22
3D Assessment of Orthognathic Surgical Outcomes
Introduction
Assessment of treatment outcomes that typically are made relative to the pre-treatment presentation or the planned treatment objectives is performed best through a careful evaluation of post-treatment records that include radiographs. Radiographic imaging allows the orthodontist and surgeon to visualize and quantify clearly the dental, skeletal, and soft tissue changes resulting from treatment. Critical post-treatment analysis forces clinicians to assess objectively whether the treatment provided met the established pre-treatment goals; continual assessment wherein clinicians reproduce the successful and abandon the unsuccessful treatment modalities eventually improves treatment outcomes. It is hoped that by analyzing the final state of a patient’s craniofacial anatomy, clinicians also will be able to assess the factors that may be associated with relapse more fully in order to better predict long-term stability for future patients (Plooij et al., 2009; Kim et al., 2011).
This chapter describes some of the limitations of determining treatment outcomes by two-dimensional (2D) orthognathic surgery imaging. It also highlights how three-dimensional (3D) imaging with CBCT may provide greater insight to the purported dental and skeletal components associated with relapse and post-treatment functional deficiencies.
History and Background of Orthognathic Surgery
Surgery on the jaws initially was performed as early as the 1840s, even before the invention and routine use of radiography (Hullihen, 1849). At that time, there were multiple limitations to successful treatment, including lack of adequate anesthesia, difficulty controlling bleeding, incomplete knowledge of surgical anatomy, lack of understanding of the biological basis for successful surgery, and inability to control infection. While improving the patient’s condition was the stated primary goal, living through the ordeal without experiencing significant complications often was the more practical goal. As an example, Cheever in the first reported LeFort I maxillary osteotomy exposed and removed a nasopharyngeal carcinoma (Moloney & Worthington, 1981). The operation was a success and the carcinoma was removed; however, the patient died about 3 days later.
In the 1930s, Wassmund and others began to revisit surgery on the jaws (Wassmund, 1935). At this time, surgery to correct severe jaw deformities concentrated almost exclusively on the mandible for correction of mandibular prognathism (Blair, 1906; Limberg, 1928; Sowray & Haskell, 1968). Outcome studies were limited and largely relied on isolated case reports or case series using extraoral facial analysis, since lateral cephalometry had not begun to be used routinely yet.
Mandibular surgery was revolutionized by Trauner & Obwegeser (1957) when they reported a novel technique, the sagittal split ramus osteotomy (SSRO), to alter mandibular position. Their technique represented the first intraoral approach to retract the mandible bodily that later was adopted also to advance the mandible. The intraoral approach was made possible with the advent of antibiotics shortly after World War II, which enabled the surgeon to control infections caused by the intraoral flora. Post-surgical relapse and normalization of function remained a shortcoming with the procedure; however, due to wire osteosynthesis, trismus following the 6–8 weeks of intermaxillary fixation, and the unsatisfactory and unpredictable control of the proximal and distal segments, post-surgical relapse and normalization of function remained a shortcoming with the procedure (Isaacson et al., 1978). No new types of osteotomies have been introduced to the field since 1957; rather, various modifications to the original surgical technique have been made (Dal Pont, 1961; Hunsuck, 1968; Epker & Wylie, 1986). Each of these modifications has focused on the goal of enhancing surgical stability, predictability, and return to pre-operative functional levels for which, until recently, the outcomes largely have been assessed by 2D radiography.
Maxillary surgery did not become routine until the late 1970s and early 1980s following the classic microangiography study on monkeys (Bell, 1973), which established the biological rationale for the LeFort I osteotomy. This work, combined with the advent of rigid internal fixation in the 1980s (Reitzik et al., 1981; Barer et al., 1987; Ellis et al., 1988), led to the routine use of orthognathic surgery in coordination with orthodontic treatment in appropriate cases, the subsequent outcome studies for which were performed again mostly by 2D analyses.
