Some compelling clinical benefits of porcelain and metal adhesion are presented. Current concepts for metal adhesion are reviewed, including modifications of metal surface and resin chemistry. Porcelain adhesion is reviewed, including little-known methods that use silane but no hydrofluoric acid etching. Clinical protocols for use of metal and porcelain adhesives are presented.
Advances in adhesion monomers and surface preparation techniques permit strong bonding of resins to metals and to porcelains. Most “pressed ceramics” bond in a manner similar to that of porcelains. The bond strengths to these materials can easily exceed the typical bond of resin to phosphoric-acid–etched enamel . Using the strong bonds to these restorative materials, many innovations in tooth-conservative procedures are possible.
When we consider that tooth reduction correlates with need for subsequent endodontic treatment , the preferred treatment option often is one that relies on minimal tooth reduction. Adhesion, with less need for tooth reduction, is a highly desirable shift away from tooth preparation for mechanical retention.
This paper first presents some clinical examples where the use of adhesives clearly provides compelling advantages compared with mechanical retention and cementation. Then methods and materials to achieve resin adhesion to metal and porcelain are reviewed. Based on these methods and materials and the author’s own clinical experience, specific adhesion protocols are given for some complex clinical applications.
The clinical advantages of using porcelain and metal adhesion
If we examine the cross section of a typical molar ( Fig. 1 ), the distance from the occlusal surface to the pulp chamber is about 7 mm. A 3-mm occlusal reduction therefore leaves about 4 mm of remaining dentin thickness ( Fig. 2 ). On the other hand, axial reduction of 2 mm leaves <1 mm of remaining dentin ( Fig. 3 ). The 2-mm axial reduction is within current pressed ceramic manufacturers’ instructions for tooth preparation.
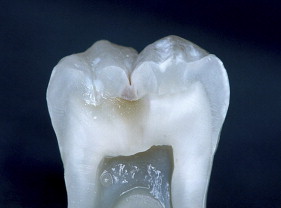
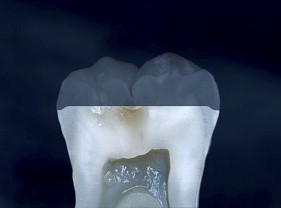
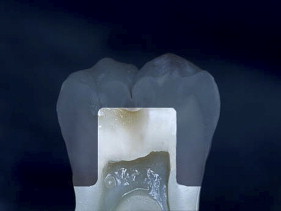
With porcelain, metal, and even dentin adhesion equivalent to etched enamel adhesion, the obvious clinical application is the adhesion onlay. The onlay may be made of metal, porcelain, or pressed ceramic ( Figs. 4 and 5 ). In these examples shown in Figs. 4 and 5 , there is no intentionally cut retention form ( Fig. 6 ). The “preparation” of the teeth was actually done by the patient through years of abrasion. No additional tooth modification was made before taking the final impression. Last photographed at 5 years and 3 months, this case remains successful after nearly 10 years.
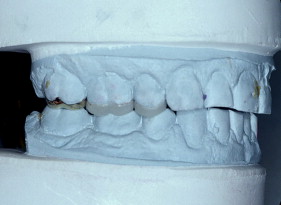
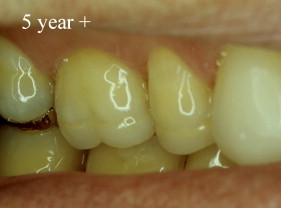
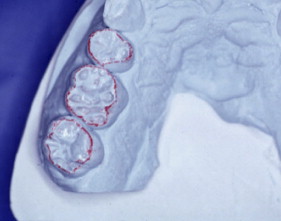
While metal adhesion onlays have been successful for many years , clinicians have been reluctant to place occlusal porcelain onlays on molars. Presumably the reluctance results from reports of clinical failure of all porcelain molar crowns. However, a recent study , where 2 mm of pressed ceramic (Empress; Ivoclar/Vivadent, Schaan, Leichtenstein) was bonded to the occlusal surfaces of molars (partial onlays, not crowns), reported 100% success at 33 months. These onlays used 2 mm of pressed ceramic, like those demonstrated on the previous case. The high success rate is presumed to be due to the ceramic being placed in compression during function, well supported by bonding to enamel and dentin.
