Introduction to Composite Restorations
The search for an ideal esthetic material for restoring teeth has resulted in significant improvements in esthetic materials and in the techniques for using these materials. Composites and the acid-etch technique represent two major advances in restorative dentistry.1–4 Adhesive materials that have strong bonds to enamel and dentin further simplify restorative techniques.5–10 The possibilities for innovative uses of esthetic materials are exciting and almost unlimited. Many of the specific applications of these materials are presented in Chapters 9 through 12; this chapter provides a general introduction to composites, the predominant direct esthetic restorative materials (Fig. 8-1).
This chapter primarily presents the properties and clinical uses of composite materials. Composites can be used in almost any tooth surface for any kind of restorative procedure. Naturally, certain factors must be considered for each specific application. The reasons for such expanded usage of these materials relate to the improvements in their ability to bond to tooth structure (enamel and dentin) and in their physical properties. The ability to bond a relatively strong material (composite) to tooth structure (enamel and dentin) results in a restored tooth that is well sealed and possibly regains a portion of its strength.11,12
Types of Esthetic Restorative Materials
Many esthetic restorative materials are available. To gain a full historical appreciation of the types of conservative esthetic materials that might be encountered, a representative list of tooth-colored materials is briefly reviewed. These materials are presented in greater detail in online Chapter 18, Biomaterials.
Ceramic Inlays and Onlays
The fused (baked) feldspathic porcelain inlay, an indirect ceramic restoration, dates from 1908, when Byram described several designs of tooth preparations for its use.13,14 Since the development of adhesive resin cements, interest in using feldspathic porcelain for inlays and onlays in posterior teeth (see examples in Chapter 11) and veneers in anterior teeth (see examples in Chapter 12) has been renewed.15–19 Many of these restorations are fabricated in a dental laboratory with materials and equipment similar to those used for other types of fused porcelain. Newer versions of ceramics, from which indirect restorations are either pressed or cast, also are available whose physical properties and ease of fabrication are much improved from classic feldspathic porcelain materials (see online Chapter 18). Sophisticated computer-aided design/computer-assisted machining (CAD/CAM) systems enable fabrication of ceramic restorations chairside, thus eliminating the need for impressions, temporary restorations, laboratory procedures and costs, and additional appointments (see Chapter 11 and online Chapter 18).20–22
Silicate Cement
Silicate cement, the first translucent restorative material, was introduced in 1878 in England by Fletcher.14 For more than 60 years, silicate cement was used extensively to restore caries lesions in anterior teeth. Silicate cement powder is composed of acid-soluble glasses, and the liquid contains phosphoric acid, water, and buffering agents. Although silicate cement is not used as a restorative material today, a practitioner still might encounter silicate restorations in older patients. Of particular interest is that glass ionomer materials are basically contemporary versions of silicate cements. The primary difference relates to the use of polyacrylic acid as opposed to phosphoric acid, rendering glass ionomers less soluble.
Silicate cement was recommended for small restorations in the anterior teeth of patients with high caries activity.23 By virtue of the high fluoride content and solubility of this restorative material, the adjacent enamel was thought to be rendered more resistant to recurrent caries. Although the average life of a silicate cement restoration was approximately four years, some of these restorations were reported to last for 10 years and longer in some patients.24,25
Acrylic Resin
Self-curing (chemically activated) acrylic resin for anterior restorations was developed in Germany in the 1930s.26 Early acrylic materials were disappointing because of their inherent weaknesses such as poor activator systems, high polymerization shrinkage, high coefficient of thermal expansion, and lack of wear resistance, all of which resulted in marginal leakage, pulp injury, recurrent caries, color changes, and excessive wear.26,27 It was not indicated for high-stress areas because the material had low strength and would flow under load. Its high polymerization shrinkage and linear coefficient of thermal expansion (LCTEs) caused microleakage and eventual discoloration at the margins as a result of percolation.26 Acrylic resin restorations are rarely used today but, as with silicate cement restorations, may be seen in older patients.
As a restoration, acrylic resin was most successful in the protected areas of teeth where temperature change, abrasion, and stress were minimal.28 It also was used as an esthetic veneer on the facial surface of Class II and IV metal restorations and for facings in crowns and bridges. A current, although limited, use of acrylic resin is for making temporary restorations in operative and fixed prosthodontic indirect restoration procedures requiring two or more appointments.
Composite
In an effort to improve the physical characteristics of unfilled acrylic resins, Bowen, of the National Bureau of Standards (now called the National Institute of Standards and Technology), developed a polymeric dental restorative material reinforced with inorganic particles.1,29 The introduction in 1962 of this filled resin material became the basis for the restorations that are generically termed composites. Basically, composite restorative materials consist of a continuous polymeric or resin matrix in which an inorganic filler is dispersed. This inorganic filler phase significantly enhances the physical properties of the composite (compared with previous tooth-colored materials) by increasing the strength of the restorative material and reducing thermal expansion.30 Composites possess LCTEs that are one-half to one-third the value typically found for unfilled acrylic resins and nearer to that of tooth structure. (See online Chapter 18 for details on composite components and properties.)
