Introduction
Surgically assisted mandibular arch expansion is an effective treatment modality for alleviating constriction and crowding. However, only mandibular symphyseal osteotomy is recommended for mandibular arch expansion. No relevant studies have compared the biomechanical responses of different corticotomy designs on mandibular expansion. Therefore, the aim of this study was to evaluate the effect of different corticotomy approaches and modes of loading on the expansion of adult mandibles using biomechanics.
Methods
Nine finite element models including 2 novel corticotomy designs were simulated. Stress, strain, and displacement of crown, root, and bone were calculated and compared under different corticotomy approaches and loading conditions.
Results
The biomechanical response seen in the finite element models in terms of displacement on the x-axis was consistent from anterior to posterior teeth with parasymphyseal step corticotomy and tooth-borne force application. In addition, the amount of displacement predicted by parasymphyseal step corticotomy in the tooth-borne mode was greater compared with other models.
Conclusions
These results suggest that parasymphyseal step corticotomy with tooth-borne force application is a viable treatment option for true bony expansion in an adult mandible.
Highlights
- •
Corticotomy design can influence mechanical response of dentoalveolar structures.
- •
Type of loading can affect mechanical response of dentoalveolar structures.
- •
The expansion predicted for step corticotomy appears to be more significant.
- •
Step corticotomy with tooth-borne mode was found to achieve substantial expansion.
A tooth size-arch length discrepancy is a common form of malocclusion seen in clinical practice. Clinical characteristics including decreased mandibular arch length, narrow intercanine width, mandibular anterior teeth crowding, and posterior buccal crossbite are associated with transverse mandibular deficiency. Moreover, from the treatment aspect, it is often believed to be a critical factor in decision making for surgical or nonsurgical expansion.
Compared with maxillary deficiency, transverse mandibular deficiency has received little attention from researchers recently. A variety of nonsurgical methods including the Schwarz and bihelix appliances have been used, with limited dimensional change and questionable long-term stability. Expansion of the mandibular arch reported in these studies was localized to alveolar bone and mostly resulted in inclination of the teeth, without changes in the mandibular body. Moreover, a compromised periodontium caused by excessive dental expansion and proclination in addition to compromised facial esthetics have been noticed as disadvantages for such treatments. However, the results of combined surgical and orthodontic treatment for adults requiring an increase in the lateral dimension have shown promising results. Recent reports have shown that corticotomy-assisted orthodontic treatment is a well-accepted option with a predictable outcome that offers solutions to many limitations associated with orthodontic treatment.
The first evidence of application of corticotomy in orthopedics comes from the early 1900s. Since then, corticotomy-assisted orthodontic treatment has been used as an effective treatment alternative for the correction of various dentoalveolar discrepancies. A patented technique called accelerated osteogenic orthodontics was also introduced by Wilcko et al in this regard. Corticotomy-assisted orthodontic treatment involves selective alveolar decortication to induce a state of increased tissue turnover that is followed by faster tooth movement, resulting in reduced treatment time. In addition, corticotomy-assisted orthodontic treatment offers other advantages such as safer expansion of constricted arches and enhanced postorthodontic treatment stability.
Three-dimensional (3D) finite element (FE) analysis is a contemporary research tool for numeric simulation of mechanical processes of a real physical system that offers several advantages, including accurate representation of complex geometries and easy model modification. Also, it is considered a valid and reliable approach for quantitative evaluation of stress-strain and displacement in the dentoalveolar structures. Moreover, it provides the freedom to simulate orthodontic force systems applied clinically, in addition to evaluation and prediction of the dentoalveolar response to the mechanical loads in 3D spaces, thereby limiting the number of animal experiments.
The physiologic characteristics of bone to adapt to the mechanical environment when subjected to mechanical load by means of 2 inherent mechanisms—bone modeling and bone remodeling—are well known. Moreover, the concept of selective decortications followed by increased tissue turnover and transient osteopenia might be implied for correction of transverse mandibular deficiency. Hence, it can be hypothesized that with the change in the design of corticotomy, accompanied by different modes of force application, the biomechanical response of the bone tissue might change, and advanced mandibular expansion can be achieved that further contributes substantially to better treatment outcomes. With this intent, this study was aimed to compare the biomechanical effects of different modes of force application on 3 corticotomy designs and to select an optimal approach for clinical adoption with respect to a corticotomized mandible. Although a number of studies have used the FE method to investigate the biomechanics and mechanobiology of mandibular symphyseal osteotomy, to the best of our knowledge, this is the first study of its kind, comparing 3 corticotomy cuts including 2 novel corticotomy approaches with different modes of force application using the FE method.
