Chapter 6 Plaque-mediated diseases – dental caries and periodontal diseases
Relationship of plaque bacteria to disease: general principles
Preventative and curative regimens for caries and periodontal diseases would be more precise if the particular microorganism(s) causing the disease could be identified. Historically, for any microbe to be considered responsible for a given condition, Koch’s postulates were applied:
Relationship of plaque bacteria to disease: contemporary perspectives
Recently, an alternative hypothesis has been proposed (the ‘Ecological Plaque Hypothesis’) that reconciles the key elements of the earlier two hypotheses. In brief, the ecological plaque hypothesis proposed that the organisms associated with disease may also be present at sound sites, but at levels too low to be clinically relevant. Disease is a result of a shift in the balance of the resident microflora due to a response to a change in local environmental conditions (Fig. 6.1). For example, repeated conditions of low pH in plaque following frequent sugar intake favours the growth of acid-producing and acid-tolerating species that cause caries, while the inflammatory response to dental plaque accumulation around the gums results in an increased flow in gingival crevicular fluid that can provide a source of different nutrients that favours the growth of the proteolytic and obligately anaerobic bacteria that predominate in periodontal disease. Importantly, therefore, prevention can be achieved not only by the direct inhibition of the causative bacteria but also by the ‘removal’ or ‘neutralization’ of the forces that drive the selection of these organisms. These theories will be discussed later in more detail.
Relationship of plaque bacteria to disease: implications for study design
Two types of epidemiological survey have been designed to determine the role of plaque bacteria in human disease (Fig. 6.2). In cross-sectional surveys, predetermined surfaces in a population are sampled at a single time point, and the plaque microflora is related to the caries or periodontal status of the site at that time. However, with this type of study, it cannot be determined for certain whether the species that are isolated at the time when disease is diagnosed caused the decay or inflammation, or arose because of it. Only ‘associations’ can be derived from this study design, but they have the advantage that large numbers of sites/people can be analysed, and different patient groups, age groups, tooth surfaces, diets, intervention strategies, etc. can be compared. Likewise, it cannot be determined whether the site, at the time of sampling, was progressing, arrested, or healing, and each phase may have a different microflora. In order to overcome these difficulties, longitudinal studies have been designed in which initially clinically healthy sites are sampled at regular intervals over a set time period (Fig. 6.2). Sites are selected on the basis of previous epidemiological surveys from which it can be predicted that a statistically relevant number of sites should suffer disease within the time span of the study. The microflora can then be compared: (a) before and after the diagnosis of disease, and (b) between those sites that became diseased and those that remained healthy throughout the study, so that true cause-and-effect relationships can be established. A consequence of this approach is that, for practical reasons, resources permit that only a limited number of individuals can be followed for prolonged periods.
Superimposed on the challenges of study design outlined above are those associated with the sampling and microbiological analysis of plaque (Ch. 4). The plaque microflora is diverse, and disease is not due to colonization by exogenous (non-oral) species (such events might be more easy to recognise), but to changes in the relative proportions of members of the resident microflora. Traditional culture techniques can recover, at present, only about 50% of the microflora (Ch. 3), and so the role of potentially significant organisms could be under-estimated or missed using these approaches. Furthermore, irrespective of the methods used, there are wide inter-subject variations in the composition of the plaque microflora (Ch. 5), so that when data are averaged from numerous individuals, clear associations between bacteria and disease can be obscured. Similarly, the composition of dental plaque can vary over relatively small distances. This can pose difficulties during longitudinal studies since, ideally, the same location should be resampled on each occasion. In addition, there is the possibility that regular sampling could distort the subsequent re-establishment of plaque. Despite these problems of study design and methodology, much progress has been made, and the major findings will now be discussed in the following sections.
Dental caries
In industrialized societies, caries affects the vast majority of individuals. Caries of enamel surfaces is particularly common up to the age of 20 years, whereas, in later life, root-surface caries is an increasing problem due to gingival recession exposing the vulnerable cementum to microbial colonization. Dental caries can be defined as the localized destruction of the tissues of the tooth by bacterial fermentation of dietary carbohydrates. Cavities begin as small demineralized areas below the surface of the enamel (Fig. 6.3); once enamel has been affected, caries can progress through the dentine and into the pulp (Fig. 6.4). Demineralization of the enamel is caused by acids, particularly lactic acid, produced from the microbial fermentation of dietary carbohydrates. Lesion formation involves dissolution of the enamel and the transport of the calcium and phosphate ions away into the surrounding environment. The initial stages of caries are reversible and remineralization can occur, particularly in the presence of fluoride.
