Three‐Dimensional Radiographic Imaging for Implant Positioning
Anders Nattestad
University of the Pacific Arthur A. Dugoni School of Dentistry, San Francisco, California, USA
Introduction
Dental implants are one of the most significant developments in dentistry in the last four decades. The treatment modality has quickly emerged as the most advanced approach to replacing missing teeth. The treatment is, however, relatively costly and can be quite complex from both a surgical and restorative perspective. The surgical challenges frequently evolve around loss of tissue support (bone and soft tissue) for the implants that does not always allow for the implants to be placed in the ideal position for the prosthetic superstructure. Also, as these treatments have become increasingly complex, the need for knowledge sharing and collaboration between the surgeon and the restorative doctor becomes increasingly important. The inherent challenge is often that the ideal position and angle of the implants may not be possible or predictable, which can leave the restorative doctor with the need to compromise the esthetic and/or functional aims. Prior to the availability of three‐dimensional (3‐D) imaging, the restorative doctor would typically manually fabricate a surgical guide based on the ideal position of the implant without always knowing if there was bone to support the implants in that position. During surgery, if it became apparent that the ideal implant position could not be achieved, it was most often not possible to discuss the alternative options with the restorative doctor, and the surgeon would make the unilateral decision how to compromise. With the entry of 3‐D imaging, it is more realistic to analyze the availability of bone in greater detail and to simulate the possible outcomes of different treatment options. Ultimately the technology could allow for greater accuracy of the placement of the implants by using 3‐D guided surgery. This chapter will describe the technology and evaluate the current evidence behind this treatment option.
Three‐dimensional radiographic imaging
The use of 3‐D radiographic imaging was initially confined to large hospitals using either computed tomography (CT) or magnetic resonance imaging (MRI). The latter does not show hard tissue well and is used little for dental applications. CT was at first very expensive, difficult to access, and came with a high radiation dose that limited its elective use in dentistry.1 This changed with the development of cone‐beam computed tomography (CBCT), where the radiation was sent out as a beam rather than a full‐size slice, dramatically reducing the radiation dose. The CBCT technology also offered availability and affordability to 3‐D imaging, and because of these benefits has become an important tool, especially in implant dentistry.2 There have even been discussions about whether or not CBCT should be considered the standard of care in implant dentistry and thus recommended for all patients. However, although it is widely agreed that CBCT is a very useful tool for most patients, there may be a limited number of cases where this technology offers inconsequential additional information and advantage.3
With the addition of 3‐D information in implant dentistry, it is now possible to consider not just evaluating the patient in three dimensions, but also to do virtual treatment planning, and ultimately a completely digital workflow from surgical placement through a 3‐D image‐guided template to an all‐digital impression.2 This technology obviously requires great accuracy to be predictable and this chapter will address this important issue in detail.
The contemporary choice for 3‐D radiographic imaging is de facto the CBCT. No other 3‐D radiographic options should be considered at this time for implant treatment planning.3 The early generations of CBCT were characterized by low resolution and the only way to increase the resolution was to increase the amount of radiation and the time it took to capture the volume. In later generations, the quality of the sensor was improved and that allowed for less radiation and shorter volume capture times.4 The latter also reduced the risks of movement during the scan, improving the quality of the captured volume. In the current generation of CBCT, the quality of the image is adequate for diagnostic needs and the radiation has been minimized to a level that should not limit the use of this technology in dental practices. One important consideration for investing in CBCT technology is medical–legal liability. From a medical–legal standpoint, the practitioner is responsible for identifying pathology – even if it is outside the dental field or outside the experience of the practitioner. The simplest way to reduce that concern is to minimize the field of view to the required area only. This will allow for a reduction in radiation as well. The other option to consider is outsourcing a radiographic interpretation from a board‐certified radiologist. This will add to the cost of using the machine, but will reduce the medical–legal concerns.2,5
The issue of radiation
The first use of conventional CT scanning in implant dentistry came with a significant amount of radiation, deeming it unfeasible for routine use (Table 4.1). With the emergence of CBCT, the radiation became a much more manageable issue; even so, over the last couple of years concerns over radiation from CBCT machines in dental offices have emerged. Most of those concerns stem from a misunderstanding of how the newest‐generation CBCT machines can minimize radiation by reducing the field of view (FOV). This brings a CBCT scan down to about the same as a panoramic x‐ray or five times that of the annual naturally occurring background radiation (Table 4.1, Table 4.2). It would appear from Tables 4.1 and 4.2 that concerns over radiation should be minimal when a CBCT scan is taken with a modern CBCT machine with the minimal amount of field of view, and when there is diagnostic information in the images that enhances the outcome of implant surgery.1,2,5 The trend is clearly that the newest‐generation machines have the smallest FOV, and the quickest scans with a smaller and smaller amount of radiation, leading to either the same or improved image quality as compared to the earlier version.
