Occlusal Considerations for Implant-Supported Prostheses
Implant-Protective Occlusion
Carl E. Misch
The clinical success and longevity of endosteal dental implants as load-bearing abutments are controlled largely by the mechanical setting in which they function. The treatment plan is responsible for the design of the prosthesis along with the position and number of the implants. The most common complications of implant prostheses relate to biomechanical factors, such as porcelain fracture, unretained prostheses (cement or screw), abutment screw loosening, early implant failure after loading, and implant component fracture1–4 (Figure 31-1). In addition, after achievement of rigid fixation with proper crestal bone contour and gingival health, the mechanical stress or strain beyond the physical limits of hard tissues is a primary cause of bone loss around loaded implants.5–13 This bone loss may affect the quality of health of the implant when the probing depths are greater than 5 mm. Anaerobic bacteria are more likely to be present in these greater pocket depths and contribute to biological complications. In addition, an increased pocket depth is more prone to shrink and may result in a longer-looking crown or loss of interproximal papillae or may even expose the implant crest module (Figure 31-2).
After successful surgical and prosthetic rehabilitation with a passive prosthesis, noxious stresses and loads applied to the implant and surrounding tissues result primarily from occlusal contacts. Complications (prosthetic or bony support) reported in follow-up studies underline occlusion as a determining factor for success or failure.13,14 Yet the choice of an occlusal scheme for implant-supported prostheses is broad and often controversial. The occlusal scheme is especially important during parafunctional activity of the jaws because the magnitude and duration of the parafunctional occlusal stresses are greater than functional stress. It is also more important when the implant foundation is not ideal in number or location to the implant–bone interface because the area of load is reduced.
Almost all implant occlusal concepts are based on those developed with natural teeth and are transposed to implant support systems with almost no modification. This approach has some justification. Complete denture wearers are reported to exhibit mandibular movement and velocity different from patients with natural dentitions. However, Jemt et al. found that after fixed implant reconstructions are placed into previous edentulous patients, the displacement of the jaw during mandibular opening and function is similar in velocity and movement to that in patients with natural teeth.15 Gartner et al. also demonstrated similar habitual chewing for implant patients and patients with natural teeth.16 During maximal occluding forces, electromyograms demonstrated that the implant patient group activated similar working and nonworking muscles as patients with natural dentition. Therefore, it appears logical to derive implant occlusion from occlusal principles for the natural dentition. However, several conditions indicate that implant prostheses are at greater biomechanical risk than natural teeth. As a result, some of the occlusal concepts for implants should be modified from concepts for the natural dentition.
Natural Tooth versus Implant Support Systems
Compared with an implant, the support system of a natural tooth is better designed to reduce the forces distributed to the tooth or restoration and the crestal bone region. The periodontal membrane, biomechanical design, elastic modulus of material, the nerve and blood vessel complex of the tooth, occlusal material, and surrounding type of bone blend to decrease the risk of occlusal overload to the tooth system. The implant system (prosthesis, cement or screw retention, abutment screw, marginal bone, implant–bone interface, and implant components) has higher stresses in comparison17 (Box 31-1).
The presence of a periodontal membrane around natural teeth significantly reduces the amount of stress transmitted to the bone, especially at the crestal region.18 The displacement of the periodontal membrane dissipates the energy to the fibrous tissue interface (periodontal ligament) surrounding natural teeth and acts as a viscoelastic shock absorber, serving to decrease the magnitude of stress to the bone at the crest and to extend the time during which the load is dissipated (thereby decreasing the impulse of the force).19 The force transmission is so efficient and within ideal strain conditions for bone that a thin layer of cortical-like bone (cribriform plate) forms around the tooth. When the tooth is lost, the cortical plate lining disappears, demonstrating that this is not an anatomic structure but is a result of an ideal strain interface to the bone. Compared with a tooth, the direct bone interface with an implant is not as resilient. No cortical lining is present around the implant because the energy imparted by an occlusal force is not dissipated away from the crestal region but rather transmits a higher intensity force to this marginal contiguous bone interface (Figure 31-3).
An implant receives a greater impact force than a natural tooth because it is not surrounded by a periodontal complex. The fact that the implant is more rigid actually means that the implant system receives greater force and is more at biomechanical risk than a natural tooth. Remember that the implant system includes the occlusal porcelain on the crown (which may fracture), the prosthesis may fracture, the cement or screw that retains the prosthesis may debond or loosen, the abutment screw that contains the components may loosen, the crestal marginal bone may be lost from pathologic overload, the complete implant–bone interface may result in mobility and failure, intermediate to late periimplantitis may result in poor esthetic outcomes, and the implant components may result in fracture (Box 31-2).
