5
The Future of Cone Beam Computed Tomography and 3D Imaging in Orthodontics
Computed tomography (CT) is the cornerstone of the modern radiology department, often the first and only examination required for diagnosis, treatment planning, or follow-up for medical and surgical diseases. The scans depict bone and soft tissue structures, often after administration of oral enteric and intravenous iodinated contrast agents. CT was adopted and has become available widely for many biomedical disciplines, especially for oromaxillofacial imaging and otolaryngology, neuroimaging, orthopedic, cardiovascular, thoracic, abdominopelvic, radiotherapy, and whole body applications. CT scanning technology has evolved with many generations of advances from the slow mechanical systems of the mid-1970s to the current state-of-the-art scanners that can freeze cardiac motion with subsection 3D acquisitions (Kalender, 2011).
For orthodontics, CT imaging more recently has been adopted based on the development of compact inexpensive specialized systems that employ cone beam geometry for examinations limited to the oromaxillofacial structures (Merrett et al., 2009; Kapila et al., 2011; Van Vlijmen et al., 2012). Similar scanners are used for otolaryngology (Hodez et al., 2011), dental implant, oral surgery, prosthetics, and general dentistry applications (Scarfe & Farman, 2008). Chapter 2 provides a historical perspective on the evolution and current status of this technology for applications in dentistry and specifically in orthodontics.
This chapter evaluates the technologies that will influence the future of CT in the next several years. There are several limitations of current scanners that can be overcome using new technology that already is available in the laboratory or for some specialized applications. To a large degree, the market for CT scanners will determine how quickly the translation of new technology into clinical practice will take place.
In a typical hospital radiology setting, 16- and 64-slice scanners are the norm. These multirow detector spiral CT scanners can acquire and reconstruct several thousand slices from a whole body acquisition in less than 2 minutes. A single CT scanner can be used to examine dozens of patients in a single day with a variety of protocols that solve complex diagnostic problems of cancer, cardiopulmonary disease, infection, trauma, and others. Most large hospitals in the United States have several CT scanners in the radiology department, with another (e.g., virtual simulator) in the radiotherapy department.
The technology improvements that motivated the expanded use of CT for whole body applications have not ceased. Future improvements in CT scanning will include new X-ray source, detector, and computational enhancements.
Fundamentally, CT scanning is used to delineate bone and soft tissue. The X-ray attenuation differences at the interface between air and soft tissue as well as between soft tissue structures and bone are high, facilitating post-processing extraction of soft tissue surfaces and bony surfaces for 3D visualization. The soft tissue discrimination of CT is orders of magnitude greater than that found with ordinary radiographs, largely as a result of X-ray collimation, high dynamic range detectors, and reconstruction methods (Hsieh, 2009; Buzug, 2010).
X-Ray Sources
Virtually all CT scanners used for biomedical applications employ thermionic emission tungsten target X-ray tubes. These have many similar characteristics to the Crookes tube technology used by Röntgen in 1896 when X-rays were discovered. Despite numerous improvements in X-ray source technology, the fundamental physics of the source remains the same.
In the future, new X-ray source technologies may be adopted. Among the most promising are carbon nanotube (CNT) sources that employ field emission (Xu et al., 2011; Figure 5.1). As cold cathode devices, there is no filament (Lee et al., 2011). They have no moving components and CNT sources can be constructed with a variety of geometries, are significantly less restrictive than the conventional X-ray tube (Wang et al., 2011), and already are in use for animal micoCT (Figure 5.2).
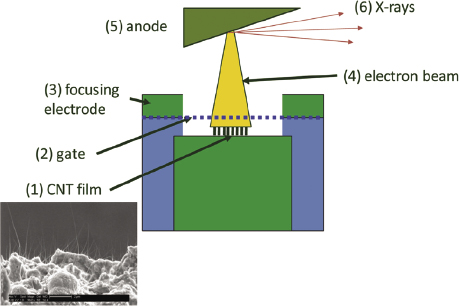
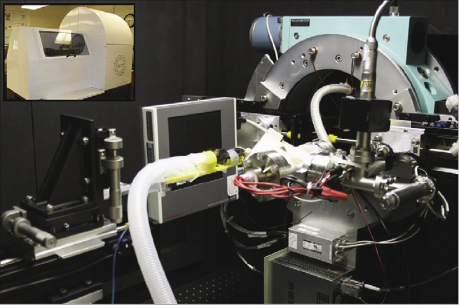
The principal reasons for the continued use of tungsten target tubes today are source brightness, cost, and reliability. Even large patients with transverse body diameters of up to 70 cm and body weight of more than 250 kg can be penetrated readily to produce high signal-to-noise ratio images with conventional X-ray tubes. This is difficult to achieve with any alternative source. The cost of the tungsten target tube, shielding, and power supply is modest in comparison to any alternative technology.