2D Mandibular Orthognathic Surgery Studies
Until recently, most studies examining the stability of orthognathic surgery have been performed using analyses from the lateral view. These studies include evaluation of proximal and distal segment rotation (Isaacson et al., 1978), surgical stability (Proffit et al., 1991; Bailey et al., 2008), and the relationship of soft tissue to skeletal tissue changes (Scheideman et al., 1981; Mansour et al., 1983; Stella et al., 1989). For example, Legan & Anderton (1991) compared relapse attributable to changes in the orientations of the proximal and distal segments with two surgical techniques, the SSRO and the intraoral vertical ramus osteotomy (IVRO), following mandibular setback surgery in Class III patients. Since SSRO and IVRO have different osteotomy lines, it is expected that the resultant orientation and approximation of the proximal and distal segments also would be different and could be an influencing factor on skeletal stability. The investigators found that when adverse proximal segment rotation occurs in the rigidly fixated SSRO group, it resulted in a direct negative effect on the position of pogonion resulting in up to 42% relapse. In contrast, in the nonrigid fixation IVRO group, the teeth and pogonion remain stable while the pterygomassateric sling and the hyoid musculature act to “reseat” the proximal segment to a physiologic position. Since physiological proximal segment seating can compensate for unfavorable and unanticipated intra-operative surgical positioning of the proximal segment, some surgeons advocate IVRO as the preferred technique for mandibular setback procedures.
In Class II patients, SSRO is the primary surgical option, since IVRO does not allow for the requisite bony overlap of the proximal and distal segments without an interpositional bone graft. Historically, alternative mandibular surgery techniques such as the “inverted L” and the “C” osteotomies were used for mandibular advancement, but they have been abandoned due to inferior results attributed to muscular influences on the proximal and distal segment orientation. In comparing stability of mandibular advancement surgery with wire osteosynthesis versus rigid internal fixation with SSRO surgery, Dolce and coworkers (2000) found that while the occlusal results remained stable with both fixation methods, the two approaches had different patterns of relapse: rigid fixation patients had more dental relapse, while wire fixation patients underwent greater skeletal changes.
This overview of previous surgical stability studies performed with 2D analyses implicates several variables in surgical relapse, including proximal segment positioning, type of fixation, and muscular influences. However, these studies examined patients only in the lateral plane of space, potentially missing information on other sources of relapse (e.g., condylar remodeling) or extracting additional details on known factors already associated with relapse that might be discernible only with CBCT.
Several studies also have assessed post-surgical changes in soft tissue, many of which have focused on correlating the changes in soft tissue anatomy to its corresponding skeletal landmarks in 2D. In general, these studies show variable soft tissue changes with one-jaw surgery. Thus, soft tissue changes range from 40 to 80% of skeletal change following maxillary advancement surgery. For mandibular advancement surgery, greater consistency is observed with nearly universal 90–100% change in soft tissue to change in corresponding skeletal tissue landmarks. In contrast, changes in soft tissue relative to skeletal tissues are unpredictable in mandibular setback surgery. Overall, these results must be viewed with caution since tremendous inter-patient variability exists: some patients exhibit high magnitudes of change, while others undergo mild changes. While these lateral cephalometric data offer helpful insights on post-surgical changes in soft tissue profiles, this type of analysis is limited by the lack of 3D structural information of the face. Consequently, it is difficult to identify some of the landmarks that are located on both sides of the face and often are observed as double images on 2D analyses. Therefore, 3D information of the shape and true volumetric surgical changes is preferred because this would provide the clinician with accurate data of the whole face and not just that of landmarks located in the midline of the face (e.g., nose tip and chin).
Although cephalometric radiography has been used for post-surgical outcome assessment for many years, this technique has several caveats, including magnification and distortion, superimposition of structures, and the fact that creating a 2D image of a 3D object inherently loses information (Baumrind & Frantz, 1971). In addition, some areas such as the temporomandibular joint (TMJ) cannot be visualized adequately with plane 2D radiography. Even Broadbent’s (1931) attempt to use two orthogonal radiographs to create a 3D view of the subject had limited success. Although these studies were steps in the right direction, extrapolation of two 2D images to reconstruct a “3D” image simply does not generate a true 3D image. While these reconstructions may be adequate for analysis of sagittal and vertical extraoral soft tissue and skeletal changes, they are insufficient to answer a myriad of other questions such as intraoral soft tissue changes, possible surgical effects on airway, and assessment of true condylar position and its potential impact on surgical orthodontics treatment stability.