An analogy of the difference in impact force between an implant and a tooth is hitting a nail with a steel hammer (a rigid structure) compared with a rubber hammer (a mobile structure). The more rigid hammer transmits a higher intensity force and drives the nail farther into the wood rather than having the energy partially dissipated through deflection of the rubber hammer.
The mobility of a natural tooth can increase with occlusal trauma. This movement dissipates stresses and strains otherwise imposed on the adjacent bone interface or the prosthetic components. After the occlusal trauma is eliminated, the tooth can return to its original condition with respect to the magnitude of movement. Mobility of an implant also can develop under occlusal trauma. However, after the offending element is eliminated, an implant does not return to its original rigid condition. Instead, its health is compromised, and failure of the entire implant system is usually imminent.
A lateral force on a natural tooth is dissipated rapidly away from the crest of bone toward the apex of the tooth. A healthy, natural tooth moves almost immediately 56 to 108 microns (primary tooth movement) and pivots two thirds down toward the tapered apex with a lateral load19–20 (Figure 31-4). This action minimizes crestal loads to the bone. An implant does not exhibit a primary immediate movement with a lateral load. Instead (with a greater force), a more delayed secondary movement of 10 to 50 microns occurs, which is related to the viscoelastic bone movement.21 In addition, this movement does not pivot (as a tooth) in the apical third of an implant but instead concentrates greater forces at the crest of surrounding bone. Therefore, if an initial lateral or angled load (e.g., premature contact) of equal magnitude and direction is placed on an implant crown and a natural tooth, the implant system (crown, cement or screw retention, abutment screw, marginal bone, implant–bone interface) sustains a higher proportion of the load.
The width of almost every natural tooth is greater than the width of the implant used to replace the tooth (Figure 31-5). The greater the width of a transosteal structure (tooth or implant), the lesser the magnitude of stress transmitted to the surrounding bone.17 Molars have greater dimensions than premolars (greatest bite forces in molar region), and the maxillary molars have greater root surface area than the mandibular counterparts to compensate for the difference in surrounding bone density and form. The size of the implant is often a polarized opposite of the natural teeth. The size of the implant often is decided by the existing bone volume rather than the amount and direction of force. Hence, the greatest-size (surface area) implants are often inserted into the anterior mandible followed by the anterior maxilla, the posterior mandible and the smallest-size implants are often inserted into the posterior maxilla.
The cross-sectional shape of the natural tooth at the crest is biomechanically optimized to resist lateral (buccolingual) loads because of the bending fracture resistance (moment of inertia) of the tooth and the direction of occlusal forces. Hence, mandibular anterior teeth are greater in size in the faciolingual directions (to resist protrusive forces), and canines have different cross-sections than other anterior teeth and sustain lateral loads in more directions. In contrast, implants are round in cross-section, which is less effective in resisting lateral bending loads; consequently, increases in stress concentration occur in the crestal region of the jaws.
The elastic modulus of a tooth is closer to bone than any of the currently available dental implant biomaterials. On the other hand, titanium is more than 10 to 20 times more stiff than cortical or trabecular bone. The greater the flexibility (modulus) difference between two materials (metal and bone or tooth and bone), the greater the potential relative motion generated between the two surfaces upon loading.17 In addition, the greater the elastic modulus difference, the greater the stress concentrations where they first meet (the crest of the ridge). Hence, under similar mechanical loading conditions, implants generate greater stresses and strains (especially at the crest) to the bone compared with a natural tooth.
The precursor signs of a premature contact or occlusal trauma on natural teeth are usually reversible and include hyperemia and occlusal or cold sensitivity.22 This condition often results in the patient’s seeking professional treatment to reduce the sensitivity and usually is treated by occlusal adjustment and a reduction in force magnitude, which concomitantly decreases stress magnitude. This treatment most often reduces the hyperemia and the symptoms associated with this condition. If the patient does not have an occlusal adjustment, the tooth often increases in mobility to dissipate the occlusal forces. If an occlusal adjustment is performed, the tooth mobility will often decrease. Hence, the early warning signs and symptoms of excessive biomechanical load on natural teeth are often reversible and designed to protect the stomatognathic system. If the patient still fails to seek professional treatment for the increased mobility, the tooth may migrate orthodontically away from the cause of the occlusal stress.
The initial reversible signs and symptoms of trauma on natural teeth do not occur with endosteal implants. An absence of soft tissue interface between the implant body and bone results in the greatest portion of the force being concentrated around the transosteal implant–bone region.17 The magnitude of stress may cause bone microfractures and place the surrounding bone in the pathologic loading zone, causing bone loss or leading to the mechanical failure of prosthetic or implant components (e.g., porcelain fracture, abutment screw loosening).23 Unlike the reversible signs and symptoms exhibited by natural teeth, implant bone loss or unsecured restorations most often occur without any warning signs. Abutment screw loosening most often occurs within the first year of loading and is a symptom of biomechanical stress beyond the limits of the system. Marginal bone loss around the implant occurs without symptoms and is more likely during the first year. The loss of crestal bone around the implant is not reversible without surgical intervention and results in a decreased implant support and increased sulcus depth around the abutment. As a result, unless the density of bone increases after loading or the amount or duration of force decreases, the bone loss condition may progress until implant failure because the implants cannot move orthodontically away from the offending force.