Evidence for caries as an infectious disease
In his famous Chemico-Parasitic Theory, Miller (1890) suggested that oral bacteria converted dietary carbohydrates into acid which solubilized the calcium phosphate of the enamel to produce a caries lesion. Although Clarke isolated an organism (which he called Streptococcus mutans) from a human caries lesion in 1924, definitive proof for the causative role of bacteria came only in the 1950s and 1960s following experiments with germ-free animals. Pioneering experiments showed that germ-free rats developed caries when infected with bacteria described then as enterococci. Evidence for the transmissibility of caries came from studies on hamsters. Caries-inactive animals had no caries even when fed a highly cariogenic (i.e. sucrose-rich) diet. Caries only developed in these animals when they were caged with or ate the faecal pellets of a group of caries-active hamsters. Further proof came when streptococci, isolated from caries lesions in rodents, caused rampant decay when inoculated into the oral cavity of previously caries-inactive hamsters, while animals treated with appropriate antibiotics did not develop caries. The importance of diet became apparent when the colonization and production of caries by most streptococcal populations occurred only in the presence of sucrose.
Mutans streptococci can cause caries of smooth surfaces, as well as in pits and fissures, in hamsters, gerbils, rats and monkeys fed on cariogenic diets, and these are the most cariogenic group of bacteria found. Other bacteria, including members of the mitis-, anginosus-, and salivarius-groups of streptococci, Enterococcus faecalis, Actinomyces naeslundii, A. viscosus and lactobacilli, can also produce caries under conducive conditions in some animals, although the lesions are usually restricted to fissures. The ability of mutans streptococci to cause dental caries has also been confirmed during vaccination studies. Immunization of rodents or primates fed a cariogenic (high sugar) diet with whole cells or specific antigens of S. mutans and S. sobrinus led to a reduction in the number of these organisms in plaque and a decrease in the number of caries lesions when compared with control animals. Subsequent experiments using defined mutants helped to confirm the importance of traits such as the production of intracellular and extracellular polysaccharides from sucrose to the cariogenic potential of mutans streptococci (see later; Table 6.5).
Aetiology of human enamel caries
Natural history of dental caries
Cavitation is the final stage of enamel caries (Fig. 6.4); it is preceded by a clinically-detectable small lesion, known as a ‘white spot’, and before that by sub- surface demineralization, which can only be detected by histological techniques (Fig. 6.3). Not all white-spot lesions progress to cavitation; in some studies only about half of these early lesions penetrated the dentine after 3–4 years. White spot lesions do not just arrest, but can even remineralize, a process that is enhanced by fluoride. Enamel caries often occurs in teeth shortly after eruption (hence, its historical association with young people), and some teeth and surfaces are more vulnerable than others. The prevalence of caries is highest on the occlusal surfaces of first and second molars, and lowest on the lingual surfaces of mandibular teeth. The risk to approximal surfaces is intermediate to those described above. With the reduction in the incidence of dental caries in the young, it is predicted that caries risk for some surfaces will now extend over the life-time of an individual. If confirmed, this trend would have important implications for caries prevention strategies. Some individuals are more caries prone than others, and this may be related to their diet (e.g. frequency of sugar intake), lack of optimum saliva flow (e.g. flow is severely reduced in xerostomia patients), and low exposure to fluoride.
Microbiology of enamel caries
Buccal and lingual smooth surfaces are easy to clean and generally suffer from decay only rarely. However, they are easy to study for experimental purposes, both in terms of clinical diagnosis and in plaque sampling. Higher proportions (10–100 fold) of mutans streptococci have been found on white-spot lesions on smooth surfaces compared with adjacent sound enamel. As stated earlier, such an association does not prove a causal relationship and the actual proportions of mutans streptococci are often low. Suspensions of plaque from white-spot lesions produce a lower pH minimum and a faster rate of pH-fall than plaque from sound enamel.