Evolving Methods for Using 3D Imaging for Surgical Outcomes Assessment
Until recently, surgical skeletal changes in 2D typically were analyzed by using anatomic landmarks on lateral and frontal cephalograms. Anteroposterior (AP) and vertical movements of the maxilla and mandible were analyzed using vectors on a 2D coordinate system; angular changes of cephalometric planes such as palatal plane and mandibular plane were investigated to understand rotations of the maxilla and the mandible. With the availability of medical computed tomography (CT), it became possible to obtain 3D images for pre-surgical diagnosis and treatment planning. However, because of cost and high radiation exposure, the risks of using CT in more routine orthognathic surgery cases outweigh its benefits. Therefore, CTs were used rarely and performed primarily in cases with large asymmetries, severe deformities, or craniofacial defects. The introduction of cone beam CT (CBCT; Mozzo et al., 1998) has provided substantial advances and advantages in overcoming the caveats of costs and high radiation of CTs, while providing images that truly are 3D, are free of structural superimposition errors, and have no magnification. Recent studies (Hilgers et al., 2005) also have shown good accuracy in condylar measurements made from CBCT, but not with 2D images even when the 2D images were reconstructed from a CBCT. Other studies (Berco et al., 2009; Kamburoglu et al., 2011) show good reliability of craniofacial measurements made from 3D cross-sections relative to those from the imaged dry skull. While these findings subsequently have fostered increasing numbers of CBCT studies, an important question of how best to transition the methodologies and approaches used in 2D to 3D remains to be addressed. Of these, the two key issues related to data analysis with CBCT are: (1) how best to superimpose structures in serial CBCT volumes and (2) how to devise methods to derive standardized measurements. These issues have been addressed by various approaches in several contemporary studies performed to evaluate surgical orthodontic treatment outcomes.
Superimpositions of 2D cephalograms traditionally have utilized landmarks in stable areas unaffected by the surgery and growth (Melsen, 1974; Bjork & Skieller, 1983; Nielsen, 1989; Cook et al., 1994). Today, some commercially available software programs allow the operator to perform a similar type of superimposition in 3D using an analogous approach requiring superimposition on three or more landmarks (Figure 22.1). Because this approach is not reliable, however, clinical outcome studies have not utilized this 3D superimposition technique. A second method performs a fully automated, operator-independent registration of scans from two time points using the gray scale intensity voxel by voxel for the cranial base region of nongrowing patients (Cevidanes et al., 2005; Figure 22.2). This technique has a high level of reliability and reproducibility (Cevidanes et al., 2010), and several recently published surgical orthodontic studies have used this superimposition technique to demonstrate treatment outcomes (Cevidanes et al., 2007; Carvalho Fde et al., 2010; Almeida et al., 2011; Motta et al., 2011; see also Chapter 21).
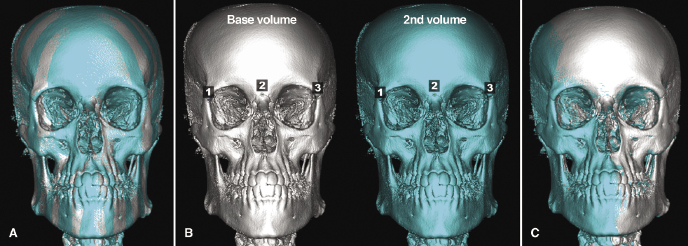
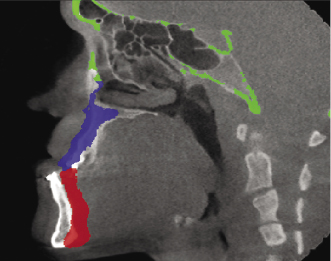
In terms of standardizing and making measurements for 3D analysis, three main methods have been utilized. One is based on linear and angular measurements (Jung et al., 2009; Kim et al., 2010, 2011; Park et al., 2012a) that apply the concepts used routinely in 2D cephalometry to 3D images. The second method is based on the use of iterative closest point algorithms (Cevidanes et al., 2007; Carvalho Fde et al., 2010; Almeida et al., 2011; Motta et al., 2011) that analyze the smallest displacements between two structures but do not take into account the shape being measured. The third and most recent method is called shape correspondence (Figure 22.3). This method correlates the displacement of the same landmark from two or more time points and, through color-coded maps and vectors, depicts the magnitude and directionality of the movement, respectively. Paniagua and colleagues (2010) showed the shape correspondence technique to be a reliable approach for orthognathic surgery simulations.