The natural teeth and their periodontal ligament provide proprioception and early detection of occlusal loads and interferences. As a result, an occlusal premature contact greater than 20 microns may alter the path of mandibular closure to decrease the noxious elements of the premature, angled force.24,25 In addition, the jaw of a dentate patient almost stops before the food is penetrated and the maximum chewing force is applied. This is why a piece of bone in meat may shear off a cusp tip because the jaws did not reduce their velocity before the contact with the piece of bone. Implant prostheses do not have as much occlusal awareness as teeth during function. As a result, the bite forces used in mastication and parafunction can be of greater magnitude, and the path of closure is not altered with a premature contact.
Several studies confirm that teeth have more occlusal awareness and less force applied compared with an implant system. For example, Trulsson and Gunne compared three patient groups holding a peanut between the teeth for 3 seconds and then biting through the peanut.26 The natural teeth group had no problem holding the peanut or biting through it afterward. The denture group of patients experienced greater problems holding the peanut without its falling or becoming dislodged. The implant group had no problem holding the peanut in place. However, the implant and denture patients bit through the peanut with a force fourfold greater than the natural dentition group. The four times higher force in the implant patients is generated to the implant system, not the soft tissue of the denture group. Hence, the decrease in proprioawareness of implant patients can lead to a higher bite force during functional or parafunctional loading.
The proprioceptive information relayed by teeth and implants differs in quality awareness. Teeth deliver a rapid, sharp pain sensation under high pressure that triggers a protective mechanism. However, implants deliver a slow, dull pain that triggers a delayed reaction, if any.27 Implant occlusal sensitivity is uncommon; if it occurs, it signifies more advanced complications.
Radiographic evidence of occlusal trauma on teeth includes an overall increase in the periodontal membrane space and an increased radiopacity and generalized thickness of the cribriform plate around the tooth (not just localized at the crest).24,25 No generalized radiographic signs are apparent around an implant under excess occlusal force except at the crestal region, which demonstrates bone loss (but may be misdiagnosed as “biological width” or periimplant disease).10
The natural tooth can show clinical signs of increased stress such as enamel wear facets, stress lines, lines of Luder (in amalgam fillings), cervical abfraction, and pits on the cusps of teeth (Figure 31-6). An implant crown rarely shows clinical signs of increased biomechanical stress other than fatigue fracture. As a result, fewer diagnostic signs are present to warn the practitioner to reduce the stress on the implant support system.
Of all the opposing occlusal surfaces in the mouth, enamel opposing enamel has the most total volume wear (the sum total of both occluding surfaces).28 The enamel on a tooth will wear when repeated lateral forces or premature contacts are introduced on the system and may reduce the magnitude and angle of force for the premature contact. In comparison, a porcelain occlusal implant crown does not wear as rapidly or as much when a lateral premature contact is present.
The tooth slowly erupts into occlusion and is present in the mouth from childhood. The surrounding bone has developed in response to the biomechanical loads. Note there is no organized cribriform plate around the deciduous or permanent tooth until it is loaded. The permanent teeth are gradually introduced a few at a time while other teeth are present and bear the load. Hence, periodontal tissues organize gradually to sustain increasing loads, including those brought to bear by an attached prosthesis. The only progressive bone loading around an implant is performed by the dentist in a much more rapid time frame and intense magnitude of load.
When implants or teeth are subjected to repeated occlusal loads, microscopic stress fractures, work hardening, and fatigue may result. Fatigue fractures are related to the amount of stress and the number of cycles of load.17 The cementum and bone around a tooth root are able to repair the micro damage. Unlike the natural tooth system, the implant components, coping screws, or cement cannot adjust or repair to these conditions and ultimately fracture. The implant needs to perform its service for scores of years, which increases the cycles in the fatigue curve and the risk of long-term complications. As a result, forces from occlusion may result in subtle changes but may cause more serious long-term problems for survival as a result of bone or implant component complications compared with natural teeth.
