Dental cements are designed to retain restorations, appliances, and post and cores in a stable and, presumably, long-lasting position in the oral environment. Conventional glass ionomer and zinc phosphate cements are among the most popular materials for luting metallic restorations and posts, whereas resin-based cements are preferred for esthetic applications. Successful cementation of esthetic restorations is largely dependent on the appropriate treatment and silane application to the internal surface of the restoration. Clinicians are frequently advised to use three-step total-etch or two-step self-etch adhesive for luting purposes to avoid problems of incompatibility between adhesives and chemical- or dual-cure cements. A reliable cementation procedure can only be achieved if the operator is aware of the mechanisms involved and the material limitations.
Dental cements are designed to retain restorations, appliances, and posts and cores in a stable and, presumably, long-lasting position in the oral environment. Retention mechanisms for restorations secured by cements are reported to be chemical, mechanical (friction), and micromechanical (hybridized tissue). Retention of the restoration is usually achieved by a combination of two or three mechanisms depending on the nature of the cement and the substrate.
Acceptable clinical performance of dental cements requires that they have adequate resistance to dissolution in the oral environment, strong bond through mechanical interlocking and adhesion, high strength under tension, good manipulation properties such as acceptable working and setting times, and biologic acceptability for the substrate .
Many dental cements are commercially available, including resin-based and non–resin-based cements. Traditionally, zinc phosphate cement has been regarded as the most popular luting material despite its well-documented disadvantages, particularly, solubility and lack of adhesion . Glass ionomer luting cements are also of great interest for clinicians, principally because these materials release fluoride that may prevent recurrent caries . Resin-based cements are generally used for esthetic restorations (ceramic or resin based) and have become popular because they have addressed the disadvantages of solubility and lack of adhesion noted in previous materials . The advent of adhesive luting cements has considerably expanded the scope of fixed prosthodontics.
Restorative dentistry is constantly undergoing change, driven in part by new clinical applications of existing dental materials and the introduction of new materials. Currently, no commercially available luting cement is ideal for all situations. There has been considerable discussion on the properties and performance of these cements. Table 1 summarizes a variety of dental cements with their respective characteristics.
Materials | Area of application | Strengths | Weaknesses |
---|---|---|---|
Zinc phosphate cement | Routine application in metal-supported crowns, bridges, and posts | Over 100 years of clinical experience |
|
Glass ionomer cement |
|
|
|
Resin-modified glass ionomer cement |
|
|
|
Adhesive resin cement | All metal-based ceramics, lab-manufactured composite works and posts (esthetics) |
|
|
This article discusses the advantages and disadvantages of most common dental cements. Emphasis is given to resin-based cements for esthetic restorations owing to the large amount of discussion of these agents in the recent literature.
Zinc phosphate cements
Zinc phosphate cement has the widest range of applications in luting restorations, which includes the cementation of fixed cast alloys and porcelain restorations. It may also be used as a cavity liner or base to protect pulp from mechanical, thermal, and electrical stimuli . The retention of restorations cemented by zinc phosphate materials (nonadhesive luting material) is largely dependent on the geometric form of the tooth preparation that limits the paths of displacement of the cast restoration.
Zinc phosphate cement can be regarded as the first “self-etch cement,” because its acidity is capable of demineralizing the dentin surface and exposing collagen fibrils ; however, a traditional hybrid layer cannot be produced, because the acidic liquid segregates from the particles that are not capable of infiltrating the interfibrillar spaces. In fact, owing to the filtration phenomenon observed with these materials and concerns regarding possible hazard to the pulp, it has been recommended that dentin be covered with a layer of copal varnish before luting with zinc phosphate cement . The adhesive potential of zinc phosphate cement was not appreciated in former usage in prosthodontics.
Because of its long-time use and excellent clinical performance, zinc phosphate cement has been regarded as the gold standard for comparative studies. If evidence-based dentistry is strictly followed, zinc phosphate cement has far more evidence of success than any other luting material available. Apart from this strong rationale for its use, disadvantages of the cement include the negative biologic effects (pulp irritation), the lack of antibacterial action, the lack of adhesion, and the elevated solubility in oral fluids . Nevertheless, zinc phosphate cements continue to deliver successful results when used to retain metal crowns, porcelain-fused-to-metal crowns, bridges, cast posts, and other restorations. Even some all-ceramic restorations can be luted with zinc phosphate cements (eg, In-Ceram, Procera), but relevant long-term clinical data on their performance are still lacking.
