Introduction
Although current techniques for accelerated tooth movement often involve invasive surgical procedures, micro-osteoperforations (MOPs) using mini-implants may facilitate orthodontic tooth movement without raising flaps, reduce surgical risks, and increase patient acceptance. In this study, we evaluated the effectiveness of mini-implant–facilitated MOPs in inducing accelerated tooth movement and investigated the potential risks for root resorption.
Methods
Five MOPs were placed on the left side around the maxillary first molars in 6 rats using an automated mini-implant driver, whereas the right side received no MOPs as the control. Closed-coiled springs were secured from incisors to first molars for orthodontic tooth movement. Tooth movement was measured, and samples underwent radiologic and histologic analyses.
Results
The MOP side exhibited a 1.86-fold increase in the rate of tooth movement with decreased bone density and bone volume around the first molars compared with the control side. Hematoxylin and eosin and tartrate-resistant acid phosphatase analyses showed increased numbers of osteoclasts as well as new bone formation. Three-dimensional volumetric analysis of all 5 roots of the maxillary first molars demonstrated no statistically significant difference in root volumes.
Conclusions
Mini-implant–facilitated MOPs accelerated tooth movement without increased risk for root resorption and therefore may become a readily available and efficient treatment option to shorten orthodontic treatment time with improved patient acceptance.
Highlights
- •
The ability of micro-osteoperforations to accelerate tooth movement was evaluated.
- •
Orthodontic mini-implants were used to create the micro-osteoperforations in rats.
- •
This treatment significantly accelerated tooth movement compared to controls.
- •
Micro-osteoperforations did not result in significant root resorption.
Many orthodontic patients are concerned with the physical and social discomfort associated with fixed appliances. Approaches to reduce the length of orthodontic treatment have been actively pursued to reduce possible dental and periodontal complications, including external apical root resorption, increased levels of dental caries, and subsequent gingivitis and periodontitis.
Many techniques for accelerated tooth movement based on the regional acceleratory phenomenon are invasive and involve surgery, requiring simultaneous treatment by a periodontist and an orthodontist. The regional acceleratory phenomenon occurs when tissues regenerate locally in response to noxious stimuli, in an intensified remodeling process that includes increased activity by osteoclasts, osteoblasts, and inflammation markers. Wilckodontics, the first technique to use the regional acceleratory phenomenon, has proven effective in accelerating tooth movement. The technique, however, requires surgical corticotomies, cuts in the cortical bone, by raising a split-thickness flap and decorticating the bone with a round bur. Although less invasive corticotomies have been subsequently developed, such as corticision using a mallet to hammer a surgical blade into the alveolar bone and piezopuncture to penetrate the buccal cortex, patient acceptance of these methods can be low.
Recently, a new method of accelerated tooth movement using micro-osteoperforations (MOPs) was introduced to stimulate alveolar bone remodeling without creating surgical trauma. This technique was developed based on previous animal studies showing that small and shallow perforations in alveolar bone increased the rate of tooth movement without the need for flaps, bone grafting, or suturing. Alveolar bone perforations activated the cytokine cascade and subsequently increased osteoclast activity, allowing for enhanced bone remodeling after orthodontic force. A follow-up clinical study involving 20 adults showed that when perforations were delivered to 1 side of the maxilla, the procedure caused little discomfort to the patients and resulted in a 2.3-fold faster tooth movement than did traditional orthodontics alone. Because of the clinical nature of these studies, the effects of accelerated tooth movement using MOPs have yet to be observed histologically.
The objective of this study was to evaluate the ability of mini-implant–facilitated MOPs to accelerate orthodontic tooth movement. Mini-implants are a convenient tool that is already commonly used by orthodontists. Thus, we aimed not only to evaluate the effectiveness of MOPs in inducing accelerated tooth movement, but also to examine the use of mini-implants as an additional technique for MOP placement. To do so, we established a novel tooth movement model in rats that eliminated variations between animals and achieved higher statistical power by placing MOPs on 1 side of the mouth, with the other side remaining as the control. Additionally, we investigated the presence of external apical root resorption after mini-implant–facilitated MOPs. By understanding the positive and adverse effects of this technique, which creates nonsurgical trauma to the alveolar bone, orthodontic treatment can progress toward higher efficiency with fewer complications for widespread patient use.