The dentist uses mobility ratings to evaluate the quality of a natural abutment. A tooth with a Miller index mobility of 0 is considered “stronger” than a tooth with mobility of 2. Implants exhibit no clinical mobility compared with teeth. Phrases such as “solid as a rock” were used originally to describe their rigid fixation. As a result, the dentist may consider the implant a stronger abutment than a tooth, especially when the literature has implied that distal cantilevers off four anterior implants can be used to restore an entire arch.29
When considering stress factors, mobility is an advantage. The natural tooth, with its modulus of elasticity similar to bone, periodontal ligament, and unique cross-sections and dimensions, constitutes a near perfect optimization system to handle biomechanical stress. In fact, the stress is handled so well that bacteria-related disease is the weak link. An implant system handles stress poorly (capturing the stress at the crest of the ridge), with an elastic modulus more than 10 to 20 times that of surrounding bone, and is unable to increase mobility without failure, so that conditions related to biomechanical stress are the weakest link in the system. As a result, ways to decrease biomechanical stress are a constant concern to minimize the risk of implant system complications (Table 31-1).
TABLE 31-1
Tooth versus Implant Biomechanics
b. Short force duration (increased force impulse)
d. Implant is always rigid (mobility is failure)
e. Lateral force increases strain to bone
f. No fremitus
g. Radiographic changes at crest (bone loss); not reversible
a. Round cross-section and designed for surgery
b. Elastic modulus five to10 times that of cortical bone
c. Diameter related to existing bone
a. Occlusal trauma induces hyperemia and leads to cold sensitivity
b. Occlusal awareness of two to five times less
c. Functional bite force four times higher
4. Occlusal material: porcelain (metal crown)
An example of the higher biomechanical risk to the system is porcelain fracture on fixed prostheses. Whereas a crown on a natural tooth has a porcelain fracture risk of 3%, fixed prostheses supported by natural teeth have a 7% to 10% risk. On the other hand, in 2009, Kinsel and Lin found that porcelain fracture of implant crowns was as high as 34.9% of patients with bruxism compared with 17.2% of those without bruxism30 (Figure 31-7). In other words, implant patients are at higher risk of complications than those with natural teeth even if they are not bruxers. When the individual crown data were evaluated, men (with higher bite forces) had 13.1% of their implant crowns fracture compared with 6.4% of crowns in women. Clearly, the biomechanical load to the implant system is a greater risk for complications than natural tooth restorations.
No controlled clinical studies have been published comparing the various theories of occlusion on teeth, let alone on implants. Implant survival rates (not quality of health) reported by different practitioners are often within similar ranges even though the restoring guidelines differ. The myriad of variables in a patient population make case series studies of different implant occlusal philosophies impossible to conduct. These statements are not meant to decrease the importance of occlusion and a quest for accurate and precise relationships but instead attempt to encourage the profession to further develop this understanding.
Rather than addressing occlusal concepts or issues that result in early implant loss, crestal bone loss, and other prosthetic complications, a risk factor analysis is of benefit. For example, smoking is a risk factor for health. Not all smokers develop health problems from smoking, but the profession considers smoking a risk factor. Likewise, uncontrolled diabetes is a risk factor for periodontal health. That certain patients with diabetes have no periodontal disease does not negate diabetes as a risk factor. In a similar fashion, risk factors for implants and their related prosthesis must include biomechanical stress: the greater the stress, the greater the risk of complications. Therefore, conditions that decrease biomechanical stress should be established for treatment plans and occlusal designs in implant dentistry. For example, implant prostheses with extended cantilevers have some success; however, an increased risk of biomechanical complications exists.31
Biomechanical parameters are excellent indicators of the increased risk because they are objective and can be measured. The dentist can determine which condition presents greater risk and by how much the risk is increased. Hence, the occlusal concepts developed in this chapter by the author stem from biomechanical risk factors.32,33 In other words, if a clinical condition creates an increased biomechanical stress to the implant–prosthetic system, the dentist should implement mechanisms to decrease the stress.
Implant-Protective Occlusion
A proper occlusal scheme is a primary requisite for long-term implant prosthetic survival, especially when parafunction or a marginal foundation is present. A poor occlusal scheme increases the magnitude of loads and intensifies mechanical stresses (and strain) to the implant system. These factors increase the frequency of complications of the prosthesis and bone support. Crestal bone loss may lead to anaerobic sulcus depths and periimplant disease states. These conditions may also cause tissue shrinkage and loss of interdental papillae and poor esthetic conditions. All of these complications may be caused by biomechanical stress as a result of occlusal loads (functional or parafunctional).
The implant-protective occlusion (IPO) concept refers to an occlusal plan specifically designed for the restoration of endosteal implants, providing an environment for reduced biomechanical complications and improved clinical longevity of both the implant and prosthesis.32,33 The biomechanical rationale for this concept was published by the author after long-term clinical evaluation and biomechanical studies (and was originally called medial-positioned, lingualized occlusion).34 This concept was specifically designed for fixed prostheses in either partial or complete edentulous patients. Clinical considerations for this concept are drawn from basic prosthetic concepts, bone biomechanical principles, and finite element analyses to reduce noxious occlusal loads and establish a consistent occlusal philosophy.
