Chapter 37 Tooth Extraction, Socket Grafting, and Barrier Membrane Bone Regeneration
Two fundamental concepts determine the position of an individual implant within a treatment plan: biomechanics and esthetics. Both of these concepts require adequate bone volume to accomplish their goals. Biomechanics requires key implant positions as the canine and first molar sites. When available bone is not present in those locations, bone augmentation is required. When the goal is an FP-1 restoration with ideal crown contour and soft tissue drape, abundant bone is necessary. If the available bone is inadequate, bone augmentation is indicated. Both factors of biomechanics and esthetics often require ideal available bone volumes.
ATRAUMATIC TOOTH EXTRACTION
The process of atraumatic tooth extraction and maintenance (or augmenting) of soft and hard tissues begins with the soft tissue. The cells of the inner layer of the periosteum are responsible for bone remodeling. Whenever the periosteum is reflected, the cells are injured and need to regenerate before the remodeling process begins. The cortical bone receives more than 80% of its arterial blood supply and faciliates 100% of its venous blood return through the periosteum.1 When the bone volume is ideal, the periosteum should not be reflected. However, the periosteum also limits the volume of bone formation. When the periosteum is separated from the bone graft by a barrier membrane, more volume of bone is regenerated. The periosteum helps bone remodeling or bone repair, but it limits bone modeling and regeneration.
The extraction of a natural tooth begins with an incision within the sulcus, preferably with a thin scalpel blade rather than a blunt periotome, 360 degrees around the tooth, to cut the connective tissue attachment fibers above the bone (Figure 37-1). There are 13 different connective tissue fiber groups around a tooth, of which six directly insert into the cementum of the tooth above the bone. If these fibers are not cut before the extraction, trauma to the soft tissue is imminent; soft tissue tearing may occur as well. The tip of the interdental papilla is avascular and should not be incised when possible. The soft tissue should not be reflected, as soft tissue retraction and shrinkage during initial healing is more evident, especially in the interdental papilla region.
The next step in an atraumatic extraction process is to observe the crown and root anatomy, especially in multirooted teeth. When a tooth is extracted next to adjacent teeth, the pathway of removal is often obstructed by the position of the adjacent tooth. If the tooth to be extracted is not reduced (especially mesial and distal), instruments may chip the enamel (or restoration) of the adjacent tooth and may cause the extraction of the tooth to take an altered pathway of removal, which is more likely to fracture the roots, bone, or both (Figure 37-2). If the roots of the tooth to be extracted are divergent, they should be sectioned and removed as individual units, rather than risking fracture of the roots or surrounding bone (Figure 37-3). When the roots are fractured, there is an increased risk of bone fracture/removal to retrieve them. If bone removal around the tooth is necessary (because the tooth is fractured or decayed to the bony crest), it ideally should be at the expense of the lingual alveolus, not the more labial bone.
Biomechanic concepts have been used to extract teeth for thousands of years and date back to the days of Aristotle (384-322 bc),2 who described the mechanics of the extraction forceps, including the advantages of “two levers acting in contrary sense having a single fulcrum.” This was 100 years before Archimedes reported on the principles of the lever.
Pierre Fauchard (1690-1761) is credited with being the pioneer of scientific dentistry and gave specific instructions for extracting teeth using a dental elevator, a “pelican,” or pincers (forceps).2,3 He describes loosening the tooth with an elevator, then using the claw of the “pelican” (invented by Chauliac in the fourteenth century).4 The pelican handle was positioned both on the tooth and on the gum below the tooth while it was rocked back and forth (which he called “shaking”) before the extraction. Taft described a similar technique using the dental key, which had left and right claws that provided twisting and rocking in both directions.5 This allowed the tooth to be loosened sufficiently to be pulled from the socket with “pincers” (forceps). Modern extraction forceps date from Tomes in 1840 with the development of the anatomical forceps, complete with a handle and beak to fit the neck of the tooth.