Material and methods
Six male Sprague Dawley rats (average body weight, 500 g) underwent orthodontic force application for tooth movement on both sides of the maxillary dentition and received mini-implant–facilitated MOPs on only the left maxilla. The contralateral side, the right maxilla, was used as the control.
After the rats were anesthetized, 5 MOPs were placed 1 to 3 mm apart, mesial and palatal to the maxillary left first molar; none were placed around the maxillary right first molar ( Fig 1 ). These MOPs were created by inserting a 1.2-mm-diameter commercially available orthodontic mini-implant to a depth of 1 mm using an automated preprogrammed slow-speed implant driver at a constant torque of 30 rpm. The mini-implant successfully pierced the gingiva and created a shallow, consistent perforation in the alveolar bone. The mini-implant was marked to ensure a consistent MOP depth of 1 mm; after reaching that depth, the mini-implant was removed using the reverse function of the slow-speed driver engine. After the MOPs were placed, hemostasis was achieved using cotton pellets and pressure.
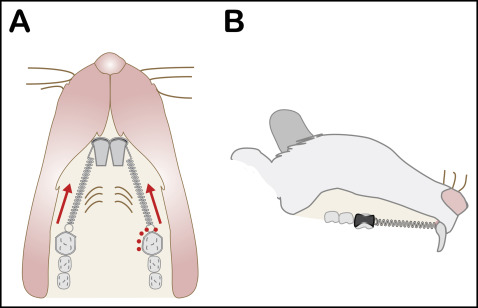
Using a high-speed handpiece, retention grooves were placed on the mesial surface of the maxillary first molar and on the facial surface extending to the distal surface of the maxillary incisor on each side. A 0.09-in stainless steel wire was bent and adapted to encircle the maxillary first molar and the maxillary incisor. Additional stability was achieved by bonding the wires into the retention grooves with bis-GMA composite resin. The wire was ligated to a 25-g nickel-titanium closed-coil spring (Sentalloy; Dentsply, York, Pa) to provide consistent light force. Because rat incisors erupt continuously, the appliances were checked daily and resecured to the most apical position of the tooth as needed. The rats were killed at 21 days with carbon dioxide asphyxiation, and their maxillae were consequently harvested and fixed in 10% buffered formalin.
The maxillae were scanned using high-resolution microcomputed tomography (SkyScan 1172; Bruker MicroCT, Kontich, Belgium) at an image resolution of 20 μm with 70 kV and a 141-μA x-ray source and a 0.5-mm aluminum filter. Three-dimensional image data sets were then reconstructed from 2-dimensional x-ray images using NRecon software (Bruker MicroCT), which processes appropriate image-correction steps including ring artifact correction, beam-hardening correction, and fine-tuning. The dynamic image range (contrast limits) was determined at 0 to 0.065 in units of attenuation coefficient and applied to all data sets for optimum image contrast. After acquisition and reconstruction of the data sets, the images were first viewed and reoriented on each 3-dimensional plane using DataViewer software (Bruker MicroCT) to align the palate parallel to the transaxial plane. To measure tooth movement, microcomputed tomography images at day 21 were used to find the distance between the enamel on the most distal aspect of the first molar and the mesial aspect of the second molar, measuring from the heights of contour. In order to evaluate differences in bone mineral density (g/cm 3 ) and bone volume fraction (%) between the MOP and the control sides, the region of interest was delineated to encompass the alveolar bone region surrounding the first molar, with limits at 1 mm mesial of the first molar to the mesial aspect of the second molar. Volumetric quantification of the root volume of the first molars was performed following a previously established protocol. After highlighting the root structure, reconstruction of slices produced a 3-dimensional representation of the root structure so that 3 buccal roots and 2 palatal roots could be digitally resected and their volumes analyzed individually. A global threshold of 80 was applied to all scans to extract physiologically accurate representations of the alveolar bone phase.