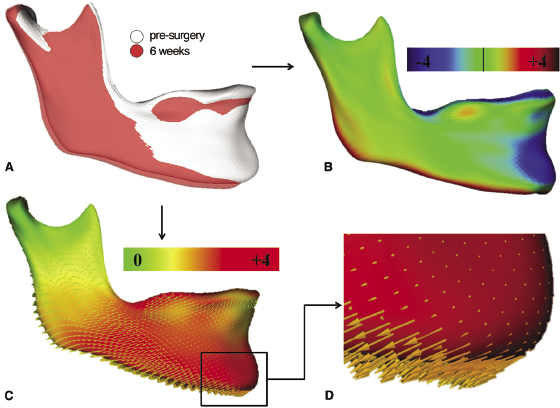
Another key area of interest in assessing post-surgical changes is the pharyngeal airway. The pharyngeal airway is a tubular-shaped space that is filled with air, surrounded by the muscles, mucosa, and a network of lymphatic tissues. Its complex morphology varies greatly depending on the facial skeletal pattern, age, head posture, obesity, and presence of respiratory diseases (e.g., acute tonsillitis). In the past, lateral headfilms were used to screen for pharyngeal airway constrictions that often are caused by adenoid and tonsillar hypertrophy, tongue position, and other abnormalities such as velopharyngeal incompetence. Quantitative analyses for these studies often has included determination of airway widths measured at different levels using reference landmarks such as cervical bones and adenoids and calculations of areas of the adenoids, as well as of different subregions of airways. However, much anatomic information is lost when the 3D airway is represented in a 2D lateral cephalogram, and, as a result, linear and angular measurements alone do not provide adequate information about the structure.
With CBCT, it now is possible to analyze both cross-sectional areas of the airway in all three planes and volumetric measurements of the pharyngeal airway, making it possible to analyze the complex airway anatomy with great accuracy. Recent studies (Ozbek et al., 1998; Aboudara et al., 2009; see also Chapter 13) have confirmed that the cross-sectional area and volumetric measurements have much greater variability than linear and angular measurement of the airway, indicating that these, rather than 2D measurements, likely better represent the normal variations in airway anatomy.
Class III Surgical Evaluations
Despite the vast amount of research concerning surgical evaluation and its long-term effects on post-operative stability and function, most of these studies have been performed using 2D lateral and/or frontal cephalograms with limited evaluation of changes in lengths and areas. CBCT technology has expanded diagnostic capabilities by making it possible to visualize craniofacial anatomy accurately and to perform volumetric analysis, such as that of the changes in relationship of skeletal structures, facial soft tissues, and the pharyngeal airway. This fast-paced and constant evolution of imaging and evaluation techniques can be illustrated using the following examples.
Maxillomandibular Changes
The study demonstrated that the average surgical displacement of the maxilla was similar for both the one- and two-jaw surgery groups, as were the resulting post-surgical changes. However, there was no consistent direction of post-surgical change; some cases demonstrated primarily vertical changes while others exhibited primarily sagittal changes (Figure 22.4).
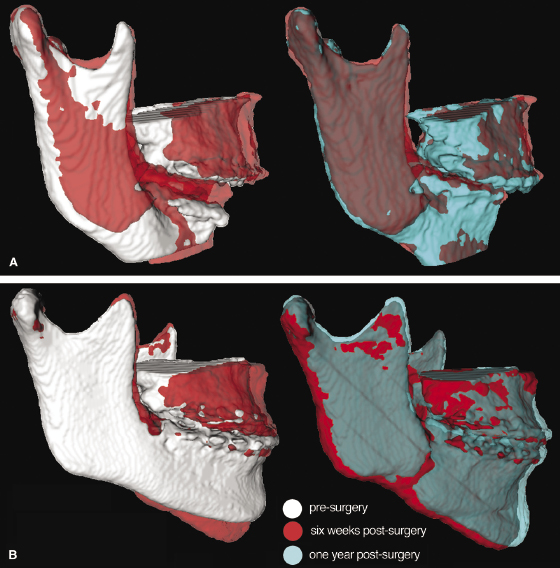
For both groups of patients, the condyles were displaced in a postero-superior direction, though the magnitude of this movement varied between individuals. Additionally, condylar rotation was observed in the two-jaw surgery group, likely resulting from a combination of torquing due to the proximal/distal segment fixation and the rotation due to a wider portion of the mandible being articulated against the ascending ramus due to the setback. Post-surgical condylar adaptations were in the opposite direction of the displacement that occurred immediately following surgery in both groups. Thus, the condyles tended to move antero-inferiorly in both groups and rotational adaptation occurred within the two-jaw surgery group as early as 6 weeks post-surgery (Figure 22.5).