The principle of the dental elevator is also not a modern development. Abulkasim (ad1050-1122) was the first to apply a single lever (elevator) under the tooth to force it from its “bed.” It was improved by Ambroise Paré in the sixteenth century to lift out the tooth before using the pelican.5 Although these biomechanical methods to remove teeth are effective, a review of the biomechanical principals is in order to decrease the trauma during the tooth extraction process.
Periotomes are usually longer and thinner wedges compared with dental elevators and should be used to begin the atraumatic extraction process. Periotomes may be used in a similar manner for extraction of intact teeth or removal of retained root fragments. The long axis of the periotome blade should be inserted into the interproximal region along the root long axis (to protect the facial plate of bone), with the tip of the periotome blade located within the crest of the alveolar bone. The instrument is then pushed into the periodontal ligament space or tapped with a mallet into the periodontal ligament space along the mesial and distal root, severing the periodontal ligament immediately below the alveolar crest and wedging the tooth against the opposing cribriform plate (Figure 37-4, A).
A period of 10 to 30 seconds is allowed to elapse while the instrument is in place. This allows biomechanical creep to occur to the ligament and reduces its strength, and because the tooth is pushed against the opposing alveolus, it also begins to expand the bone. This process is much more effective when there is no adjacent tooth contact. Reducing the mesial and distal contacts of the tooth to be extracted not only decreases the risk of damage to the adjacent tooth crown, but also aids in the first step to extract the hopeless tooth. The periotome is then pushed farther down into the periodontal ligament toward the root apex, often using a mallet and light tapping force. This process continues along the crestal one third of the tooth. At the completion of this procedure, the tooth is often slightly mobile (see Figure 37-4, B).
Once the periotome is used as a moving wedge, it may then be converted to a lever (see Figure 37-4, C). The blade of the periotome is often 3 to 4 mm wide. When the handle is rotated, one side of the periotome is applied to the tooth root, the other side to the cribriform plate, and the width of the “wedge” is now the length of a lever, which magnifies the rotation force (moment). The rotation of the periotome handle increases both tooth mobility and the force against the opposite cortical plate to further expand it within physiologic limits.
A single-rooted tooth is most often tapered. As the periotome is tapped down to the cribriform plate and slightly rotated, the tooth often is pushed against the opposing cribriform plate. Because the socket is tapered, the lateral force on one side of the tooth is converted to a coronal direction force on the other side and the root is pushed out of the socket. As a result, the periotome may now be pushed farther apical,1 toward the root apex. When time elapses between each force application, the tooth may even slide up and completely out of the socket. Additional time and elevation may be required if significant tooth mobility is not achieved.
A traditional dental forceps should not be applied to the tooth until significant tooth mobility is achieved. Once the wedge and lever action of the dental elevator is applied to a tooth, most often a dental forceps is used to ultimately grasp and deliberately rock the tooth back and forth and to rotate it as much as conditions will allow. The combination of these tooth movements expands the bony socket and separates the periodontal ligaments. As a consequence, the tooth may be removed (see Figure 37-4, D).
where F is the applied force and r is the distance from the force applied to the object. The concept of a moment arm is key to the operation of the lever, which is capable of generating mechanical advantage. This means that the force applied to an object is affected by the length of the lever arms. The lever arm is the distance from the force input to the fulcrum or from the fulcrum to the force output.
Once the treatment is in position around the tooth root, no squeezing pressure is applied to the tooth. Instead, the handles, once in position, are rotated as one unit facially for a few degrees and stopped for approximately 60 seconds (Figure 37-5, A). The torque force generated on the tooth, periodontal ligament, and bone is related to the length of the handle to the bumper (8 cm), divided by the distance from the bumper to the forceps beak (1 cm). As a result, a force on the handle connected to the bumper will increase the force on the tooth periodontal ligament and bone by eight times. No force is required to be placed on the forceps beak, which is on the tooth. Therefore the tooth does not split, crush, or fracture. The 60 seconds of constant force cause biomechanical creep into the bone and periodontal ligament. Once creep has expanded and weakened the periodontal ligament and bone, the forceps handle may be slowly rotated another few degrees. This usually releases and elevates the tooth a few millimeters from the socket within an additional 10 seconds (see Figure 37-5, B). At this point the tooth is loose and ready to be removed from the socket using any pincer-like device (e.g., pick-ups, an extraction forceps, a hemostat) (see Figure 37-5, C).