Glass ionomer cements
Glass ionomer cements are commonly used for cementation of cast alloy and porcelain restorations. These cements exhibit several clinical advantages , including physicochemical bonding to tooth structures , long-term fluoride release, and low coefficients of thermal expansion ; however, their low mechanical strength compromises their use in high stress-bearing areas .
The major benefit of glass ionomer cements is their ability to adsorb permanently to the hydrophilic surfaces of oral hard tissues, offering the possibility of sealing margins developed at the tooth-material interfaces during restorative and luting procedures . Improvements in the formulation of the original glass ionomer cements have led to the development of hybrid materials that contain varied amounts of resin monomers. If the material can set properly by the acid-base reaction without the need of light activation, the material can be regarded as a resin-modified glass ionomer cement. Otherwise, if the setting mechanism is mainly directed by the light curing of the resin monomers, the material is more likely a polyacid-modified resin composite (compomer) and does not fit into the class of glass ionomer cements.
Resin-modified glass ionomer cements are indeed improved materials when compared with traditional glass ionomer cements. They represent the most used material among its class. The advantages of resin-modified glass ionomer cements include a dual-curing mode (light activated and self-curing), fluoride release from the cement, and higher flexural strengths in comparison with conventional glass ionomer cements. They are also easer to handle, including the fact that they are capable of bonding to composite materials. Although widely used as dental cements, glass ionomer cements have some disadvantages. One problem is that they do not always promote sufficient bond strength to enamel and dentin . The other disadvantage is their ability to absorb water from the surrounding environment. Premature exposure to water leads to leaching of ions and swelling and weakening of the cement, whereas loss of water leads to shrinkage and cracking of the cement . In general, bond strengths are greater to enamel than to dentin, leading to the conclusion that bonding occurs to the mineral phase of the tooth via chelation of calcium ions at the surface of the hydroxyapatite .
Glass ionomers remain the only materials that are self-adhesive to the tooth tissue without any surface pretreatment. Nevertheless, pretreatment with a weak polyalkenoic acid conditioner has been demonstrated to significantly improve their bonding and sealing efficiency . The additional conditioning step becomes more important when coarse cutting diamonds are used and consequently thicker smear layers are produced. The increase in bonding efficiency must be attributed in part to a “cleaning effect” in which loose cutting debris is removed and to a partial “demineralization” effect in which the surface area is increased and microporosities for micromechanical interlocking or hybridization are exposed. More recently, chemical adhesion to partially demineralized dentin has been demonstrated that favors the stability of the bond. In this respect, glass ionomers can be considered as adhering to tooth tissue through a self-etch approach . The basic difference with the resin-based self-etch approach is that glass ionomers are self-etching through the use of a relatively high molecular weight polycarboxyl-based polymer, whereas resin-based self-etch adhesives make use of acidic low molecular weight monomers .
Glass ionomer cements exhibit properties beyond bonding to tooth structure. They can potentially be used as matrices for the slow release of active species, as has been previously documented for fluoride ions. They are able to bond chemically to surface active glasses, which have in their compositions substances such as calcium, sodium, phosphorus, and silicon. The combination of glass ionomer cements with these bioactive glasses (BioGlass or BAG) results in glass ionomer cements with surface activity properties . The bioactive nature of BioGlass and glass ceramics is related to their ability to form a bonelike apatite layer on their surfaces in the body environment . Some findings suggest that BioGlass could be used for remineralizing damaged dentin, and that it has potential as a filler component in mineralizing restorative materials such as glass ionomer cements .
A recent study demonstrated that resin-modified glass ionomer cements containing BioGlass have the potential to mineralize dentin. In contact with saliva, these materials promote calcium phosphate precipitation on the dentin surface. The ability of these materials to precipitate minerals on the dentin surface may makes them promising resources for the treatment of dentinal hypersensitivity and for lining deep cavities in dentin.
Chlorhexidine is another example of a substance that can be incorporated into glass ionomer cements. Chlorhexidine can subsequently be released, resulting in the antibacterial properties of the cement. Additionally, chlorhexidine-releasing materials may be somewhat beneficial in preventing the action of host-derived matrix metalloproteinases in the degradation of exposed collagen ; however, the addition of chlorhexidine to glass ionomer cement may alter its properties. A recent study showed that the incorporation of chlorhexidine in glass ionomer cement resulted in increases in the working and setting times and a decrease in compressive strength . Additional studies are evaluating the benefits of incorporating active substances and fillers in the basic formula of glass ionomer cements. Clinicians may expect several improvements in glass ionomer cement–based materials in the near future. For a more complete update, readers are advised to review the September 2006 issue (volume 34, issue 8) of the Journal of Dentistry , which carries the proceedings of the Second European Glass-Ionomer Conference held in May of 2004.