Table 4.1 Effective radiation dosages (in micro‐sieverts) from different sources.1,2,5
Source: Data from Bouserhal 2002.1
Radiation source | Effective radiation (μsv) |
Conventional CT scan | 500–3000 |
Full‐mouth series periapical x‐rays on film | 150 |
Conebeam CT (see Table 4.2) | 10–200 |
Panoramic x‐ray (digital) | 14 |
Flying at 30 000 feet per hour | 4 |
Background radiation per year | <3 |
Table 4.2 Radiation dosage for different CBCT machines and different field of view (FOV).
Source: Data from Benavides et al. 2012.5
Device and FOV | Effective radiation (μsv) |
ICAT first‐generation 13–22 cm | 69 |
ICAT newest generation at 6 cm | 11–15 |
Kodak 9000 3D | 5–38 |
Accuitomo 4 × 4 cm | 13 |
Levels of adjunct use of CBCT in implant dentistry
During the past decade, there has been a significant development in the level of sophistication with which CBCT technology is being used to aid implant dentistry.5 Initially the technology was used mainly to diagnose and visualize the 3‐D availability of bone using multiple cross‐sectional slices of the potential implant sites (passive or CBCT). Later additional tools became available to allow for simulation or virtual treatment planning, and tools to aid the positioning during surgery (semi‐active or CBCT guided), and most recently the use of CBCT combined with a guidance system to facilitate the correct positioning of surgical instrumentation (active or fully CBCT guided).6 This technology is still in very early stages of development and there has been relatively little development in this technology as compared to the use of guides to facilitate implant accuracy. At this time there is no evidence that this approach can or will become more accurate than guided surgery described in more detail in this chapter. For the remainder of this chapter, the focus will be placed on the first two levels.
Diagnostic use of CBCT (passive or CBCT aided)
The CBCT scan is used primarily to evaluate the anatomy of the implant site in three dimensions. The advantage over conventional radiographs is the added third dimension that can be difficult to evaluate clinically depending on the case. The 3‐D representation will help evaluate the cross‐sectional width of the bone around the implant site and the proximity to important anatomical landmarks, such as nerves and vessels. In addition, it can serve as a more accurate tool for measuring the vertical amount of bone in the maxilla, where the maxillary sinuses often limit the availability of bone. The diagnostic value depends on the assessment of clinical findings and the conventional radiographs, which may indicate an adequate amount of bone in the implant site both vertically and horizontally, and in those cases CBCT will add little extra diagnostic value.
The CBCT volumetric data can also be used to evaluate the bone density. Soft tissue will similarly be assigned different shades of grey (in the darker spectrum). It is, however, very difficult to see the difference between gingiva, tongue, and lips. Therefore, it has been shown useful to scan patients with cotton rolls or gauze to keep these tissues apart. This will increase the diagnostic quality of the image.5,7 This gradient in radiodensity is often expressed in Hounsfield units (HU), which is a linear scale based on the difference between the radiodensity of air and water. This scale is very useful with medical or conventional CT scans, which are calibrated to this scale. There is third‐party software available on the market that will apply this scale to CBCT imaging, which is not as reliable as medical CT, as the CBCT is not calibrated in the same fashion due to the volumetric data acquisition and reconstruction of CBCT. However, recent studies suggest that there may be a strong correlation between high CBCT Hounsfield units and increased bone density and correlation to good bone quality and higher initial insertion torque of implants.5 It has been suggested, for example, that implants placed in bone with HU below 500 should not be loaded immediately.8 Except for this facility of bone density evaluation and Hounsfield units, the diagnostic level of sophistication with CBCT and implants can usually be accomplished without any third‐party imaging software or special equipment. Most CBCT scanners come with software to scan and view the images captured and many will allow for a read‐only viewer to be delivered with the image, if the machine is not on site.