Fissures on occlusal surfaces (Figs 2.2 and 5.10) are the most caries-prone sites. Caries can develop rapidly on these surfaces, and it is at these sites that the strongest association between mutans streptococci and dental decay has been found. In one cross-sectional study, 71% of carious fissures had viable counts of mutans streptococci that were >10% of the total cultivable plaque microflora, whereas 70% of the fissures that were caries free at the time of sampling had no detectable mutans streptococci. An inverse relationship between mutans streptococci and S. sanguinis is frequently observed. In a longitudinal study of North American children, the proportions of mutans streptococci, S. sanguinis and lactobacilli were monitored before and at the time of caries development in occlusal fissures. The subjects were divided into several groups according to their previous caries experience and to their caries activity during the study. The proportions of mutans streptococci increased significantly at the time of diagnosis of most lesions. However, mutans streptococci were only a minor component of the plaque from five fissures which became carious. Counts of lactobacilli were significantly higher at these sites and, it was concluded, that these were probably responsible for lesion formation. A subsequent longitudinal study confirmed these findings, and demonstrated an even stronger relationship between mutans streptococci and caries initiation (Table 6.1), whereas lactobacilli, when present, were strongly associated with sites requiring restoration. Thus, there was a strong correlation between mutans streptococci and fissure decay, although lesions could also develop at some sites in the apparent absence of this group of bacteria. There were also surfaces where mutans streptococci appeared to persist in moderately high numbers without any evidence of detectable caries.
Table 6.1 Mean proportions of mutans streptococci (MS) and lactobacilli (L) on teeth in schoolchildren (7–8 years old) who remained caries free or who developed a caries lesion during a longitudinal study
A major prospective study of young Swiss children (7–8 years) specifically examined the question as to whether colonization by mutans streptococci was a risk factor for caries in fissures (and on smooth surfaces). Both fissures and smooth surfaces of first permanent premolars that suffered demineralization without cavitation were heavily colonized with mutans streptococci (104-105 colony forming units [CFU]/ml of sample) around 12–18 months prior to the clinical diagnosis of the lesion. The proportions of mutans streptococci appeared to increase markedly 6–9 months prior to lesion detection to reach 11–18% and 10–12% of the total streptococcal microflora of fissures and smooth surfaces, respectively. This study demonstrated that colonization and an increase in proportions of mutans streptococci preceded lesion formation by about six months. As with other studies of caries, however, several sites had high counts of mutans streptococci (>20% of the total streptococcal count) but no evidence of caries. Larger lesions (with cavitation) were found only at a relatively few sites. In five out of six carious fissures, the median count of mutans streptococci rose from 102 to >104 CFU/ml sample around 12 months prior to lesion detection; this represented a final mean proportion of 18% of the total streptococcal count. The remaining carious fissure had no detectable mutans streptococci at any time during the study, again illustrating that species other than mutans streptococci can play a role in lesion formation on occasions. An additional feature of the design of this study was that some sites were diagnosed with a lesion which subsequently remineralized. Some of these sites had levels of mutans streptococci greater than 20% of the streptococcal microflora, and yet the lesion remineralized within 12 months. During this period, the levels of mutans streptococci fell markedly between 6 and 9 months prior to the diagnosis of the reversal. Overall, however, this study provided convincing data on the important role of mutans streptococci in the initiation of dental caries.
A challenge for studies of approximal surfaces lies with the difficulty in accurately diagnosing early lesions, and with the fact that plaque samples are inevitably removed from the whole interproximal area, including that overlying sound enamel as well as carious enamel. The microflora can vary markedly at different sites around the contact area between teeth, irrespective of whether a lesion is developing, so that specific associations can sometimes be obscured. Early cross-sectional studies found a positive correlation between elevated levels of mutans streptococci and lesion development. Many of these studies, however, were limited in scope, and only monitored a few types of microorganism, and sometimes only mutans streptococci. In order to combat this, a limited number of longitudinal studies have been performed. A less clear cut association was found in a survey of English schoolchildren, aged 11–15 years. Mutans streptococci could be found in high numbers prior to demineralization at a number of sites, but lesions also appeared to develop on occasions in the apparent absence of this group of bacteria. Mutans streptococci were also present at other sites in equally high numbers for the duration of the study without any diagnosis of caries. There was evidence that the isolation frequency and proportions of mutans streptococci increased after, rather than before, the first radiographic detection of some lesions, especially in those that progressed deeper into the enamel, suggesting that the composition of the microflora might shift as the lesion progresses through the tooth (microbial succession; Ch. 4).
Similar findings were found in a study of Dutch army recruits, aged 18–20 years. Mutans streptococci were isolated from 40% and 86% of sites from caries-free and caries-active recruits, respectively. Marked differences in the distribution of individual species were found; S. mutans (serotype c) strains were isolated from both groups whereas S. sobrinus (serotype d) was recovered almost exclusively from caries-active recruits (Table 6.2). The prevalence of the combined species of mutans streptococci also showed a direct but not unique correlation with the progression of a lesion into the dentine. Again, relatively high proportions of mutans streptococci persisted at some tooth surfaces without caries progression while on occasions caries could develop in their apparent absence.