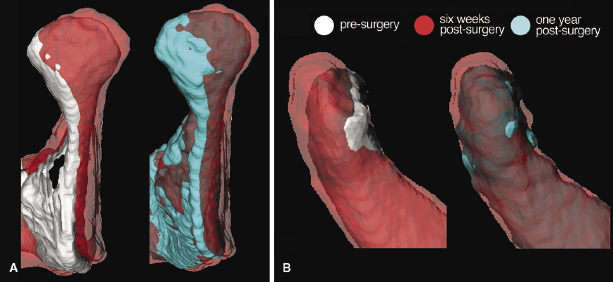
The two study groups also demonstrated differences in surgical changes in the ramus. For the LeFort I-only group, the changes were associated directly with the surgical displacement and post-surgical changes of the maxilla such that the ramus adapted with either clockwise or counterclockwise rotation, depending on the surgical movement of the maxilla. Changes in the ramus in the two-jaw surgery group not only followed the same pattern as that in the maxillary surgery-only group, but also demonstrated lateral rotation of the condyle and a greater anterior ramus displacement when compared with the maxilla-only group (Figure 22.6). This may be the result of the previously reported anterior relapse following surgical mandibular setback reported in studies using 2D analyses, but also could represent a condylar malpositioning that 3D imaging now is able to detect more precisely.
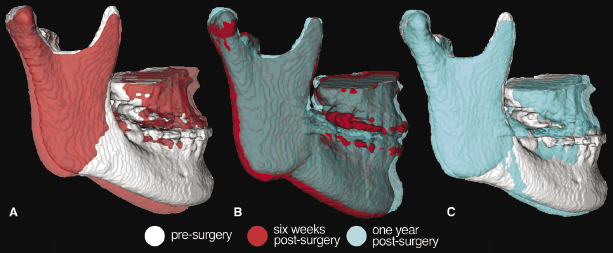
Not surprisingly, displacement of the chin in the LeFort I–only group was due primarily to the amount of vertical displacement of the maxilla. Therefore, the clockwise (maxillary inferior positioning) or counterclockwise (maxillary superior reposition of the mandible) rotation resulted in a corresponding downward and backward or upward and forward displacement of the chin, respectively. In addition to the rotation from an altered maxillary position, the two-jaw surgery group demonstrated a greater posterior displacement of the chin due to the sectioning of the mandible and the posterior positioning of the distal segment. These results led to different post-surgical changes of the chin; the LeFort I-only group exhibited small primarily rotational changes and the two-jaw group demonstrated both posterior displacement and rotation.
Soft Tissue Changes
Because facial appearance is an important factor in human interrelationships and affects social and psychological development, orthognathic surgery must have the objective of correcting skeletal discrepancies as well as altering facial balance to achieve esthetic results in patients with severe disharmony of the jaws (Soncul & Bamber, 2004; Baik & Kim, 2010). The ideal positioning of the jaws through orthognathic surgery does not always result in an ideal soft tissue appearance because the patient’s facial soft tissue does not respond to movement of the underlying skeletal tissues in a 1 : 1 ratio (Lim et al., 2010; Ryckman et al., 2010). Therefore, surgical treatment objectives must focus on the desired soft tissue outcome (Sarver, 1998), rather than on the correction of skeletal discrepancies only.
For the lower third of the face, including chin and lower lip, one- or two-jaw surgeries generally result in similar soft tissue changes, particularly for those with advancement surgery. There is a greater variation in responses within the middle third of the face, however, depending on the type of surgery. The changes in soft tissue anatomy following surgery for Class III correction utilizing landmarks described in Table 22.1 are summarized below.
Table 22.1 Soft tissue landmarks of the face.