Material and methods
A cone-beam computed tomography scan projection of an adult mandible was obtained from the Department of Oral and Maxillofacial Radiology, College of Stomatology, Chongqing Medical University, in Chongqing, China. This scan (slice thickness, 1 mm; pixel size, 0.42 mm) served as the pattern for construction of the mathematical model. Processing of the data was performed using Mimics software (version 9.0; Materialise, Leuven, Belgium). The generated DICOM file was imported into the Mimics software for semiautomatic edge detection, followed by meshing of surface elements using an automated meshing module (Geomagic Studio; Geomagic, Morrisville, NC) to construct the 3D analytical model of the mandible and dentition through thresholding, region growing, and calculating 3D operations. The study protocol was approved by the ethics committee of Chongqing Medical University, and informed consent was obtained from the subject before the study.
With the help of Rapidform software (version 6.5; INUS, Seoul, Korea), we performed scaling and Boolean operations on the surface model of individual teeth and mandibular bone to produce cortical bone, trabecular bone, and periodontal ligament with average thicknesses of 2.0, 2.0, and 0.2 mm, respectively, thereby creating parametric solids from 3D scans. For the purpose of generating the geometric model, 5 materials including teeth, mucosa, trabecular bone, cortical bone, and periodontal ligament were assembled; they were assumed to be linearly elastic, homogeneous, and isotropic as shown in Figure 1 . Furthermore, the orthodontic appliances and miniscrew implants were designed and modeled in CAD software (Solid Works, Dassault Systems, Concord, Mass). All components were saved in initial graphics exchange specification format and imported into ANSYS (Swanson Analysis Systems, Houston, Tex).
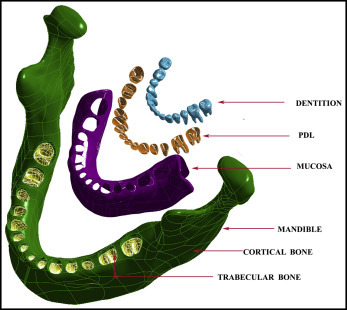
The constructed model was then exported to the FE software ANSYS, which divided the geometric model into finite elements; these elements were connected to adjacent elements by nodes, thereby creating a numeric representation of the geometric model. Table I gives the types and numbers of elements and nodes. Furthermore, to simulate the temporomandibular joint (TMJ), 2 blocks of temporal bone were made, and the space between temporal bone and condyles was filled by a 2-mm thick layer of articular disc. With regard to boundary conditions for the TMJ, constraints were placed on the 2 bone blocks in all 3 axes ( Fig 2 ). Geometric and FE models used in the study are shown in Figure 2 , A and B , respectively). Subsequently, the simulated FE model behaved like the actual prototype after the allocation of material properties such as Young’s modulus and Poisson’s ratio (lateral strain/longitudinal strain). The material properties were determined from the values in the literature ( Table II ).
Material | Elements | Nodes |
---|---|---|
Cortical bone | 15040 | 28112 |
Trabecular bone | 20735 | 36206 |
Periodontal ligament | 35772 | 72547 |
Teeth | 10636 | 20375 |
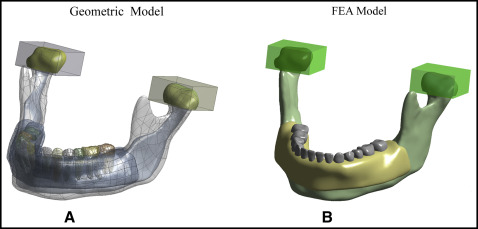
Material | Poisson’s ratio | Young’s modulus (MPa) | Reference |
---|---|---|---|
Teeth | 0.3 | 20.7E3 | Lee and Baek |
Periodontal ligament | 0.45 | 50 | Lin et al |
Trabecular bone | 0.3 | 9.7E2 | Aversa et al |
Cortical bone | 0.3 | 10.7E3 | Aversa et al |
Three corticotomy cuts including midline symphyseal corticotomy, angulated midline symphyseal corticotomy, and parasymphyseal step corticotomy were performed on the simulated FE models. Following the modern conservative approach, only the buccal cortex was perforated, whereby the vertical cut for the angulated midline symphyseal corticotomy was performed in the usual fashion between the central incisors, extending below their roots, followed by an angulated cut of almost 120° that extended laterally until the inferior border of mandible ( Fig 3 , B ). Furthermore, the parasymphyseal step corticotomy cut was oriented in a stepwise manner, including a vertical corticotomy between the canine and first premolar that extended below the canine root and was brought medially as a horizontal cut extending up to the contralateral canine-premolar region followed by a vertical corticotomy to the inferior border of the mandible ( Fig 3 , C ).