For a composite to have good mechanical properties, a strong bond must exist between the organic resin matrix and the inorganic filler. This bond is achieved by coating the filler particles with a silane coupling agent, which not only increases the strength of the composite but also reduces its solubility and water absorption.30,31
Macrofill (or Conventional) Composites
Macrofill composites were the first type of composites introduced in the early 1960s. Although these types of composite restorations are sometimes found in some older patients, they are no longer used in clinical practice. Macrofill composites generally contained approximately 75% to 80% inorganic filler by weight. The average particle size of conventional composites was approximately 8 µm.29 Because of the relatively large size and extreme hardness of the filler particles, macrofill composites typically exhibit a rough surface texture. (This characteristic can be seen in the scanning electron micrograph in Fig. 8-2.) The resin matrix wears at a faster rate than do the filler particles, further roughening the surface. This type of surface texture causes the restoration to be more susceptible to discoloration from extrinsic staining. Macrofill composites have a higher amount of initial wear at occlusal contact areas than do the microfill or hybrid types.
Microfill Composites
Microfill composites were introduced in the late 1970s. These materials were designed to replace the rough surface characteristic of conventional composites with a smooth, lustrous surface similar to tooth enamel. Instead of containing the large filler particles typical of the conventional composites, microfill composites contain colloidal silica particles whose average diameter is 0.01 to 0.04 µm. As illustrated in the scanning electron micrograph in Figure 8-3, this small particle size results in a smooth, polished surface in the finished restoration that is less receptive to plaque or extrinsic staining. Because of the greater surface area per unit volume of these microfine particles, however, microfill composites cannot be as heavily filled because of the significant surface area per unit of volume.31 Typically, microfill composites have an inorganic filler content of approximately 35% to 60% by weight. Because these materials contain considerably less filler than do conventional or hybrid composites, some of their physical and mechanical characteristics are inferior. Nonetheless, microfill composites are clinically highly wear resistant. Also, their low modulus of elasticity may allow microfill composite restorations to flex during tooth flexure, better protecting the bonding interface. This feature may not have any effect on material selection for Class V restorations in general, but it might make microfill composites an appropriate choice for restoring Class V cervical lesions or defects in which cervical flexure can be significant (e.g., bruxism, clenching, stressful occlusion).32
Glass Ionomer
Conventional Glass Ionomers
Glass ionomers were developed first by Wilson and Kent in 1972.33 Similar to silicate cements, their predecessors, the original glass ionomer restorative materials were powder/liquid systems. Glass ionomers have the same favorable characteristics of silicate cements—they release fluoride into the surrounding tooth structure, yielding a potential anti-cariogenic effect, and possess a favorable coefficient of thermal expansion.34,35 In contrast to silicate cements, which have phosphoric acid liquid, glass ionomers use polyacrylic acid, which renders the final restorative material less soluble.
Although conventional glass ionomers are relatively technique-sensitive with regard to mixing and insertion procedures, they may be good materials for restoration of teeth with root-surface caries because of their inherent potential anti-cariogenic quality and adhesion to dentin. Similarly, because of the potential for sustained fluoride release, glass ionomers may be indicated for other restorations in patients exhibiting high caries activity.36 Because of their low resistance to wear and relatively low strength compared with composite or amalgam, glass ionomers are not recommended for the restoration of the occlusal areas of posterior teeth. Glass ionomer cements also have been widely advocated for permanent cementation of crowns.
Resin-Modified Glass Ionomers
In an effort to improve the physical properties and esthetic qualities of conventional glass ionomer cements, resin-modified glass ionomer (RMGI) materials have been developed (Table 8-1). RMGIs are probably best described as glass ionomers to which resin has been added. An acid-base setting reaction, similar to that of conventional glass ionomer cements, is present. This is the primary feature that distinguishes these materials from compomers (see the next section). Additionally, the resin component affords the potential for light-curing, autocuring, or both. RMGIs are easier to use and possess better strength, wear resistance, and esthetics than do conventional glass ionomers. Their physical properties are generally inferior to those of composites, however, and their indications for clinical use are limited. Because they have the potential advantage of sustained fluoride release, they may be best indicated for Class V restorations in adults who are at high risk for caries and for Class I and II restorations in primary teeth that would not require long-term service.37
Important Properties of Composites
The various properties of composites should be understood for achieving a successful composite restoration. These properties generally require that specific techniques be incorporated into the restorative procedure, either in tooth preparation or in the application of the material. The various property factors are presented here, with additional information provided primarily in online Chapter 18 but also in Chapters 9 through 12.
Linear Coefficient of Thermal Expansion
The LCTE is the rate of dimensional change of a material per unit change in temperature. The closer the LCTE of the material is to the LCTE of enamel, the lower the chance for creating voids or openings at the junction of the material and the tooth when temperature changes occur. The LCTE of modern composites is approximately three times that of tooth structure.38 Bonding a composite to etched tooth structure reduces the potential negative effects as a result of the difference between the LCTE of the tooth structure and that of the material.