Implant planning (semi‐active or CBCT guided)
The next level of sophistication of the use of CBCT in implant dentistry is to use third‐party software that can simulate the brand, width, and length of the implant, and be used to visualize the implant when placed relative to the bony architecture and in proximity to relevant anatomical landmarks, such as the inferior alveolar nerve and the maxillary sinuses. This simulation aids in the determination of appropriate width and length of implants and may result in a decision to graft bone either concurrent with the implant or months before the implant is placed.6 As such, these 3‐D simulations are also very useful in communications between the involved parties, such as referring dentist, surgeon, and/or laboratory. If the CBCT is captured with a radiographic template (Figure 4.1) it will allow for the position of the implants to be correlated to the desired position of the crown and the relative trajectory of the implants can be discussed between the surgeon and the restorative doctor. The preoperative availability of virtual planning can improve the quality of the discussion of the design requirements of screw‐ vs. cement‐retained restorations. It is also possible to drill the desired trajectory of the implant in the radiographic guide. This “access hole” can be visualized with gutta percha or other fiduciary radiopaque material on the CBCT image and, if this fits well with where the bone is, the radiographic guide can be converted into a surgical guide or manual modifications can be made as necessary. At this basic level of sophistication, the surgical guide is not customized accurately to the implant brand, size, or exact position, but rather to be used in a semi‐guided approach to placing the implant. It should not be expected at this level that there is a great correlation between the planned and actual position of the implant.9
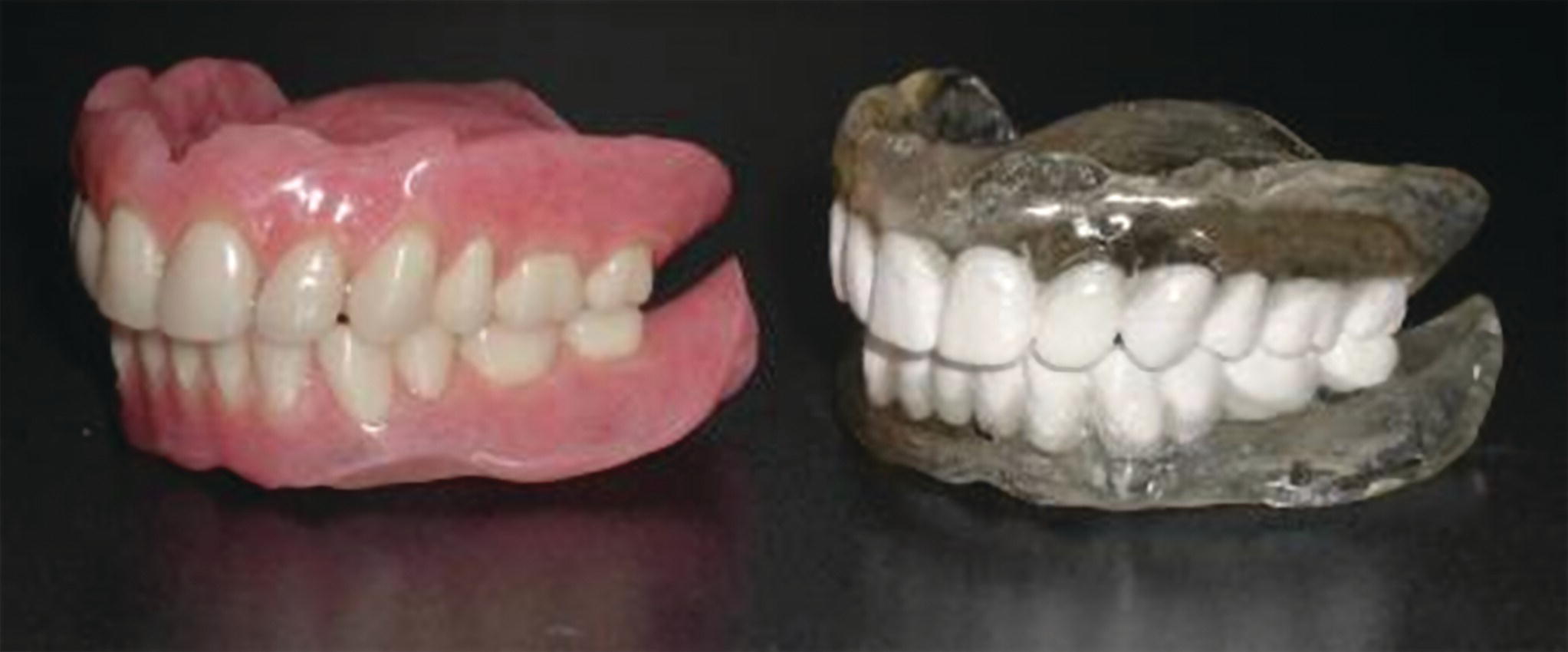
Figure 4.1 Radiographic template used for identifying the desired position of the restored teeth, here marked by barium sulfate.