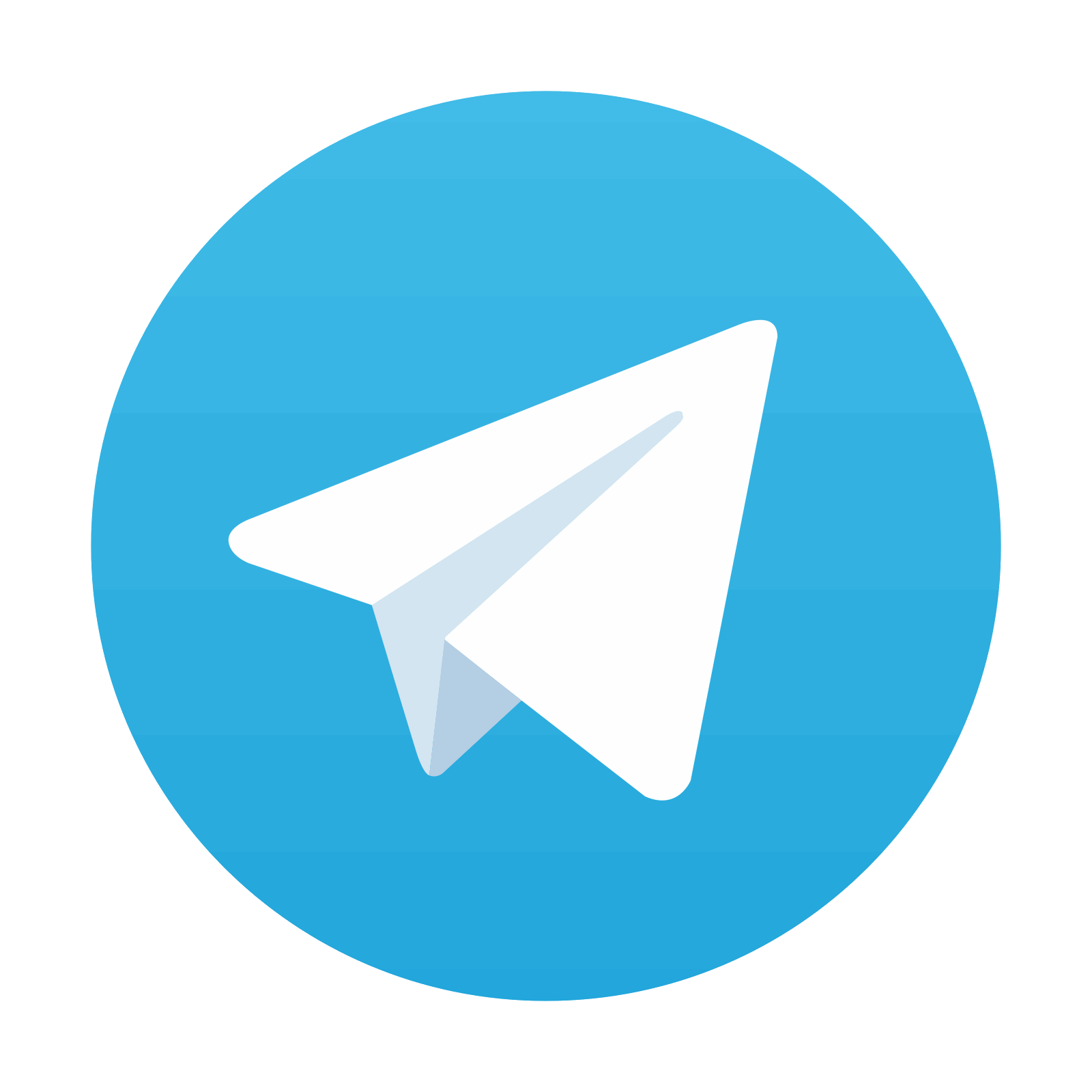
Stay updated, free dental videos. Join our Telegram channel

VIDEdental - Online dental courses
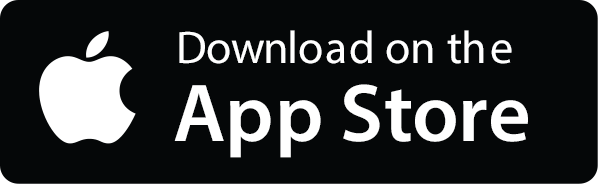
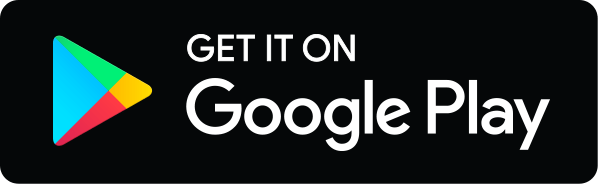