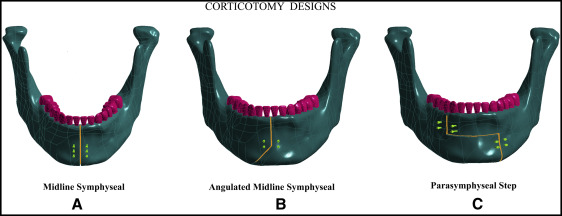
Nine FE models including 3 diverse modes of force application and 3 corticotomy approaches were designed and structured in this study ( Fig 4 ). The corticotomy approaches we used differed in the designs; the symphyseal corticotomy was performed in 3 models, whereas the other 6 models included 2 novel corticotomy designs respectively, as shown in Figure 4 , D-I . Each corticotomy approach was subjected to a tooth-borne, bone-borne, or hybrid device. Pure horizontal mechanical loads were applied in all 9 models, and each model was analyzed for stress, strain, and displacement (amount and direction in relation to the x-axis) on the tooth (crown and root) and the bone. The baseline characteristics of the study design are listed in Table III .
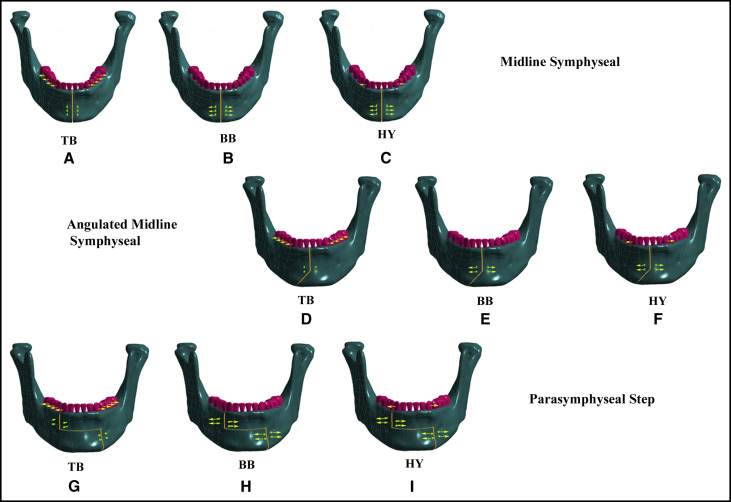
Biomechanical parameter analyzed | Mode of load application | Corticotomy design ∗ | |
---|---|---|---|
Part I | Stress (crown, root, bone) | Tooth borne | Midline |
Angulated | |||
Step | |||
Bone borne | Midline | ||
Angulated | |||
Step | |||
Hybrid | Midline | ||
Angulated | |||
Step | |||
Part II | Strain (crown, root, bone) | Tooth borne | Midline |
Angulated | |||
Step | |||
Bone borne | Midline | ||
Angulated | |||
Step | |||
Hybrid | Midline | ||
Angulated | |||
Step | |||
Part III | Displacement (crown, root, bone) | Tooth borne | Midline |
Angulated | |||
Step | |||
Bone borne | Midline | ||
Angulated | |||
Step | |||
Hybrid | Midline | ||
Angulated | |||
Step |
∗ Midline , Midline symphyseal corticotomy; angulated , angulated midline symphyseal corticotomy; step , parasymphyseal step corticotomy.
Results
The results are shown in Figures 5-7 .
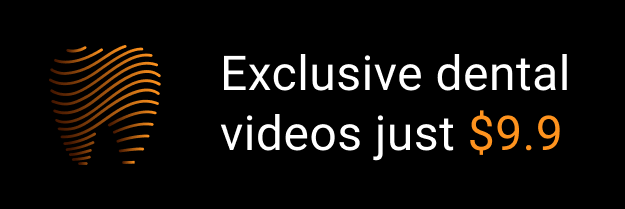