Source: Reproduced with permission from Charles Goodacre.
The next level of sophistication is to digitally replicate the initial and/or revised planned brand, size, and position of the implants in a surgical guide that is fabricated more directly, based on the virtual planned position of the implant(s) on the CBCT. There are several different ways to achieve this and they have been shown to not all be equally accurate.5,10 The first comprehensive systematic review of these systems was done by Jung et al.6 in 2009 using published reports until the end of 2007. They reported on three different approaches to this transfer of the virtual treatment planning to the surgical placement of the implants: a) mechanical positioning devices or the use of drilling machines based on a transfer of a coordinate system from the patient’s CBCT scan; b) CAD/CAM stereolithographic (SLA) models; and c) navigation systems, where the position of the implant driver was recorded in three dimensions and compared in the planning. The outcomes in terms of accuracy and survival of implants were very mixed among different studies and techniques.6 There has been a significant amount of research done on types a) and b). These are described below, differentiated by manufacturing process and how the guide is fixed to the patient. As for type c), the navigation system, it is still in a very early stage of development and it would appear that additional data and refinement of the technology are necessary before that technology is ready for a more comprehensive deployment.
Generations of guides for CBCT‐guided surgery
During the last 10 years, CBCT image‐guided implant surgery has gone through several generations of the imaging and fabrication process. During this iteration process, reports of varying outcomes have been plagued with heterogeneity, leading to difficulty in assessing the critical variables for accuracy. The first variable was the quality of the CBCT, including the slice thickness. Some early studies used very large slice thickness and later studies had much finer details, with slice thickness down to about 0.2 mm. A second variable was that guides can be placed on bone, soft tissue, or teeth. A third variable was the fabrication method for the guide. Some studies used printed stereolithographic (SLA) guides and other used a vacuum‐formed splint on a printed SLA model. Finally, a fourth variable was the method and amount of guidance. Some of the early generations had a metal sleeve for the pilot drill only, whereas others used a series of handles to center the osteotomy of more/all of the drill diameters. Some guides and drill systems allow for placement of the implant through the guide and some have a vertical stop on the delivery process to indicate when the implant is at the planned vertical position. Due to the complexity of combining simultaneous variations in these four parameters, guided surgery experiences have varied and studies have revealed significant variation in outcomes. In the following paragraphs, it has been attempted to describe the most common combinations of these variables and correlate them to outcomes as evaluated by accuracy and implant survival. It is critically important to evaluate these variations to understand the opportunities and limitations with this technology.