Modern CT scanners with rotating gantries have a medium frequency power supply and liquid or gas cooled X-ray tube mounted on a frame that rotates around the patient. The mechanical characteristics and weight of this system can tolerate 30 Gs of acceleration when rotated 360° around patients in less than 300 ms. The X-ray source can be operated at 80, 100, 120, or 140 kVp up to 1000 mAs, depending on the patient’s body habitus and specific scanning requirements.
Detectors
Almost all CT scanners use scintillators linked to photodiodes as detectors. The solid-state systems can be built with sufficient economy and performance to satisfy the demanding requirements of modern scanners. They have significant limitations, however, particularly dose efficiency and electronic noise floor. In the future, direct photon-counting detectors may be perfected and incorporated into CT instruments (Shikhaliev et al., 2009). Photon counting offers significant advantages in low dose performance with reduced electronic noise and the ability to discriminate X-ray energies that carry specific information on the material composition of the imaged objects (Bornefalk, 2011).
Spectral CT
By detecting X-rays with different energies or spectra, it is possible to expand the capabilities of CT, providing better characterization of imaged materials (Figure 5.3 and Figure 5.4). For example, an X-ray beam inspecting a body part may penetrate air, soft tissue, fat, and bone. After administration of intravenous contrast, iodinated agents that enhance blood vessels must be discriminated. Spectral CT can be done at two, three, or more simultaneous energy levels. Using dual energy techniques, it is possible to discriminate structures with calcium, iodinated contrast in vessels, soft tissue, and fat. Beam-hardening artifacts may be suppressed greatly using equivalent mono-energetic CT scan reconstructions reconstructed using dual energy scans (Johnson et al., 2010).
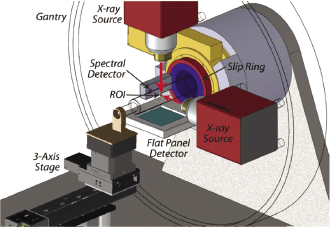
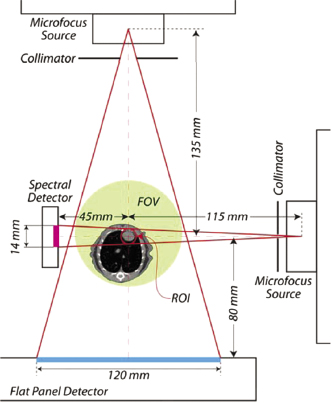
Spectral CT refers to the fact that the X-ray source illuminates body parts with a spectrum of energies that are attenuated differently by structures with varying composition. Spectral CT detectors include the simplest dual energy designs and newer laboratory instruments that can discriminate three, five, or more energy ranges in the detected X-rays. Different materials have variation in X-ray attenuation according to their elemental composition. These can be separated with post-processing image reconstruction to create new CT scan images with a variety of unique and potentially valuable characteristics. For example, the separation of bone and soft tissue can be enhanced. Iodinated contrast within blood vessels can be separated from vascular calcifications due to atherosclerosis in the vessel walls. Artifacts due to metallic appliances can be suppressed. The enhancement of vascular beds by exogenous iodinated contrast can be used to extract CT perfusion parameters such as transit time and blood flow per gram of tissue.
CT Image Reconstruction
Virtually all biomedical CT scanners have utilized the filtered back-projection method to reconstruct images since the early 1980s when the technique was perfected. Despite years of development, alternative CT reconstruction methods did not become popular until recently (Pan et al., 2009).
Filtered back projection is a technique that modifies the raw projections using a finite impulse response digital filter that can be computed rapidly, followed by a back-projection summation step. This algorithm has been implemented in hardware, and conventional medical scanners that produce a 512 × 512 pixel image matrix can reconstruct up to 40 slices per second on state-of-the-art instruments.