The extraction of a tooth using the Physics Forceps is similar to the removal of a nail from wood with use of a carpenter’s hammer (instead of pliers). The handle of the hammer is a lever, and the beaks of the hammer fit under the head of a nail (they do not squeeze the head). The hammer head acts as a fulcrum. A rotational force applied to the hammer handle magnifies the force by the length of the handle, and the nail is elevated from the wood.
Creep is a phenomenon whereby a material continues to change shape over time under a constant load. In tooth extraction, creep may occur to bone and the periodontal ligament. Reilly established the creep curve of bone, whereby under a constant load of 60 Mpa, the bone over time responds in three different stages.6 The majority of bone changes occur within the first minute, whereby the initial strain of bone (the change of length divided by the original length) is modified. The greater the force, the greater the deformation of the bone. This process allows the tooth socket to expand and the tooth to exit the socket.
HEALING OF EXTRACTION SOCKETS
Once the tooth is extracted, the site may heal by repair or regeneration of the bone. All fibrous tissue from periodontal disease or endodontic origin should be completely removed, as these tissues impair bone formation and delay bone healing for extended periods. Repair occurs when there is injury or conditions of the bone that cause incomplete bone volume to form in the residual ridge. The most common conditions that cause repair are the absence of a labial plate before or as a consequence of tooth extraction. Other factors include a bony wall that is less than 1.5 mm thick (usually the facial), exudate, gross apical pathology, or excessive heat from a dental drill during root extraction. On rare occasions, bisphosphonate drugs or systemic conditions may cause a repair rather than a regeneration process.
Ohta has proposed four stages of bone regeneration after a tooth is extracted with a healthy surrounding alveolus.7 The time frames presented here have been modified from his dog study to reflect the human sigma of bone. The week following extraction is called the initial angiogenic stage. Initial angiogenesis develops from the broken ends of blood vessels in the residual periodontal ligament covering the cribriform plate. Blood plasma leaks from these broken vessels, and immature fibroblasts aggregate at the plasma-rich regions. The blood clot begins to shrink, and capillaries form sinusoids and granulation tissue, starting from the apex and the surrounding walls of bone. Fibroplasia begins early in the sequence during the first week as a result of the ingrowth of capillaries and fibroblasts. White blood cells kill bacteria and begin to dissolve foreign bodies and bone fragments. With few exceptions, the angiogenesis begins at the bottom of the socket because this area is not severely injured during the extraction and has the greatest source of blood vessels. Within 5 days of the extraction, early granulation tissue composed of immature capillaries and fibroblasts appears at the bottom of the socket and spreads upward along the socket walls. New bone trabeculae also form at the apical portion of the socket during this early stage.
The new bone formation stage begins as early as the third week after extraction.7 At this time, the entire socket is filled with granulation tissue. This period demonstrates the greatest sinusoid formation activity. The forming trabeculae of woven bone first start from the bottom of the socket following the meshwork of newly formed anastomosing sinusoidal capillaries. Bone formation is more rapid at this point, creating a three-dimensional lattice pattern of woven bone. It has been observed that, during this time, the cortical bone of the marginal crest of the socket continues to resorb, especially in the interseptal regions and the thinner facial plate.
The bone growth stage starts 4 to 5 weeks after the extraction.7 New bone trabeculae forming on the walls and apical region have thickened and fill the apical two thirds of the socket. The center of the socket is still woven bone because ossification occurs on randomly formed collagen fibers; hence its lattice appearance. The more organized lamellar bone starts to form from the lining of the socket toward the center of the socket.
The bone reorganization stage occurs at least 6 weeks after extraction.7 The primary bone trabeculae remodel to form thicker secondary spongiosa. This process always begins at the apex of the extraction socket. The complete cortical lining of bone around the socket is not fully resorbed, and remodeling continues for 4 to 6 months after the initial extraction (Figure 37-6).