For the histologic analysis, the samples were then decalcified in 14% neutral buffered EDTA for 21 days. After decalcification, they were dehydrated through graded ethanol and embedded in paraffin. The samples were sectioned coronally in 10-μm sections. Sections were stained with hematoxylin and eosin.
Three consecutive specimens from each side were immunostained with antibodies for tartrate-resistant acid phosphatase (TRAP) using a TRAP staining kit (Sigma Aldrich, St Louis, Mo) according to the manufacturer’s instructions. Slides were then counter-stained with hematoxylin for 8 seconds to differentiate between soft and hard tissues. Osteoclasts were defined as multinucleated TRAP+ cells on the bone surface. For quantification, the numbers of TRAP+ cells were counted for the tension and pressure sides around the maxillary first molars under 100-times magnification. The numbers were presented as the number of TRAP+ cells per square millimeter of bone area. Quantification was performed by 1 operator (T.C.) blinded to the clinical information at 2 separate times.
Statistical analysis
The data were expressed as means and standard deviations for each group. Paired Student t tests were performed between the 2 groups to establish significance at an alpha of 0.05.
Results
Linear distances between the first and second molars were measured on the microcomputed tomography images for accuracy, and tooth movement at 21 days was significantly greater in the MOP side compared with the control side. On average, the MOP side (0.54 ± 0.13 mm) showed a 1.86-fold increase in tooth movement compared with the control side (0.29 ± 0.15 mm) ( Fig 2 ). All rats in the experimental group showed complete soft tissue and hard tissue healing of the MOP sites at day 21. In addition, osteopenia illustrating decreased bone volume and bone density around the maxillary first molar was observed on the MOP side compared with the control side in all animals ( Fig 3 , A and B ). According to the microcomputed tomography volumetric analysis, the MOP side (76.06% ± 2.72%) showed a statistically significant decrease in bone volume fraction compared with the control side (81.39% ± 4.60%) in all animals ( Fig 3 , C ). The MOP side (2.50 ± 0.13 g/cm 3 ) also showed a statistically significant decrease in bone mineral density compared with the control side (2.74 ± 0.22 g/cm 3 ) ( Fig 3 , D ).
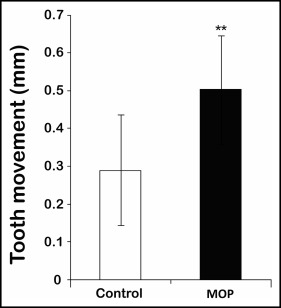
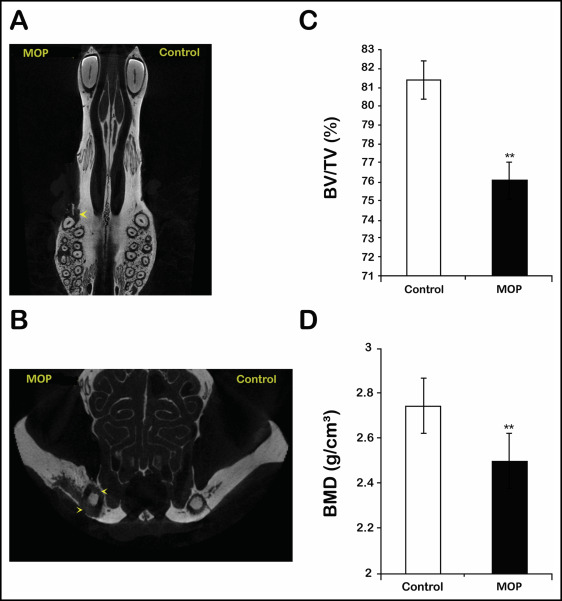
Any type of orthodontic tooth movement is generally associated with root resorption. To examine whether root resorption was induced during orthodontic movement after mini-implant–facilitated MOPs, we evaluated root resorption and bone quality using hematoxylin and eosin stained slides. Histologic examination of these slides showed that the MOP side experienced notable bone loss as indicated by the shortened interradicular trabecular bone heights and the increased numbers of multinucleated osteoclasts in all rats. Additionally, mild-moderate to moderate root resorption with blunted root apices on the MOP side was found in 3 animals ( Fig 4 , A ). On the other hand, the control sides showed normal interradicular trabecular bone heights, indicating minimal bone loss and no resorption with well-defined root apices and periodontal ligament spaces ( Fig 4 , A ). Further examination using microcomputed tomography volumetric analysis on all 5 roots of the maxillary first molars showed no statistically significant difference between root volumes of the control (2.71 ± 0.41 mm 3 ) and the MOP sides (2.62 ± 0.50 mm 3 ) ( Fig 4 , B ), indicating that mini-implant–facilitated MOPs induced accelerated tooth movement without causing significant root resorption.