A primary goal of an occlusal scheme is to maintain the occlusal load that has been transferred to the implant system within the physiologic and biomechanical limits of each patient. These limits are not identical for all patients or restorations. The forces generated by a patient are influenced by ranges of parafunction, masticatory dynamics, implant arch position and location, arch form, and crown height. The treatment plan philosophy for dental implants varies greatly and depends on these several parameters. The implant dentist can address these force factors best by selecting the proper position, number and implant size, increasing bone density when necessary by progressive bone loading, and selecting the appropriate occlusal scheme using stress-relieving design elements.
Implant and natural tooth position, number, size, and prosthesis design produce a myriad of possible combinations. However, consistent occlusal patterns may be established. The following guidelines are used to restore fixed implant–supported prostheses. A slightly different occlusal concept of the author is presented for complete dentures or removable prosthesis type 5 (RP-5) implant overdentures (implant and soft tissue support) and is called medial-positioned, lingualized occlusion (see Chapter 33).
The IPO principles for fixed prostheses address several conditions to decrease stress to the implant system, including existing occlusion, implant body angle to occlusal load, cusp angle of implant crowns, mutually protected articulation, cantilever or offset loads, crown height, crown contour, occlusal contact position, timing of occlusal contacts, and protection of the weakest component (Box 31-3).
Occlusal Considerations for Fixed Implant Prostheses
Existing Occlusion
Maximal intercuspation (MI) is defined as the complete intercuspation of the opposing teeth independent of condylar position, sometimes described as the best fit of teeth regardless of the condylar position.24 Centric occlusion (CO) is defined as the occlusion of opposing teeth when the mandible is in centric relation (CR).25 This may or may not coincide with the tooth position of MI. Its relationship to CR (a neuromuscular position independent of tooth contact with the condyles in an anterior, superior position) is noteworthy to the restoring dentist. The potential need for occlusal adjustments to eliminate deflective tooth contacts as the mandible closes in CR and the evaluation of their potential noxious effects on the existing dentition and the planned restoration is important to evaluate.
Correction of the deflective contacts before treatment presents many advantages and may follow a variety of approaches depending on the severity of the incorrect tooth position: selective odontoplasty (a subtractive technique), restoration with a crown (with or without endodontic therapy), or extraction of the offending tooth. The existing occlusion is best evaluated with facebow-mounted diagnostic casts on an articulator mounted with an open-mouth bite registration in CR. (This process was addressed in Chapter 16.)
Controversy exists as to the necessity to have MI harmonious with CO (CR occlusion). A vast majority of patients around the world do not have such a relationship, yet they do not exhibit clinical pathology or accelerated tooth loss. Therefore, it is difficult to state that these two positions must be similar. However, it is important to evaluate the existing occlusion and the mandibular excursions to consciously decide whether the existing situation should be modified or be maintained. In other words, dentists should determine whether they are going to ignore or control the occlusion of the patient (Figure 31-8).
Many dentists begin to evaluate the occlusion of the patient when the final implant prosthesis is delivered to the patient. However, this time frame is often too late to properly restore the patient (Figure 31-9). The underlying question that helps determine the need for occlusal correction before restoration of the implant patient is the observation of negative symptoms related to the existing condition. This may include temporomandibular joint (TMJ) conditions, tooth sensitivity, mobility, wear, tooth fractures, cervical abfraction, or porcelain fracture.24,25 The fewer and less significant the findings, the less likely an overall occlusal modification is required before restoration of the patient. However, to properly assess these conditions, the dentist must not ignore them before treatment.
As a general rule, the more teeth replaced or restored, the more likely the patient is restored to CO. For example, if a completely edentulous mandible is to be restored with an implant-supported fixed prosthesis, CO provides consistency and reproducibility between the articulator and the intraoral condition. The slight changes in occlusal vertical dimension (OVD) and its relationship to the position of anterior implant abutments to the direction of force may be studied and implemented on the articulator without the need to record a new occlusal vertical position on the patient. On the other hand, when one anterior tooth is being replaced, the existing MI position is often satisfactory to restore the patient even though a posterior interference and anterior slide into full interdigitation may be present (with little clinical variance from the ideal conditions). However, in a partially edentulous patient, the existing occlusion should be evaluated to determine if noxious conditions are present.
Premature Occlusal Contacts
A fundamental biomechanical formula is stress equals force divided by the area over which the force is applied (S = F/A).17 Therefore, during either maximum intercuspation or CO, no occlusal contacts should be premature, especially on an implant-supported crown. Premature occlusal contacts often result in localized lateral loading of the opposing contacting crowns.35 Because the surface area of a premature contact is small, the magnitude of stress in the bone increases proportionately (i.e., S = F/A). All of the occlusal force is applied to one region rather than being shared by several abutments and teeth. In addition because the premature contact is most often on an inclined plane, the horizontal component of the load increases the shear crestal stresses and the overall amount of stress to the entire implant system. The occlusal porcelain, the abutment screw, and the cement retaining the crown are all at increased risk because shear loads render more complications.