SOCKET GRAFTING
Multiple bone graft procedures and studies have been evaluated for socket augmentation at the time of extraction. However, variable results have been observed, not only among different reports, but also within each study.8–11 Rather than use the same technique regardless of clinical conditions, when bone repair rather than regeneration is likely, the clinician should provide as many keys to bone grafting as possible to increase the socket.
In 1993, Misch and Dietsh suggested different graft materials and techniques based on the number of bony walls that remained after the tooth is removed.12 A thick five bony wall defect will grow bone with almost any resorbable graft material (RGM); for example an alloplast, allograft, or autograft. When a wall of bone is less than 1.5 mm or a labial plate is missing (four bony wall defect), an autograft or an alloplast or freeze-dried bone (FDB) with BM and GBR increased the predictability of restoring the original bony contour. Becker et al. evaluated demineralized freeze-dried bone (DFDB) alone in extraction sockets, and no evidence of bone formation was observed.13 It appears DFDB alone may be a poor choice for socket grafting. LeKovic et al. compared extractions alone to BM with extractions. At 6 months, crestal bone loss (0.38 versus 1.50 mm) and horizontal ridge resorption (–1.31 versus −4.56 mm) were found.14 A two or three bony wall defect requires an RGM and, at least, some autogenous bone with a barrier membrane. A block graft of cortical autogenous bone fixated into the host bone position is suggested for one bony wall defect (Figure 37-7).
Thick Five Bony Wall Defect
Regeneration restores complete morphology and bone volume to the residual ridge. This most often occurs when there are five thick bony walls around the extraction site. Most of the keys for predictable bone formation are present under these conditions, and the socket often forms bone in the extraction socket without loss of width or height. The atraumatic extraction of a tooth without pathology provides many of the keys necessary for predictable bone regeneration. The extraction process sets up a regional acceleratory phenomenon (RAP) for healing (which increases the rate of repair and adds bone morphogenetic protein [BMP] to the site); the five bony walls protect the graft from mobility; the torn blood vessels in the periodontal complex leak growth factors into the region (platelet-derived growth factor [PDGF], transforming growth factor [TGF]); the space is maintained by the five walls of the bone; the bony walls provide blood vessels from bone into the site; and the defect size is small (i.e., one tooth). As a result, the only key initially missing is soft tissue closure (Figure 37-8). The soft tissue around the extraction site begins to grow over the clot and granulation tissue of the socket and within 2 to 3 weeks covers the site. For example, a mandibular third molar extraction site often has thick lateral walls of bone, and most often the bone area is regenerated in both height and width with no graft material or regeneration technique in the sockets.
Four to Five Wall Bony Socket
When conditions of repair instead of regeneration are present, socket grafting for ridge augmentation at the time of extraction is indicated.12 Tooth extraction without grafting in these conditions results in residual bone loss as a result of resorption. The maxillary anterior region may be reduced 23% in the first 6 months after an extraction and another 11% over the following 5 years.15 Within 2 years, an average of 40% to 60% of the original height and width of bone may be lost with multiple extractions.16
Barrier Membrane with Alloplast/Freeze-Dried Bone
When the facial plate is thin, the socket may be filled with FDB (e.g., MinerOss, Puros) or a mineralized hydroxyapatite (HA) source (e.g., BioOss, Osteograf-N). When the labial plate is missing, the FDB may be placed in the apical portion, but particulate autologous bone should be placed in the crestal half of the socket. The walls of bone on the mesial, distal, and palatal provide bone blood vessels to this autograft. The extension of collagen or AlloDerm then covers the top of the socket and is tucked below the palatal tissue. Sutures are then placed over the top of the BM. Primary closure of the soft tissues is not obtained, as the tissues would need to be reflected and advanced over the socket, which would affect the soft tissue drape (Figure 37-9, A to H).
