification EDS chemical analysis was carried out at 15 kV and a working distance of 8.5 mm. Scanning electron micrographs at high magnification in the backscatter electron mode were captured, and EDS was performed in rectangular areas of the intact sealers surface.
2.3.2
X-ray Diffraction analysis
Phase analysis of both the sealer-tooth and sealer-block samples was carried out. Cylindrical samples (10 mm diameter, 2 mm height) of sealers in no contact with CHX were also prepared and analyzed as controls. The surface analysis was performed using glancing angle X-ray diffraction analysis at a fixed angle of incidence of 5. The X-ray diffractometer (Bruker D8 Discover, Bruker, Billerica, Massachusetts, MA, USA) was operated in grazing-incidence asymmetric Bragg mode using Cu Ka radiation, an operating current of 40 mA and voltage of 45 kV for 15–458 2 u with a sampling width 0.058, scan speed 18/min. The other settings included divergent slits at 1 mm, divergent height slit 10 mm, scintillator slit 8 mm and receiver slit 13 mm. Phase identification was accomplished using a search-match software utilizing Crystallography Open Database (COD) (Diffrac.Eva, Bruker, Billerica, Massachusetts, MA, USA).
2.3.3
Fourier transform infrared spectroscopy (FT-IR)
The measurements were performed in FT-IR spectrometer (Nicolet 6700, Thermo Scientific, Waltham, Massachusetts, USA) on sealer-tooth and sealer-block samples. The spectra were taken by the Smart MIRacle Accessory, Diamond setup. The data were analyzed by stacking the spectra retrieved from the FT-IR, using the accompanied software (Omnic).
2.4
Statistical analysis
The statistical analysis was performed with GraphPadPrism version 6.00 for windows (GraphPad software, La Jolla, CA, USA). The antibacterial assays were analyzed using the nonparametric Kruskal–Wallis test and Dunn’s post-hoc method due to absence of normal distribution (p < 0.05). Statistical analysis of the physical properties was performed using one-way analysis of variance, followed by Tukey multiple comparisons (p < 0.05).
3
Results
3.1
Antibacterial Properties
No surviving planktonic bacteria of any species tested were recovered when CHX was applied in short- (1 minute) and long-term (along with setting process) for all sealers investigated and negative controls. For AH Plus without contact with CHX, there was no difference in the number of bacteria recovered from samples in saline or without saline for all tested species. BioRoot RCS without contact with CHX did not significantly reduce the numbers of surviving bacteria compared to controls except for S. mutans (p < 0.05). Saline application on BioRoot RCS rendered the sealer to lose its antibacterial properties compared to control (p > 0.05). PCS without CHX and saline did not exhibit any antibacterial properties against E. faecalis, S. epidermidis and S. aureus except for S. mutans . PCS with saline application eliminated all the bacteria of any species (p < 0.05). The data for the antibacterial properties of sealers on planktonic bacteria are shown in Table 1 .
The antibacterial activity was further investigated against bacteria in biofilms. For AH Plus, long-term exposure to CHX, increased the antibacterial activity against monospecies biofilms for all bacteria investigated (p < 0.05). BioRoot RCS was effective against S.epidermidis biofilms in all conditions investigated (p < 0.05). After long-term exposure to CHX, it was antibacterial against biofilms formed by any of the bacterial species investigated (p < 0.05). In addition, short-term contact with CHX improved the antibacterial efficacy of BioRoot RCS against S.aureus biofilms. PCS reduced bacterial survival in E. faecalis and S. mutans biofilms in all conditions (p < 0.05). Short- and long-term exposure to CHX had an antibacterial effect on S. epidermidis and S. aureus biofilms compared to controls (p < 0.05). In negative controls, only long-term exposure to CHX significantly reduced surviving bacteria in S. mutans biofilms (p < 0.05). No carryover effect was detected in the biofilm model (data not shown). The results for the antibacterial properties of sealers on biofilms are shown in Table 2 .