This is a general criterion for natural teeth, but the concept is much more important on implant prostheses with their higher impact force and less occlusal awareness for the several reasons previously addressed. Myata et al. evaluated premature contacts on implant crowns in monkeys (Macaca fascicularis).11 The crestal bone was histologically evaluated on implant crowns with 100 microns, 180 microns, and 250 microns of premature contacts for 4 weeks.12 The crowns with 100-micron premature contacts had little bone changes. The 180-micron group demonstrated a V-shaped pattern of bone loss for several millimeters. The 250-micron implant crowns for 4 weeks had a large V-shaped defect around the implants that extended for more than two thirds of the implant body (Figure 31-10). The implant is rigid, and the premature implant load cannot be released by increased mobility or occlusal material wear as with a natural tooth.
Isidor et al. evaluated excessive premature contacts on implants in monkeys over a 20-month period on eight integrated implants.13 Implant failure occurred in six of eight implants between 2 and 14 months. The implants that did not fail had greater bone density and crestal bone loss with osteoclastic activity within the threads of the implants (Figure 31-11). The premature contact on an implant system contributes to a higher risk of early abutment screw loosening, porcelain fracture, early loading failure, and crestal bone loss.
The elimination of premature occlusal contacts is especially important when habitual parafunction is present because the duration and magnitude of occlusal forces are increased. The elimination of premature contacts is more critical than in natural teeth because of the lack of proprioception and the implant inability to move and dissipate the forces. Because of increased proprioception, an initial premature occlusal contact on a tooth often affects the closure of the mandible to result in an MI position different from CO. A premature contact on an implant crown does not benefit from such protective features; as a result, the implant system is at increased risk. Therefore, occlusal evaluation in CO and MI and adjustment as necessary in partially edentulous implant patients are more critical than in natural dentition because the premature contacts can result in more damaging consequences on implants compared with teeth.36
Implant Body Orientation
Forces acting on teeth and dental implants are referred to as vectors (defined in magnitude and direction).17 Occlusal forces are typically three dimensional, with components directed along one or more of the clinical coordinate axes. The primary forces of occlusion can be resolved into a combination of components in any given plane. The same magnitude of force can have dramatically different effects on the implant system, solely because of the direction of the applied load. This is especially noted on implant support systems because they are more rigid.
The teeth are designed primarily for long-axis loads. The natural tooth roots in the majority of the mouth are perpendicular to the curves of Wilson and Spee. Although chewing is in an elliptical “tear drop” pattern, when the teeth finally contact, the forces are in the long axis of the roots, especially during power biting (Figure 31-12). The apical movement of teeth is minimal compared with their lateral movement. The maxillary anterior teeth receive a lateral load. The consequences of a lateral force to a tooth are reduced because of the increased tooth mobility, which decreases the effects of the lateral force component of a load.
Implants are also designed for long-axis loads. Two-dimensional finite element analysis by Binderman in 1970 evaluated 50 endosteal implant designs and found that all designs sustained stress contours concentrated primarily at the transosteal (crestal) region.37 In addition, less stress was observed under a long-axis load compared with angled loads. Since then, two- and three-dimensional finite element analyses by several authors have yielded similar results38–51 (Figure 31-13).
An axial load over the long axis of an implant body generates less overall stress and a greater proportion of compressive stress compared with an angled force to the implant body. When an implant body is loaded along its long axis, a 100-N force results with an axial force component of 100 N, and no lateral force component is observed. Therefore, the implant body should be positioned perpendicular to the curves of Wilson and Spee, just as with natural teeth.
Most anatomical variations of the bone (e.g., bony concavities) are located on the facial aspect and influence implant body inclination. An implant body may be positioned with a 15-degree angle to avoid the facial concavity and therefore is positioned at 15 degrees to the occlusal load. This angled implant may be restored during prosthetic reconstruction with a 15-degree angle abutment. From the level of the crest of the ridge to the occlusal plane, the implant abutment looks similar to one in an axial implant body. Hence, the laboratory technician and restoring dentist often treat the angled implant and axial implant in similar fashion. However, in the 15-degree angled implant body, the load to the facial bone increases by 25.9% compared with an axial load32 (Figure 31-14). If the implant surgeon places the implant body with a 30-degree angulation, the buccal force component of any occlusal load will result in a 50% increase of the load applied to the facial bone.32
Hence, the risk of crestal bone loss is increased with an angled implant.52,53 In addition, the greater force is applied to most of the implant system. The occlusal porcelain may be loaded in the long axis with the angled abutment, but the abutment screw loosening and implant component fracture risks increase in direct comparison to the load applied to the bone. Therefore, although the restoring dentist may place a 30-degree angled abutment and restore the case similar to the axial implant, the conditions and risks of early loading failure, crestal bone loss, and loose abutment screws are dramatically different (Figure 31-15).