Table 6.2 Prevalence of mutans streptococci at approximal tooth surfaces with and without caries progression
A major limitation of traditional culture approaches is that around 50% of the oral microflora cannot as yet be grown in the laboratory, and so molecular methods have been developed to characterize the complex communities in the mouth (Chs 3 and 4). A more diverse microflora has been reported when these methods have been applied to sites with caries, and novel taxa have been described. One study confirmed the relationship of S. sanguinis with sound enamel and S. mutans and lactobacilli with caries lesions, but additionally found Actinomyces gerencseriae and other Actinomyces spp. to be implicated in caries initiation and Bifidobacterium spp. with advanced lesions. Another study used molecular approaches to investigate the microbial diversity of plaque from teeth with lesions at different stages of disease. They found 10% of subjects with rampant caries in the secondary dentition did not have detectable levels of S. mutans. In lesions where mutans streptococci could not be detected, there were high levels of lactobacilli, low pH tolerating non-mutans streptococci and Bifidobacterium spp.. High levels of Actinomyces species and non-mutans streptococci were found in early (white spot) lesions, while mutans streptococci and lactobacilli, together with Propionibacterium and Bifidobacterium spp. dominated advanced (deep dentine) lesions. The data supported the ecological plaque hypothesis in that shifts in the bacterial composition of plaque were seen between healthy sites and those with lesions of increasing severity, and the organisms associated with caries were all acid producing species.
‘Nursing-bottle’ caries is the extensive and rapid decay of the maxillary anterior teeth associated with the prolonged and frequent feeding of young infants with bottles or pacifiers containing formulas with a high concentration of fermentable carbohydrate. Plaque bacteria receive an almost continuous provision of substrates from which they can make acid. Such prolonged conditions of low pH are conducive, and indeed selective, for mutans streptococci and lactobacilli, and proportions of mutans streptococci in plaque can reach >50% of the microflora. Other studies have reported elevated levels of S. mutans, Actinomyces israelii, lactobacilli, Veillonella spp. (presumably reflecting high concentrations of lactate; see Ch. 5), and C. albicans (which also tolerates acidic conditions) in nursing caries lesions.
Microbiology of root surface caries
The reduction in enamel caries in industrialized societies has resulted in large proportions of the public retaining their teeth into later life. Gingival recession occurs in old age exposing the susceptible cementum surface of the root to microbial colonization; root surfaces can also become exposed due to mechanical injury or to periodontal surgery (e.g. following scaling and root planing). These cementum surfaces are even more vulnerable than enamel to demineralization by plaque acids. The prevalence of root surface caries increases with age; approximately 60% of individuals aged 60 years or older now have root caries or fillings.
Human studies
Subsequent studies have tended to confirm a stronger association between mutans streptococci and lactobacilli with root surface caries. In a major longitudinal survey in Canada, although no direct correlation between specific bacteria and root caries was found, the presence of mutans streptococci and lactobacilli on root surfaces was predictive for the subsequent development of a lesion. Other studies have attempted to subdivide the lesions into initial (or ‘soft’) and advanced (or ‘hard’). Several groups reported higher proportions (often around 30% of the total cultivable microflora) of mutans streptococci at the initial lesion (Table 6.3), sometimes in association with lactobacilli. Lactobacilli have occasionally been found at arrested (hard, black-coloured) lesions. Following improvements to the classification of Actinomyces spp., the predominant species isolated from infected dentine of active root caries lesions are A. israelii > A. gerencseriae > A. naeslundii > A. odontolyticus > A. georgiae.