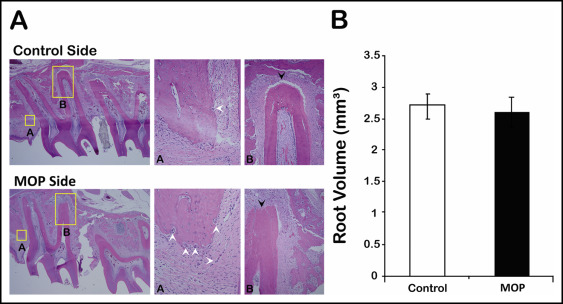
Although we found no difference in root volumes, closer examination of the bone surfaces showed more multinucleated osteoclasts in the MOP side compared with the control side ( Fig 4 , A , middle panels). To quantitatively evaluate bone resorption, the number of osteoclasts was determined after TRAP staining ( Fig 5 , A ). The MOP side showed significantly greater numbers of osteoclasts in the alveolar bone surrounding the maxillary first molars compared with the control side ( Fig 5 , A ). On average, there were 44% more osteoclasts in the total alveolar bone surrounding the first molars on the MOP side compared with the control side and 55% more osteoclasts on the pressure side of the alveolar bone on the MOP side compared with the control side ( Fig 5 , B ).
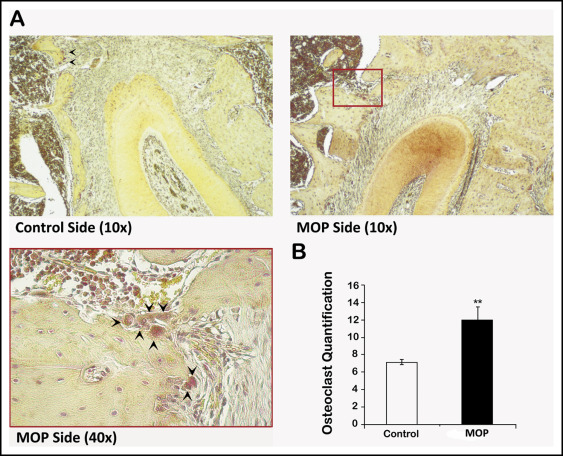
Bone remodeling occurs by inducing bone resorption as well as bone formation. Therefore, we also evaluated new bone formation. Hematoxylin and eosin analysis also showed that the MOP side had greater new bone formation as indicated by dark blue lines showing less-organized woven bone and increased bone metabolic activity, as opposed to the predominantly organized and mature bone of the control side ( Fig 6 , A ). New bone formation was quantified with hematoxylin and eosin by defining a fixed size of rectangle enclosing the alveolar bone of the maxillary first molar for each rat. The areas of new bone were measured, and the percentages of new bone formation were calculated using the equation: Σ (new bone area)/(total alveolar bone area). The MOP side (11.0% ± 4.7%) showed a statistically significant increase in new bone formation compared with the control side (6.2% ± 5.8%) ( Fig 6 , B ).
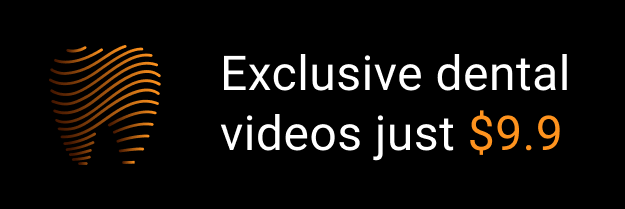