Another tooth-conserving example is the cantilevered adhesion bridge ( Figs. 7–9 ) of porcelain fused to metal, bonded to enamel. This is similar to the “Maryland bridge” but the “Yamashita adhesion bridge” uses resistance channels placed in enamel . Such mesial and distal channels produce a highly successful result when the metal is bonded to phosphoric-acid–etched enamel.
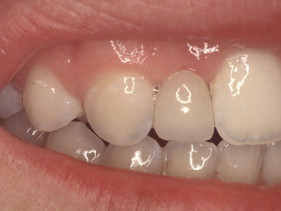
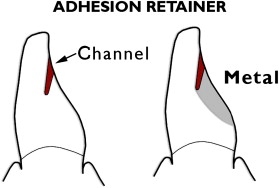
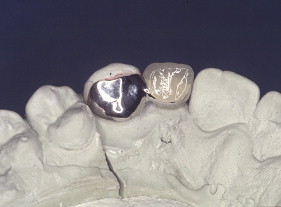
Porcelain veneers are a third compelling application of adhesives. The adherends are porcelain and enamel, porcelain and dentin, or porcelain and both enamel and dentin. Veneers can be done successfully with minimal intra-enamel tooth reduction . The survival rate in most reports exceeds 90% at 10 years. The high success rates can be attributed to durable bonding and support for the porcelain provided by tooth structures ( Figs. 10–13 ).
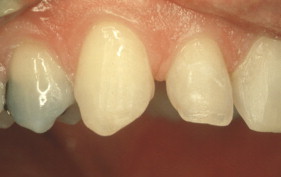
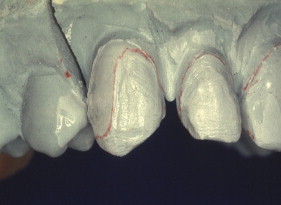
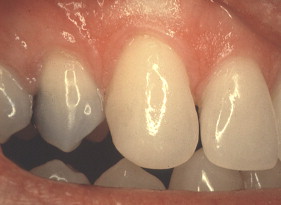
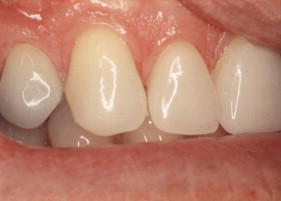
All the clinical examples illustrated above can generally be accomplished in a straightforward manner by following manufacturers’ instructions for the adhesive. While sometimes not as straightforward, porcelain repairs can also be highly successful. Some repairs require bonds to several dissimilar surfaces. After the discussion of metal adhesion and porcelain adhesion, some repair applications are illustrated and specific clinical protocols given for adhesion to multiple surfaces.
Metal adhesion
Mechanical retention
Adhesion to metal has long been a goal in dentistry. Resin-bonded “Rochette” retainers were described in 1973. Retention was provided mechanically by composite resin locking into tapered perforations in the metal. A different approach to mechanical retention, using electrolytic etching to produce a microscopically retentive surface on the entire inner surface of the metal, was subsequently reported . This etched metal is used for what is usually called the “Maryland bridge.” Using the microscopic approach, two critical factors for successful adhesion to a metal substrate are (1) using an adhesive liquid with excellent wetting characteristics and (2) providing a high-energy metal surface to promote good wetting.
Substrate modification
Several researchers took a different approach to modifying the adherend metal surface. By itself or in conjunction with other procedures, sandblasting with aluminum oxide has become an almost universal procedure. Sandblasting with aluminum oxide can be done extraorally or intraorally. Sandblasting provides a fresh and uncontaminated surface that is high in surface energy and can be easily wetted by suitable metal bonding agents. Some other reported adherend modifications are high-temperature oxidation, immersion in an oxidizing agent, anodizing, and alloying the surface with a liquid gallium–tin alloy. In contemporary dental practices, these modifications do not appear to be used often. A different and more popular approach is adding a SiO x- C coating by injecting a solution through a special flame . Then a special silane is applied to the coated surface. This method is known as Silicoater (Heraeus Kulzer, Hanau, Germany). A more recent approach, widely used, is known commercially as Rocatec in the laboratory and CoJet chairside (3M ESPE AG, Seefeld, Germany). With this method, a tribochemical silica coating is sandblasted onto the metal surface to provide ultrafine mechanical retention. When treated with the silica-coating system, the surface is not only “abraded,” but becomes embedded with a silica coating derived from silica-coated aluminum oxide particles. Metal and many other surfaces may be abraded with 30-μm grain size CoJet-Sand. Intraoral application is done with an intraoral sandblaster ( Fig. 14 ), such as the Microetcher (Danville Materials, San Ramon, California). A specialized silane coupling agent is then applied.