Bone‐level and SLA printed guides
The first guides that were described were SLA printed to be placed on bone. An early comprehensive report on this approach was by van Steenberghe et al.,11 which evaluated edentulous patients receiving multiple implants with an immediate loading protocol. This study was predominantly focusing on the protocol and patient satisfaction with the Nobel Biocare “Teeth‐in‐an‐hour” concept, which has since been discontinued in its original form. Another of the early reports on guided surgery was by Lal et al.12,13 in 2006, where the use of SLA templates to plan and place implants was described. This was mostly a proof‐of‐concept study with a case report. The approach used was a bone level guide made on a SLA printed model of the jaw based on a CT scan with 1 mm slice thickness. The printed SLA model, slice thickness, and use of bone to position the guide have later all proven to be the least accurate approach to guided implant surgery. Later in 2008, Nikzad and Azari14 published on a methodology very similar to that of Lal et al.12
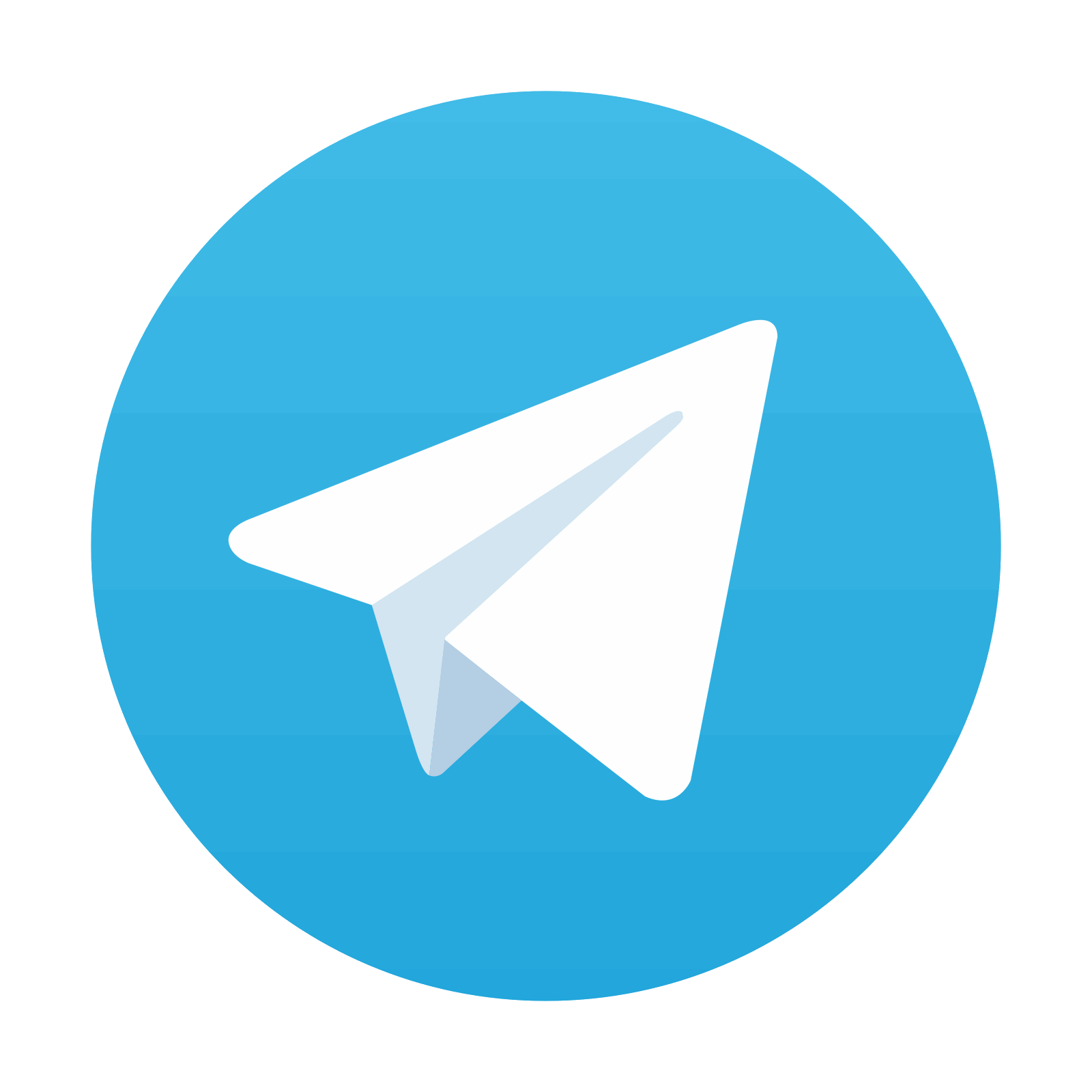
Stay updated, free dental videos. Join our Telegram channel

VIDEdental - Online dental courses
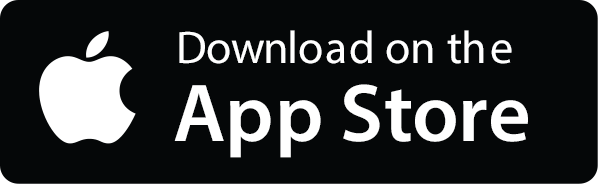
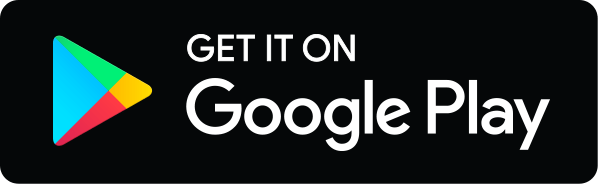