Table 6.3 Mean percentage viable counts (and percentage isolation frequencies in parentheses) of some plaque bacteria from root surfaces, with and without caries
The recent application of more sophisticated approaches to sampling plaque has given new insights into the relationship between the composition of the microflora and lesion development. One study used a specially designed device to lift plaque from discrete areas directly into reduced transport fluid to preserve the viability of obligately anaerobic bacteria. The caries status of these precise sampling sites was then assessed by a variety of sensitive techniques including contact microradiography, and light and electron microscopy. In this way, the surface directly beneath the plaque that had been sampled could be reliably classified as being a sound, active or arrested carious root lesions. Regardless of the degree of mineralization, the microflora from these root surfaces was more diverse than had previously been reported, and resembled that associated with gingivitis (see later). On all surfaces, Actinomyces were the predominant group of bacteria, especially A. naeslundii, A. odontolyticus and A. gerencseriae. Arrested lesions had significantly lower numbers of bacteria than either sound surfaces or active lesions. Gram negative species formed around 50% of the microflora on sound and active carious surfaces, with Prevotella spp. (particularly P. nigrescens) being highly prevalent. Other Gram negative bacteria, including Capnocytophaga spp., Campylobacter spp. and Leptotrichia buccalis, were preferentially isolated from plaque overlying active lesions. This may be because a number of these species are sufficiently saccharolytic to demineralize cementum and dentine, while others are proteolytic, and could hydrolyze the dentine collagen matrix. These data suggest that (a) caries initiation on root surfaces can have a polymicrobial aetiology, and (b) bacterial succession occurs during the development of root surface lesions.
Molecular and culture approaches have been used to characterize the microbial community of carious dentine. The predominant bacteria recovered by anaerobic cultivation included a novel Propionibacterium spp., Olsenella profusa and Lactobacillus rhamnosus. Even more novel taxa were detected when DNA was extracted directly from the lesions, amplified by PCR, and bacterial identification was based on 16S rRNA gene sequence analysis (Chs 3 and 4). Forty four taxa were detected by the molecular approach alone, of which 31 had never been described previously (although they were generally close relatives of cultivable species). The predominant taxa identified by the molecular methods included S. mutans, lactobacilli and Rothia dentocariosa.
Bacterial invasion of dentine and root canals
Scaling and root planing can predispose some root surfaces to bacterial invasion by exposing dentine tubules; in addition, bacteria may lodge in injured pulp following a transient bacteraemia (anachoresis) (Fig. 6.5).
The microflora found in the dentine and pulp of periodontally-diseased human teeth is also diverse and may be derived predominantly from the subgingival area. Numerous Gram positive and Gram negative species have been identified; some are more prevalent in the dentine (e.g. A. odontolyticus, Bifidobacterium spp.), some predominate in the pulp (e.g. black-pigmented anaerobes), while others are found equally at both sites (e.g. A. naeslundii, Veillonella spp., F. nucleatum). Dentine collagen is denatured and modified during the caries process, and becomes more susceptible to breakdown by non-specific proteases, and this explains the presence of both acidogenic and proteolytic bacteria.
Once bacteria are in the pulp, inflammation can occur which may result eventually in necrosis of the root canal. A further consequence is that microorganisms can invade and destroy tissue surrounding the apex of the root, producing a spreading or localized infection (Fig. 6.5). Diverse mixed culture of bacteria are cultured, including black-pigmented anaerobes (Prevotella intermedia, Prevotella melaninogenica, Porphyromonas endodontalis, P. gingivalis), and Prevotella dentalis, Campylobacter sputorum, Eubacterium spp. and Parvimonas (formerly Peptostreptococcus) spp.. Some of these species (P. endodontalis, P. dentalis) are found almost exclusively in infected root canals and abscesses of endodontal origin. Another study of necrotic pulps found Propionibacterium, Eubacterium and Fusobacterium spp. to be the predominant bacteria, with Bifidobacterium, Lactobacillus, Actinomyces and Veillonella spp. as minor components. Certain bacterial combinations are associated with endodontic clinical symptoms, e.g. Parvimonas micra (formerly Peptostreptococcus micros), Porphyromonas and Fusobacterium are linked with pain.
The treatment of infections of the root canal (endodontics; Ch. 7) involves the removal of infected and dead tissue both mechanically and by irrigation, sometimes accompanied by treatment with antimicrobial agents to reduce the microbial community to a level where the cavity can be restored effectively.
Microbiological tests to predict caries activity
Microbiological tests have been used in an attempt to predict sites or individuals at risk of caries. These usually involve the estimation of the numbers of mutans streptococci in either plaque or saliva samples by plate counts in the laboratory, or by semi-quantitative, commercially-available, chair-side tests. There is now the promise of rapid molecular methods to detect cariogenic bacteria. Although a strong positive correlation has been found between levels of mutans streptococci and caries experience on a group or population level, the association is less clear cut for individuals. This is not surprising given that caries is a multi-factorial disease (Fig. 1.1), and clinical studies indicate that the relationship between mutans streptococci and caries is not absolute.