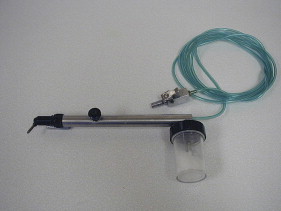
Chemical modification of filled resins
Modification of the chemistry of resin to create affinity for metals is another approach taken to strongly adhere resins to metals. Following the development in 1978 of a new adhesive monomer, 4-META , a commercial powder and liquid acryliclike product, Super Bond C&B (Sun Medical Co., Ltd., Kyoto City, Japan) was introduced in 1982 for adhesion to dental alloys and to tooth structures . To improve mechanical properties of the adhesive resin and to change the handling characteristics, another approach was taken, which resulted in the product Panavia Ex (Kuraray, Osaka, Japan) . It is a low-viscosity, quartz-filled composite resin containing a phosphate monomer known as M-10-P. Panavia is known to bond well to sandblasted base metals, but its bond to noble metals decreases when stored in water. To overcome this bond instability, the manufacturer developed a laboratory tin–plating apparatus, known as the Kura-Ace. It is used on noble metal alloys after sandblasting. Subsequently, two manufacturers produced portable tin platers capable of intraoral use . These are Kura-Ace Mini (Kuraray) and Microtin (Danville Materials). A more recent product, similar to Panavia but using a different adhesion monomer, is Bistite II DC (Tokuyama, Tokyo, Japan). Tin plating has been challenged by the use of metal primers, presumably because metal primers are more expedient to use. One recent study compared the adhesive primers Alloy Primer (Kuraray), Metal Primer II (GC America, Alsip, Illinois), and Metaltite (Tokuyama) and the resin cements Bistite II (Tokuyama), Panavia F and Super-Bond C&B. All combinations appear to be potentially successful for the bonding of prosthodontic restorations, although there were reported differences in bond stability.
Unfilled “universal” bonding resins
As opposed to using a chemically modified filled resin, another method is to use a “universal” bonding agent, such as the All-Bond 2 System (Bisco, Inc., Schaumburg, Illinois), and then add a layer of filled resin. Primer B of the All Bond 2 System can function as a metal primer . According to the manufacturer, when using the All-Bond 2 System, tin plating does not improve the bond strength of composite resin to sandblasted noble alloys. Similarly Clearfil (Kuraray) adhesives, such as Photo Bond and SE Bond, provide excellent metal bonding agents owing to the inclusion of the same adhesion monomer (M-10-P) as in Panavia.
Metal surface preparation
Contaminants on metal surfaces reduce surface energy and may compromise the bond strength to all resins. Sandblasting can remove such contaminants, creating a fresh, clean, and microscopically irregular surface with high surface energy. A high-energy surface is more wettable than a low-energy surface. An investigator, Tom Blake, looked at the effect of a delay after sandblasting, before the adhesive was applied. Blake, Director of Research at Danville Engineering, reported to the author in 2006 that the delay has a significant effect on wetting. Figs. 15 and 16 show the wetting of hydroxyethyl methacrylate (HEMA), a component of many bonding agents, at two elapsed times after sandblasting. When a measured drop of HEMA was applied to the “freshly” sandblasted gold alloy surface, a low contact angle and good wetting was observed. When the time was delayed for 24 hours after sandblasting, the HEMA did not wet as well, presumably because an absorbed layer on the metal surface lowered the surface energy.
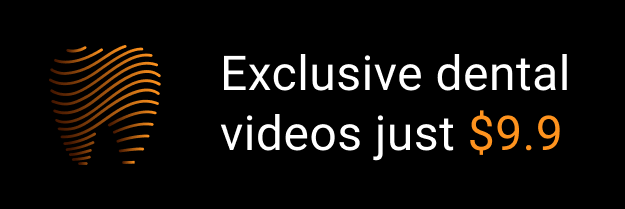