Biofilms | AH Plus | BioRoot RCS | PCS | Controls |
---|---|---|---|---|
E. faecalis | ||||
no CHX | 10.510 (0.279) | 8.916 (1.306) | 8.54 (0.819)* | 10.540 (0.278) /9.944 (0.128)/8.505 (0.494) |
short CHX | 10.620 (0.178) | 8.642 (0.805) | 7.591 (0.886)* | |
long CHX | 7.447 (0.513)* | 6.362 (0.541)* | 7.759 (0.379)* | |
S. mutans | ||||
no CHX | 9.960 (0.234) | 8.472 (1.077) | 4.778 (0.699)* | 9.903 (0.257) /6.613 (1.123)/0 (0)*0 (0)* |
short CHX | 6.623 (0.284) | 6.759 (1.263) | 0 (0)* | |
long CHX | 0 (0)* | 0 (0)* | 0 (0)* | |
S. epidermidis | ||||
no CHX | 10.599 (0.329) | 6.477 (0.323)* | 8.305 (0.485) | 10.701 (0.299) /10.10 (0.248)/8.676 (0.311) |
short CHX | 10.49 (0.518) | 6.778 (0.426)* | 7.846 (1.099)* | |
long CHX | 7.929 (0.114)* | 5.903 (0.517)* | 6.699 (0.401)* | |
S. aureus | ||||
no CHX | 10.748 (0.202) | 8.346 (0.534) | 8.833 (0.236) | 10.731 (0.211) /10.38 (0.273)/8.675 (0.246) |
short CHX | 9.995 (0.191) | 8.065 (0.992)* | 8.022 (0.577)* | |
long CHX | 7.914 (0.222)* | 7.477 (0.271)* | 7.643 (0.584)* |
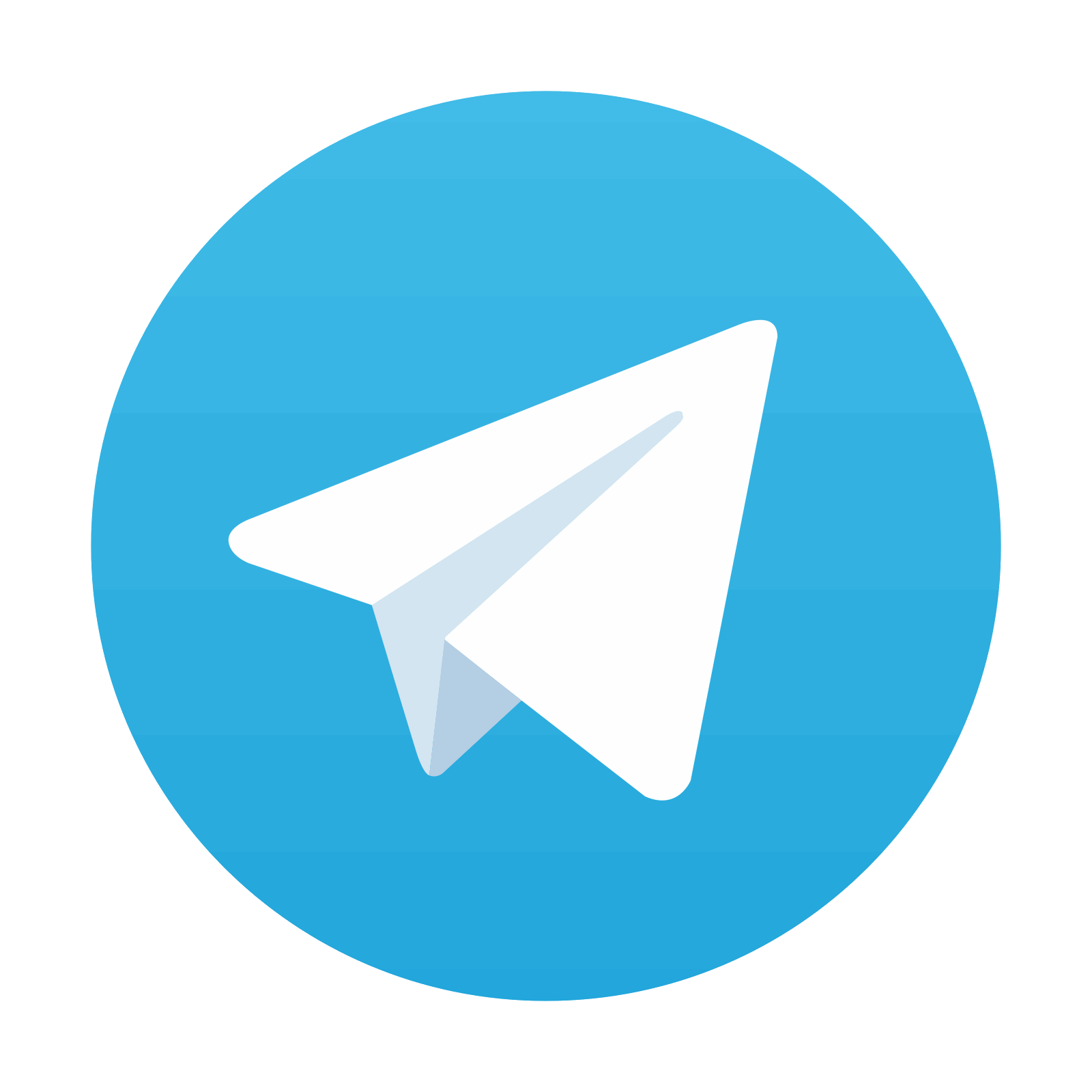
Stay updated, free dental videos. Join our Telegram channel

VIDEdental - Online dental courses
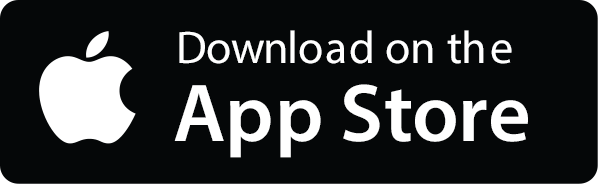
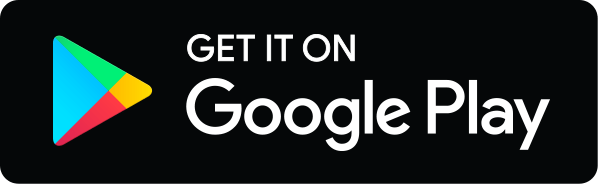
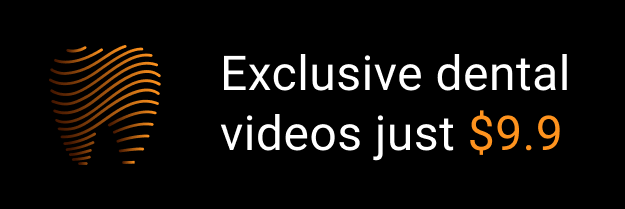