Glass ionomer cements
Glass ionomer cements are commonly used for cementation of cast alloy and porcelain restorations. These cements exhibit several clinical advantages , including physicochemical bonding to tooth structures , long-term fluoride release, and low coefficients of thermal expansion ; however, their low mechanical strength compromises their use in high stress-bearing areas .
The major benefit of glass ionomer cements is their ability to adsorb permanently to the hydrophilic surfaces of oral hard tissues, offering the possibility of sealing margins developed at the tooth-material interfaces during restorative and luting procedures . Improvements in the formulation of the original glass ionomer cements have led to the development of hybrid materials that contain varied amounts of resin monomers. If the material can set properly by the acid-base reaction without the need of light activation, the material can be regarded as a resin-modified glass ionomer cement. Otherwise, if the setting mechanism is mainly directed by the light curing of the resin monomers, the material is more likely a polyacid-modified resin composite (compomer) and does not fit into the class of glass ionomer cements.
Resin-modified glass ionomer cements are indeed improved materials when compared with traditional glass ionomer cements. They represent the most used material among its class. The advantages of resin-modified glass ionomer cements include a dual-curing mode (light activated and self-curing), fluoride release from the cement, and higher flexural strengths in comparison with conventional glass ionomer cements. They are also easer to handle, including the fact that they are capable of bonding to composite materials. Although widely used as dental cements, glass ionomer cements have some disadvantages. One problem is that they do not always promote sufficient bond strength to enamel and dentin . The other disadvantage is their ability to absorb water from the surrounding environment. Premature exposure to water leads to leaching of ions and swelling and weakening of the cement, whereas loss of water leads to shrinkage and cracking of the cement . In general, bond strengths are greater to enamel than to dentin, leading to the conclusion that bonding occurs to the mineral phase of the tooth via chelation of calcium ions at the surface of the hydroxyapatite .
Glass ionomers remain the only materials that are self-adhesive to the tooth tissue without any surface pretreatment. Nevertheless, pretreatment with a weak polyalkenoic acid conditioner has been demonstrated to significantly improve their bonding and sealing efficiency . The additional conditioning step becomes more important when coarse cutting diamonds are used and consequently thicker smear layers are produced. The increase in bonding efficiency must be attributed in part to a “cleaning effect” in which loose cutting debris is removed and to a partial “demineralization” effect in which the surface area is increased and microporosities for micromechanical interlocking or hybridization are exposed. More recently, chemical adhesion to partially demineralized dentin has been demonstrated that favors the stability of the bond. In this respect, glass ionomers can be considered as adhering to tooth tissue through a self-etch approach . The basic difference with the resin-based self-etch approach is that glass ionomers are self-etching through the use of a relatively high molecular weight polycarboxyl-based polymer, whereas resin-based self-etch adhesives make use of acidic low molecular weight monomers .
Glass ionomer cements exhibit properties beyond bonding to tooth structure. They can potentially be used as matrices for the slow release of active species, as has been previously documented for fluoride ions. They are able to bond chemically to surface active glasses, which have in their compositions substances such as calcium, sodium, phosphorus, and silicon. The combination of glass ionomer cements with these bioactive glasses (BioGlass or BAG) results in glass ionomer cements with surface activity properties . The bioactive nature of BioGlass and glass ceramics is related to their ability to form a bonelike apatite layer on their surfaces in the body environment . Some findings suggest that BioGlass could be used for remineralizing damaged dentin, and that it has potential as a filler component in mineralizing restorative materials such as glass ionomer cements .
A recent study demonstrated that resin-modified glass ionomer cements containing BioGlass have the potential to mineralize dentin. In contact with saliva, these materials promote calcium phosphate precipitation on the dentin surface. The ability of these materials to precipitate minerals on the dentin surface may makes them promising resources for the treatment of dentinal hypersensitivity and for lining deep cavities in dentin.