Force Direction and Bone Mechanics
The noxious effect of offset or angled loads to bone is exacerbated further because of the anisotropy of bone. Anisotropy refers to the character of bone whereby its mechanical properties, including ultimate strength, depend on the direction in which the bone is loaded and the type of force applied. For example, cortical bone of human long bones has been reported as strongest in compression, 30% weaker in tension, and 65% weaker in shear54 (Figure 31-16). Porcelain, titanium components, and cements are also weakest to shear components of a load. Therefore, IPO attempts to eliminate or reduce all shear loads to the implant system because the bone, porcelain, titanium components, and cement are weakest to shear loads.
Any occlusal load applied at an angle to the implant body may be separated into normal (compressive and tensile) and shear forces. As the angle of load to an implant body increases, the amount of compressive and tensile forces is modified by the cosine of the angle. Hence, the force is slightly reduced. However, the angled component of force is a shear force, and the shear force is the amount of force times the sign of the load, which considerably increases the load. The force the bone observes is the sum of the compressive, tensile, and shear forces. For example, a 100-N force applied at 12 degrees off-axis will increase the total force to the bone by 100 N × cosine 12 degrees = 97.81 N + 100 N × Sine 12 degrees = 20.79 N. The total force is 97.81 N + 20.79 N = 118.60 N (or almost a 20% increase in total force).The greater the angle of load to the implant long axis, the greater the compressive, tensile, and shear stresses (Figure 31-17).
In finite element analysis, when the direction of the force changes to a more angled or horizontal load, the magnitude of the stress is increased by three times or more.51,52 In addition, rather than a primarily compressive type of force, tensile and shear components are increased more than 10-fold compared with the axial force. In a photoelastic block with implants inserted, the strain contours in the bone may be observed (Figure 31-18). The axial-loaded implants have less strain in the system (left side and lower right of figure). The angled implant has more strain lines indicating greater loads (right upper implant).
An angled load to the implant long axis increases the compressive forces at the crest of the ridge on the opposite side of the implant, increasing the tension component of force along the same side as the load. The greater the angle of force to the long axis of the implant body, the greater the potentially damaging load at the crest of the bone. For example, three-dimensional finite element analysis demonstrates that a vertical load on an implant with 100% bone contact may have compressive stress of 4000 psi (27.6 MPa) and almost no tensile stress at the bone-to-implant crest interface.51 With a load at a 45-degree angle on the same implant design, the compressive stress may increase to 14,000 psi (96.6 MPa), and on the opposite side, tensile stress may increase to 4000 psi (27.6 MPa). Hence, the compressive stresses are tripled, and the tensile stress increases 1000-fold with a load from a 45-degree angle.
The stress contours in the bone simulant of the three-dimensional studies resemble the clinical pattern of early crestal bone loss on implants. Therefore, not only does the magnitude of stress increase under angled loads, but it also evolves into a more noxious shear component, which is more conducive to bone loss and screw loosening.39 The greater the angle of the force, the greater the shear component. Bone is 65% weaker to shear load. Hence, the amount of the force increases, and the strength of the bone decreases. It has been reported that angled occlusal forces decrease the ability of successful bone repair on natural teeth. It may also impair successful bone remodeling around an implant.55
Not only is the bone weakest to shear loads, but forces applied at an angle to the bone also further affect the physiologic limit of compressive and tensile strengths of bone.54,56 A force applied at a 30-degree angle may decrease the bone strength limits by 10% under compression and 25% with tension (Table 31-2). A 60-degree force reduces the strength 30% under compression and 55% under tension. Therefore, not only does the crestal bone load increase around the implant with angled forces, but also the amount of stress the bone may withstand (i.e., the ultimate strength) decreases in shear, tension, and compression. The greater the angle of load, the lower the ultimate strength of bone. Therefore, IPO attempts to eliminate lateral or angled loads to an implant-supported prosthesis because the magnitude of the force increases and the strength of the bone decreases.
TABLE 31-2
Cortical Bone Strength Related to Angle of Load
Type | Strength (mPa) | Direction of Load |
Compression | 193 | Longitudinal |
173 | 30 degrees off axis | |
133 | 60 degrees off axis | |
133 | Transverse | |
Tension | 133 | Longitudinal |
100 | 30 degrees off axis | |
60.5 | 60 degrees off axis | |
51 | Transverse |
From Reilly DT, Burstein AH: The elastic and ultimate properties of compact bone tissue, J Biomech 80:393–405, 1975.