Wear Resistance
Wear resistance of contemporary composite materials is generally good. Although not yet as resistant as amalgam, the difference is becoming smaller.39,40 A composite restoration offers stable occlusal relationship potential in most clinical conditions, particularly if the occlusal contacts are shared with the contacts on natural tooth structure.
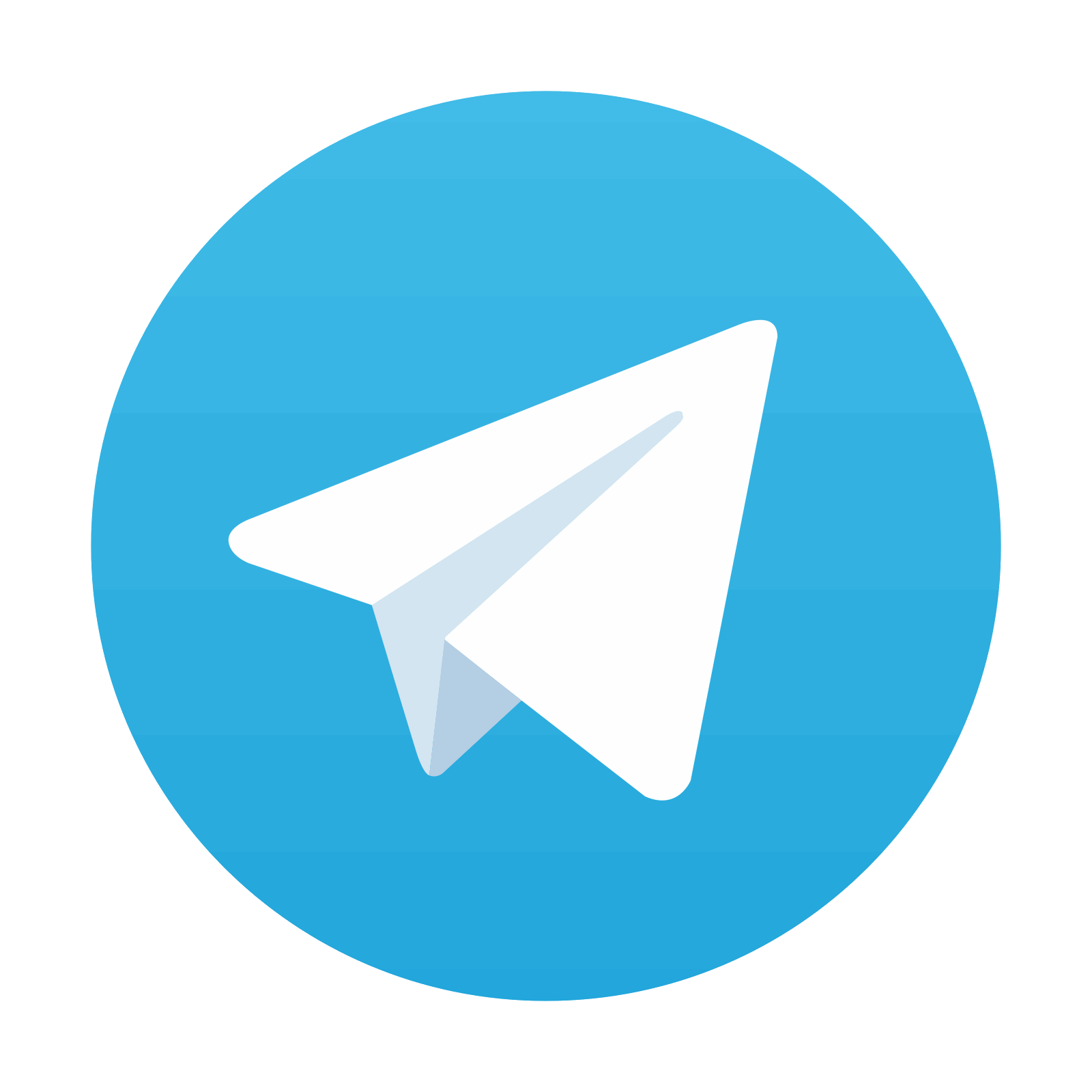
Stay updated, free dental videos. Join our Telegram channel

VIDEdental - Online dental courses
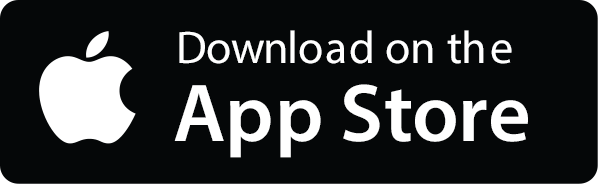
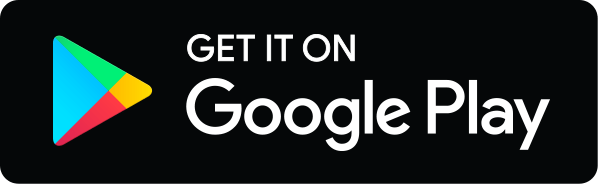