Chlorhexidine is another example of a substance that can be incorporated into glass ionomer cements. Chlorhexidine can subsequently be released, resulting in the antibacterial properties of the cement. Additionally, chlorhexidine-releasing materials may be somewhat beneficial in preventing the action of host-derived matrix metalloproteinases in the degradation of exposed collagen ; however, the addition of chlorhexidine to glass ionomer cement may alter its properties. A recent study showed that the incorporation of chlorhexidine in glass ionomer cement resulted in increases in the working and setting times and a decrease in compressive strength . Additional studies are evaluating the benefits of incorporating active substances and fillers in the basic formula of glass ionomer cements. Clinicians may expect several improvements in glass ionomer cement–based materials in the near future. For a more complete update, readers are advised to review the September 2006 issue (volume 34, issue 8) of the Journal of Dentistry , which carries the proceedings of the Second European Glass-Ionomer Conference held in May of 2004.
Resin cements
Resin cements have become popular clinically owing to their ability to bond to both the tooth structure and restoration. The use of indirect restorations retained with adhesive procedures constitutes a substantial part of contemporary dental treatments. Metal and metal-free crowns, inlays, onlays, veneers, posts, and even resin-bonded fixed prostheses are now routinely bonded to tooth substrates by the use of adhesive resin cements ( Table 2 ) . The successful use of resin cements depends on several aspects related to the bonding mechanisms to both dental and restorative substrates. Recent publications have addressed many previously unknown issues that are key factors in determining the reliability of luting procedures with resin cements. These issues are discussed in the following sections.