The extraction site may be reentered after 4 to 6 months. The clinical time for reentry is determined by the absence of the cortical lining of the socket (cribriform plate) on a periapical radiograph. Once this has occurred, the implant may be inserted and a regular protocol of healing and restoration used (see Figure 37-9, I to N).
Maxillary Tuberosity Donor Site
The tuberosity offers a variable amount of trabecular bone. This area is convenient for use in maxillary sinus grafting12,17,18 and also may be considered for smaller areas of ridge augmentation19,20 (Figure 37-10). The cancellous nature of the bone allows it to be molded into an alveolar defect, such as an extraction socket.12 However, the trabecular graft will more often require the use of a barrier membrane in onlay grafting to minimize resorption and stabilize the graft.21 The tuberosity autograft has growth factors for osteoinduction and to accelerate blood vessel growth in the host site.
Socket Seal Surgery
A composite graft socket seal surgery has been developed by Misch et al.22,23 composed of connective tissue, periosteum, and trabecular bone used to seal a fresh extraction socket. A connective tissue graft has the advantage over a keratinized graft by blending into the surrounding attached gingival regions, offering similar color and texture of the epithelium. This is most advantageous in the maxillary anterior region and other aesthetic areas. The composite graft also contains autogenous bone. The major advantage of autologous bone is a more rapid and predictable bone formation via osteogenesis. This technique may be used any time a tooth is extracted and an implant is planned as replacement.
A 6- to 10-mm trephine bur corresponding to the extraction site diameter is used in a slow-speed, high-torque hand piece to harvest a gingival graft with underlying bone. The most common site for the intraoral composite graft harvest is the maxillary tuberosity region (see Figure 37-10). The trephine drill drills through the unreflected, keratinized, attached gingiva and into the bone of the tuberosity region at the prescribed depth related to the thickness of the tissue and the amount of donor bone available. Care should be exerted not to enter the antrum, but that is of little consequence if the antrum is healthy during the process. A trephine bur may be used as a lever to greenstick fracture the bone core from its base, once it is in position within the bone. A Molt elevator may also be used for this purpose. The bone core (usually 5 to 10 mm in height) and the attached soft tissue (about 3 mm in height) is trimmed of its epithelium with a tissue scissors, leaving 3 to 6 mm of connective tissue attached to the bone core.
If the bone core does not fill the extraction socket completely, a mineralized bone graft material (e.g., FDB, bovine bone) may be used in the apical portion of the socket, provided the labial plate is still intact. Because the new bone forms from the apical portion of the socket, this is the least important region to augment. If no bone plate remains in the apical half of the socket, additional autogenous bone should be harvested from an additional intraoral site to overfill the apical half of the socket. The bone of the composite graft (connective tissue attached to periosteum and bone) is compressed and fitted into the remaining portion of the socket. The tissue of the composite graft will seal the socket and remain above the surrounding gingiva. A mallet and blunt instrument should be used to tap it into place and compress the bony core to conform to the crestal contour of the socket. The connective tissue portion of the graft is then sutured to the surrounding gingival tissue with facial and palatal interrupted 4-0 PMA or Vicryl sutures (Figure 37-11). A removable transitional prosthesis should not be permitted to load the tissue during the first few weeks after extraction; otherwise, the composite graft may become mobile and sequestrate. A fixed transitional restoration may use an ovoid pontic design to maintain the interdental papilla height and contour.