Pathogenic determinants of cariogenic bacteria
Determinants of cariogenicity are often linked to sugar metabolism (Table 6.4). Mutants defective in various aspects of sucrose metabolism have been compared in terms of their relative cariogenicity in animal models (Table 6.5). Strains of S. mutans defective in insoluble glucan synthesis were unable to colonize teeth as effectively as the parent strains, and caused fewer smooth surface caries lesions in an animal (rat) model. The synthesis of intracellular storage compounds enables S. mutans to continue making acid even in the absence of dietary carbohydrates. Mutants defective in intracellular polysaccharide (IPS) synthesis produced fewer caries lesions than parent strains when inoculated in pure cultures in gnotobiotic rodents. Mutants of S. mutans defective in either lactic dehydrogenase or fructosyltransferase (Ch. 4) activity are also markedly less cariogenic in rat caries models while, in contrast, fructanase was shown not to be essential for virulence.
Table 6.4 Characteristics of mutans streptococci that contribute to their cariogenicity
Property | Comment |
---|---|
Sugar transport | High and low affinity transport systems operating over a wide range of conditions to ensure substrate uptake, even under extreme environments, e.g. low pH. |
Acid production | An efficient glycolytic pathway rapidly producing low terminal pH values in plaque. |
Aciduricity | Cells have specific biochemical attributes enabling them to survive, metabolize and grow at low pH values. |
Extracellular polysaccharide (EPS) production | EPS contributes to the plaque matrix, consolidates attachment of cells, and may localize acidic fermentation products. |
Intracellular polysaccharide (IPS) production | IPS utilization allows acid production to continue in the absence of dietary sugars. |
Table 6.5 The use of mutants of mutans streptococci to determine traits linked to cariogenicity
Trait | Property of mutant | Effect on cariogenicity* |
---|---|---|
glucosyltransferase** | decreased colonization and plaque formation | reduced |
fructosyltransferase | loss of extracellular fructan | none |
fructanase | no breakdown of extracellular fructans | none |
IPS production | no intracellular glycogen | reduced |
antigen 1/11 | decreased ability to adhere | none |
enzyme II (PTS) | decreased sucrose transport | none |
lactic dehydrogenase | no lactic acid production | reduced |
“aciduricity”*** | reduced tolerance of low pH | reduced |
* If a mutation did not lead to a reduction in caries, it does not necessarily mean that the trait is not important in cariogenicity. It may reflect the fact that the particular trait is not essential for caries in an animal model; also, mutans streptococci often have more than one mechanism for a particular function, e.g. adherence.
** mutations in gtfB and gtfC, but not in gtfD, led to a reduction in cariogenicity.
*** this mutant was not fully characterized.
Distinctive characteristics of cariogenic bacteria are:
Few oral bacteria are able to tolerate acidic conditions for prolonged periods, but most strains of mutans streptococci and lactobacilli are not only able to remain viable (survive) at a low pH, but are able to continue to metabolize and multiply, i.e. they are acidogenic (strongly acid producing) and aciduric (acid-loving). Microbial survival in acidic environments depends on the cell maintaining a favourable intracellular pH despite sharp fluctuations in external pH (Ch. 4), and S. mutans achieves this by a number of specific mechanisms (Table 4.8). Some strains of other species also display an acid tolerant phenotype, including members of the anginosus-, mitis- and salivarius-groups of streptococci, while there can be variation in acid tolerance even among strains of S. mutans.
Re-evaluation of the microbial aetiology of dental caries
A consistent feature of most of the clinical studies already described has been the occasional but consistent finding of carious sites from which no mutans streptococci can be isolated. This suggests that bacteria other than mutans streptococci can make a contribution to demineralization. Bacteria isolated from such plaque samples have been compared in their ability to lower the pH of laboratory media. While strains of S. mutans produced a final pH in the region of 3.95–4.10, a range of other streptococci achieved terminal values of pH 4.05–4.50. These strains are often numerically dominant, and belong to the mitis-group (especially S. mitis, S. oralis and S. gordonii), salivarius-group and anginosus-group of streptococci. Similarly, when the rates of acid production were compared, mutans streptococci were generally faster at low environmental pH values, but other streptococci had greater rates at pH 7.0 and pH 6.0. Such findings reinforce the view that although mutans streptococci are clearly key causative organisms in enamel caries, other bacteria can contribute to, and modulate, the strength of the cariogenic challenge at a site. The converse situation is also not uncommon, where mutans streptococci are found in high numbers but in the apparent absence of any demineralization of the underlying enamel. This may be due to the presence of lactate-consuming species (e.g. Veillonella), or to the production of alkali at low pH (e.g. ammonia production by urease or arginine deiminase activities of bacteria such as S. salivarius and S. sanguinis, respectively).