The image quality from filtered back projection has been sufficient to satisfy clinical needs. The computational efficiency of the algorithm and modest implementation cost has resulted in universal adoption of the technique (Han/>
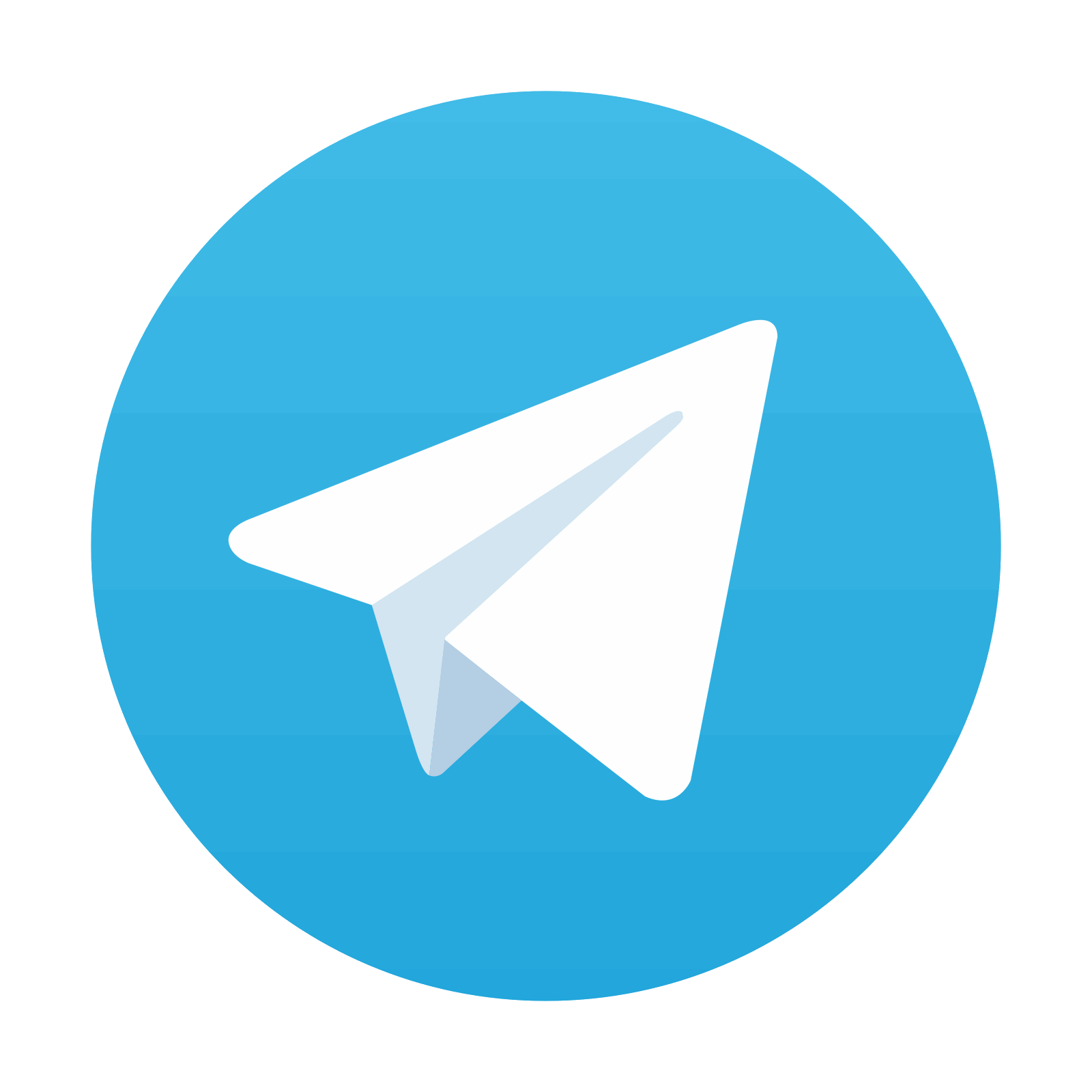
Stay updated, free dental videos. Join our Telegram channel

VIDEdental - Online dental courses
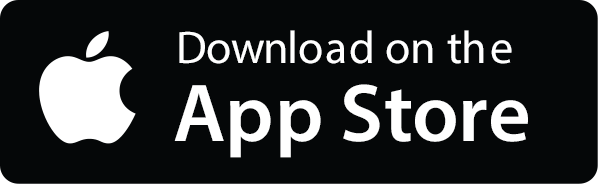
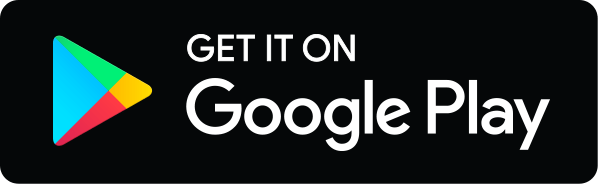