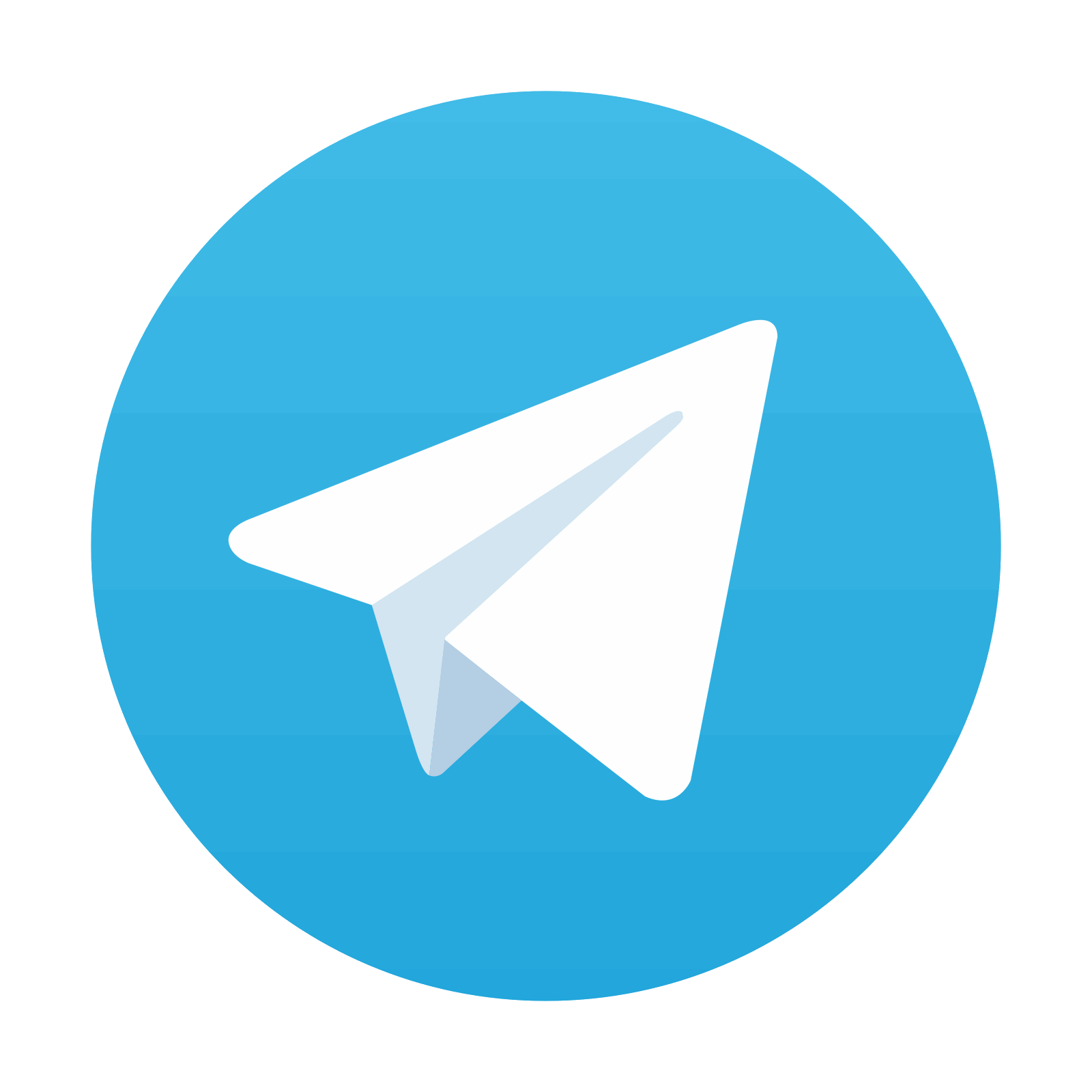
Stay updated, free dental videos. Join our Telegram channel

VIDEdental - Online dental courses
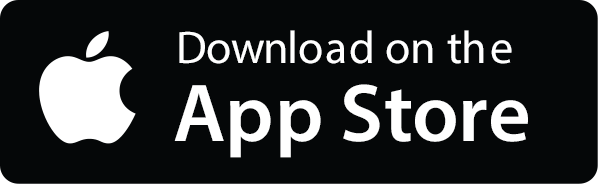
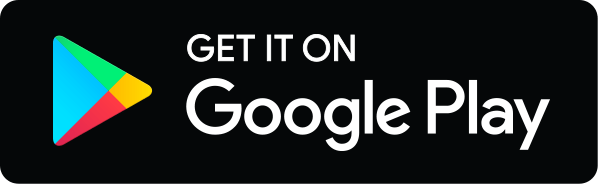