These findings allow a model to be constructed to explain the changes in the ecology of dental plaque that lead to the development of a caries lesion. Cariogenic bacteria may be found naturally in dental plaque, but at neutral pH, these organisms are weakly competitive and are present only as a small proportion of the total plaque community. In this situation, with a conventional diet, the levels of such potentially cariogenic bacteria are clinically insignificant, and the processes of de- and remineralization are in equilibrium. If the frequency of fermentable carbohydrate intake increases, then plaque spends more time below the critical pH for enamel demineralization (approximately pH 5.5; Fig. 2.3). The effect of this on the microbial ecology of plaque is two-fold. Conditions of low pH favour the proliferation of mutans streptococci and lactobacilli, and tip the balance towards demineralization. Greater numbers of mutans streptococci and lactobacilli in plaque result in more acid being produced at even faster rates, thereby enhancing demineralization still further. Other bacteria could also make acid under similar conditions, albeit at a slower rate, but would contribute to the initial stages of demineralization, or could cause lesions in the absence of other (more overt) cariogenic species in a susceptible host. If aciduric species were not present initially, then the repeated conditions of low pH coupled with the inhibition of competing organisms might increase the likelihood of colonization by mutans streptococci or lactobacilli. This sequence of events would account for the lack of total specificity in the microbial aetiology of caries and explain the pattern of bacterial succession observed in many clinical studies. This model forms the basis of the ‘ecological plaque hypothesis’ (Fig. 6.6). In this hypothesis, caries is a consequence of changes in the natural balance of the resident plaque microflora brought about by an alteration in local environmental conditions (e.g. repeated conditions of high sugar and low plaque pH). The hypothesis also acknowledges the dynamic relationship that exists between the microflora and the host, so that the impact of alterations in key host factors (such as saliva flow) on plaque composition is taken into account. This is of great significance for caries prevention since implicit in the hypothesis is the concept that disease can be controlled not only by targeting directly the putative pathogens (e.g. by inhibition of mutans streptococci by antimicrobial agents) but also by interfering with the factors that are driving the deleterious shifts in the balance of the microflora (e.g. lowering the acid challenge by reducing the frequency of sugar intake, or by promoting the use of snacks containing sugar substitutes). These, and other caries preventive strategies, will be discussed in a later section.
The ecological plaque hypothesis also defines aetiological agents in a different way. Rather than just focussing on the role of particular named organisms in disease, the ecological plaque hypothesis attempts to define the involvement of microbes on the basis of their properties or functions. Thus, cariogenic bacteria are acidogenic and aciduric; such properties will not be unique to a single species in the same way that toxin production can be specific to a medical pathogen such as Clostridium tetani (the aetiological agent of tetanus), and could vary among strains of a particular species. Indeed, there will be a spectrum of pathogenic potential among many species of plaque bacteria and even among strains within a species. In general, however, the organisms that are optimally equipped biochemically to be acidogenic and aciduric belong to the mutans streptococci (these strains also produce glucans that contribute to the plaque matrix, and they store fermentable intracellular polysaccharides (IPS) produced from sucrose) and lactobacilli, and these organisms are consistently isolated in elevated numbers from caries lesions, but other strains of bacteria will contribute to a degree that is proportional to their acidogenic/aciduric phenotype.
Further reading – dental caries
Aas JA, Griffen AL, Dardis SR, et al. Bacteria of dental caries in primary and permanent teeth in children and young adults. J Clin Microbiol. 2008;46:1407-1417.
Becker MR, Paster BJ, Leys EJ, et al. Molecular analysis of bacterial species associated with childhood caries. J Clin Microbiol. 2002;40:1001-1009.
Bowden GHW. The microbial ecology of dental caries. Microb Ecol Health & Dis. 2000;12:138-148.
Brailsford SR, Shah B, Simons D, et al. The predominant aciduric microflora of root-caries lesions. J Dent Res. 2001;80:1828-1833.
Burne RA. Oral streptococci….products of their environment. J Dent Res. 1998;77:445-452.