Product | Company (location) | Bonding system per manufacturer | Indications | Curing mode | Additional features |
---|---|---|---|---|---|
Bistite II DC | Tokuyama (Tokyo, Japan) | Primer 1A+1B + Primer 2 | A, I, and M | Dual-curing system |
|
BisCem | Bisco (Schaumburg, Illinois) | Self-adhesive | A, B, I, M, and P | Dual-curing system |
|
Calibra | Dentsply/Caulk (Milford, Maine) | Prime & Bond NT | A, B, I, M, P, and V | Dual-curing system |
|
C&B Cement | Bisco (Schaumburg, Illinois) |
|
A, B, I, M, and P | Self-curing system |
|
Cement-Post | Angelus (Londrina, PR, Brazil) | Angelus Primer + Angelus Adhesive | A, B, M, and P | Self-curing system | Angelus silane |
Choice | Bisco (Schaumburg, Illinois) |
|
A, B, M, and V | Dual-curing system |
|
Duo-Link | Bisco (Schaumburg, Illinois) |
|
A, I, and P | Dual-curing system | Dual-syringe mixer |
Illusion | Bisco (Schaumburg, Illinois) | One-Step | A, B, I, M, P, and V | Dual-curing system |
|
Multilink | Ivoclar Vivadent (Schaan, Liechtenstein) | Primer A + B | A, B, I, M, and P | Self-curing system | Multilink Automix |
Nexus 2 | Kerr (Orange, California) | OptiBond Solo Plus | A, B, I, M, P, and V | Dual-curing system |
|
Panavia F 2.0 | Kuraray (Okayama, Japan) | ED Primer | A, B, I, and M | Dual-curing system |
|
RelyX ARC | 3M ESPE (St. Paul, Minnesota) | Adper Single Bond | A, B, I, M, and P | Dual-curing system |
|
RelyX UNICEM | 3M ESPE (St. Paul, Minnesota) | Self-adhesive | A, B, I, M, and P | Dual-curing system |
|
Super-Bond C&B | Sun Medical (Moriyama, Japan) | Monomer + catalyst V + polymer powder | A, B, I, M, P, and V | Self-curing system |
|
Variolink II | Ivoclar Vivadent (Schaan, Liechtenstein) | Excite adhesive system | A and I | Dual-curing system |
|
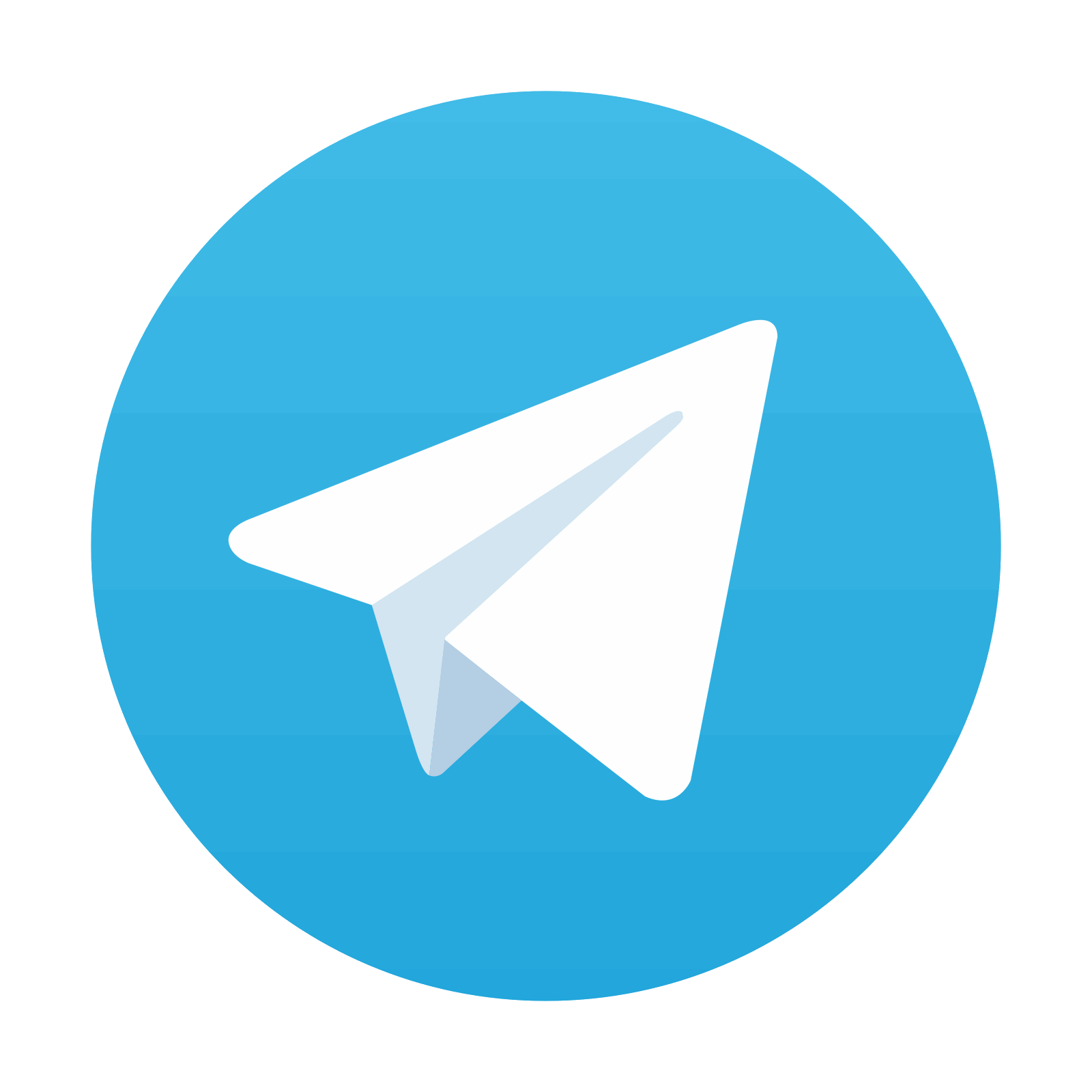
Stay updated, free dental videos. Join our Telegram channel

VIDEdental - Online dental courses
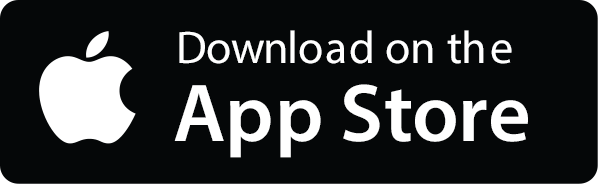
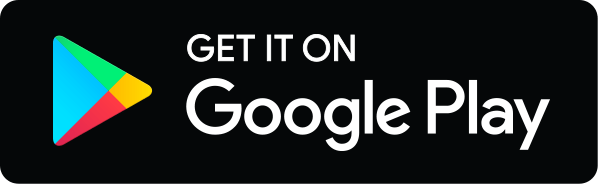
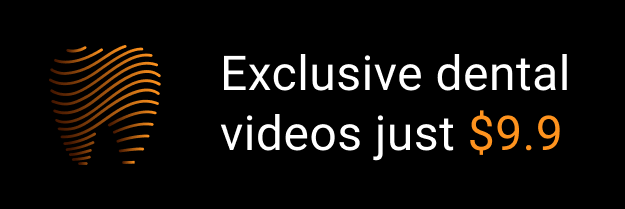