Barbier and Schepers histologically evaluated implants loaded in the long axis versus off-axis loading in dogs.
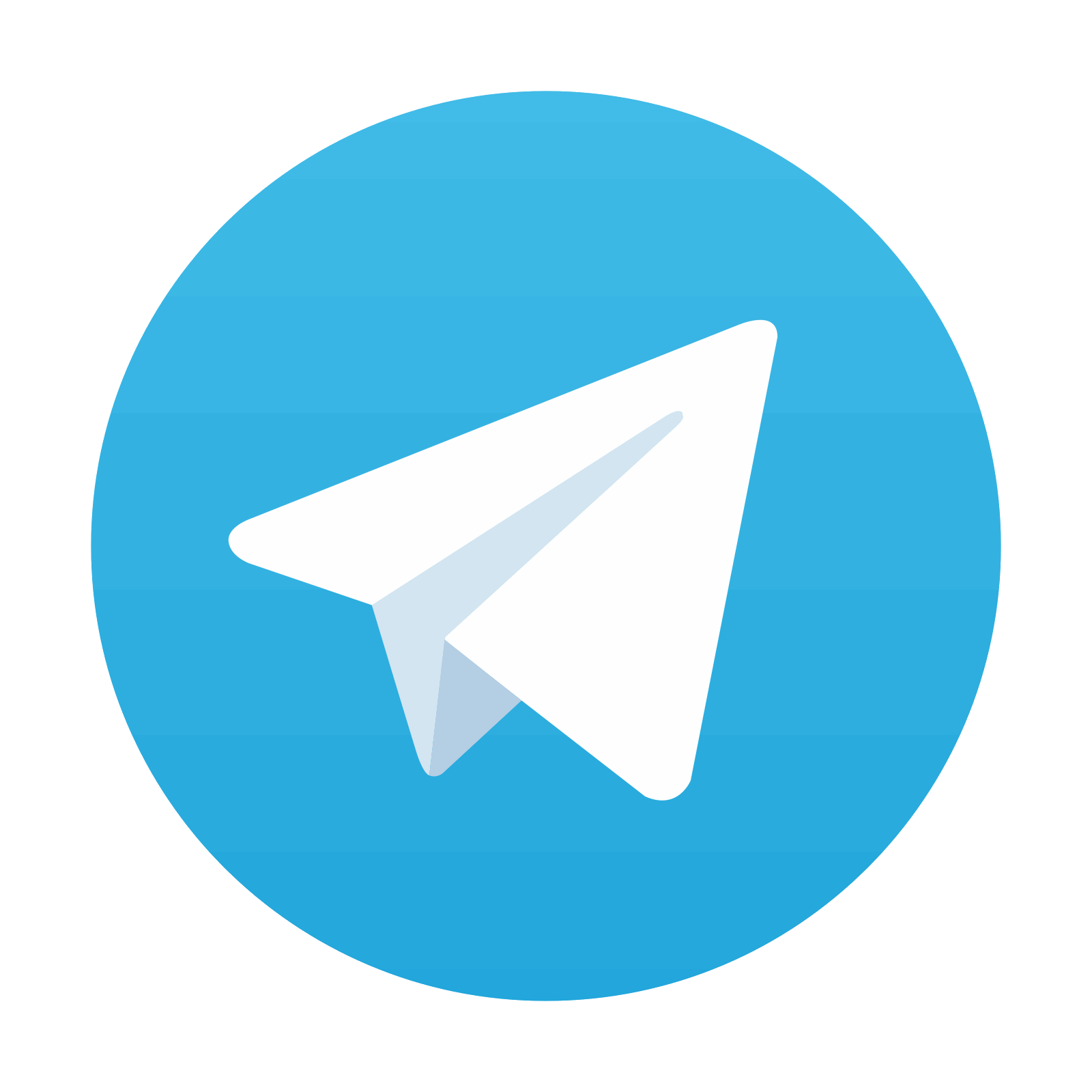
Stay updated, free dental videos. Join our Telegram channel

VIDEdental - Online dental courses
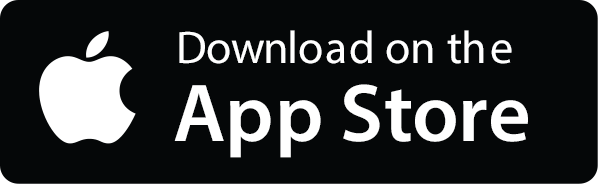
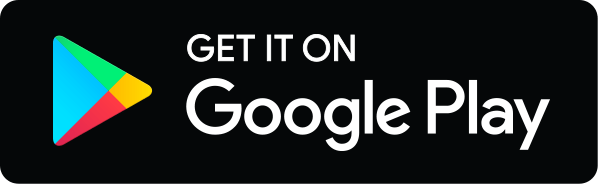