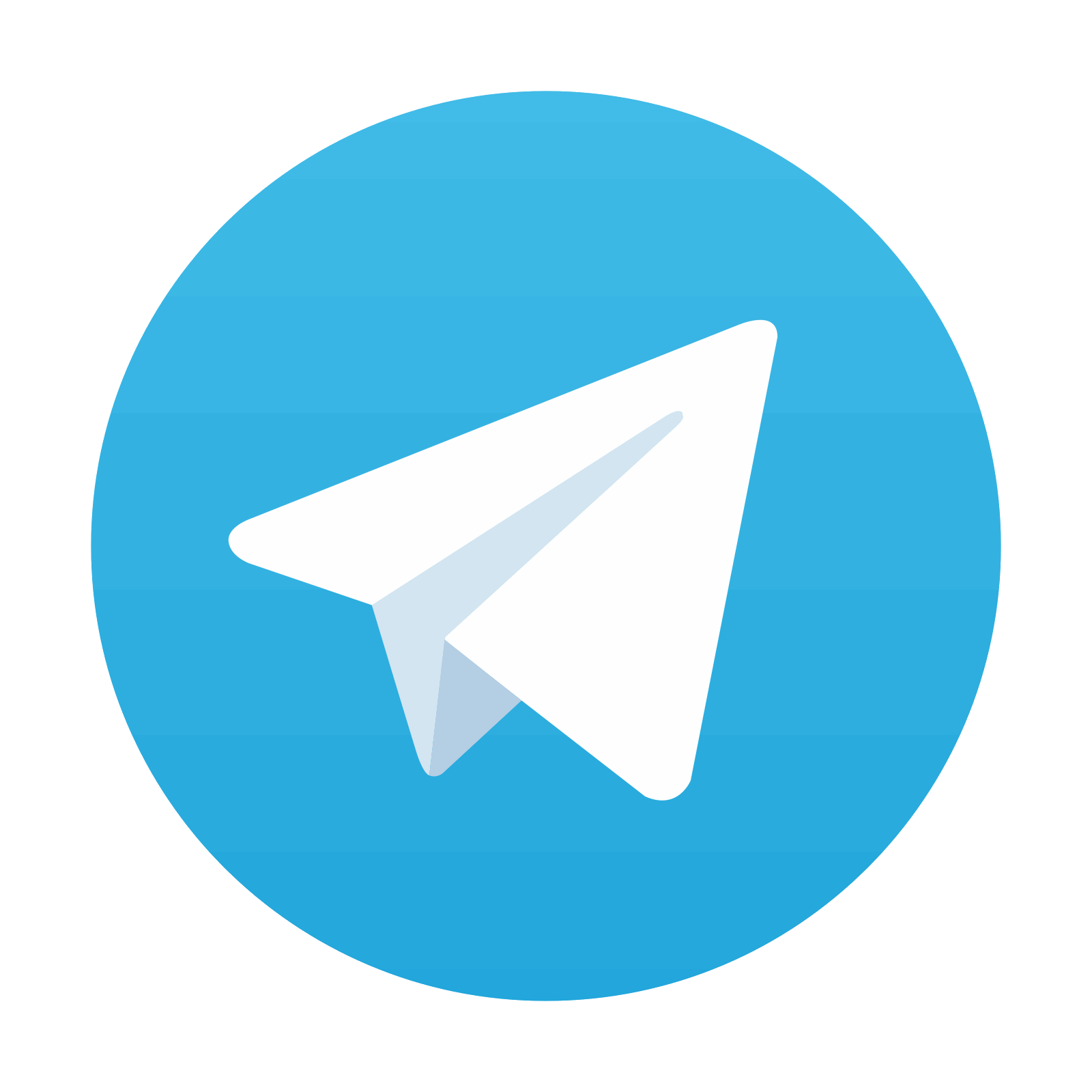
Stay updated, free dental videos. Join our Telegram channel

VIDEdental - Online dental courses
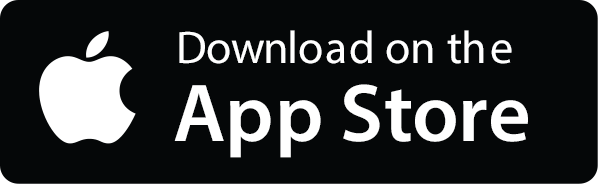
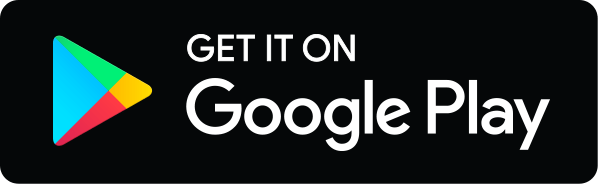