Pharmacology of Local Anesthetic Agents
2. Discuss the mechanism of actions of local anesthetics.
3. Describe the structure of local anesthetics.
4. Discuss the difference between esters and amides.
5. Discuss the properties and ionization factors of local anesthetics.
6. Describe the dissociation constant (pKa), and its effects on the onset of action of local anesthetics.
7. Discuss how infection in the area of local anesthetic administration decreases its efficiency.
8. Describe the differences between the membrane expansion theory and the specific receptor theory.
9. Discuss the pharmacodynamics of local anesthetic drugs.
10. Discuss the pharmacokinetics of local anesthetics, including onset of action, duration, absorption, distribution, biotransformation, the difference between esters and amides, and differences within the same groups.
11. Discuss the systemic effects of local anesthetic drugs on the central nervous system and cardiovascular system.
12. Correctly complete the review questions and activities for this chapter.
The period of time required to eliminate the amount of drug in the body by one-half of its strength.
Intermediate hydrocarbon chain
Determines if the local anesthetic is classified as an ester or an amide.
Introduction
The use of reversible local anesthetic chemical agents is the most common method to secure pain control in dental practice. Box 3-1 describes the properties of ideal local anesthetics. Not all local anesthetics that are available for use in dentistry today meet all of these properties, although many are clinically acceptable.
Chemistry
Local anesthetic agents are divided chemically into two major groups: the esters and the amides. The clinical significance of the two major groups is the potential for allergic reactions and the route of biotransformation. Ester local anesthetics have a high probability of producing an allergic reaction compared to amide local anesthetics. With ester local anesthetics, a patient who has an allergic reaction to one ester agent is likely to experience hypersensitivity to all ester anesthetics. This is less likely to occur within the amide local anesthetic group. Cross-hypersensitivity between esters and amides is unlikely. Due to the high degree of hypersensitivity to injectable esters, all injectable local anesthetics manufactured for dentistry today are in the amide group. Ester local anesthetics are hydrolized in the plasma by the enzyme pseudocholinesterase, and amide local anesthetics are biotransformed generally in the liver. Topical anesthetics are available in both the esters and amides. (See chapter 6.)
The local anesthetic molecule consists of three components: (1) the lipophilic aromatic ring, (2) intermediate hydrocarbon ester or amide chain, and (3) hydrophilic terminal amine (Figure 3-1). The lipophilic aromatic ring improves the lipid solubility of the molecule, which facilitates the penetration of the anesthetic through the lipid-rich membrane where the receptor sites are located. The greater the lipid solubility of the anesthetic molecule, the greater the potency of the drug. For example, the local anesthetic bupivacaine is more lipid-soluble than lidocaine, and it is therefore more potent because more of the anesthetic dose can enter the neurons. Because bupivacaine is more lipid-soluble and is more potent than lidocaine, it is prepared as a 0.5% concentration (5 mg/mL) rather than a 2% concentration of lidocaine (20 mg/mL).
The hydrophilic terminal amine (or acid cation form) may exist in a tertiary form (three bonds) that is uncharged and lipid-soluble (or base anion form) or as a quaternary form (four bonds) that is positively charged and renders the molecule water-soluble, which is how it is delivered from the dental hygienist’s syringe into the patient’s tissue. As explained earlier, the aromatic ring determines the actual degree of lipid solubility, but the terminal amine acts as an “on-off” switch allowing the local anesthetic to exist in either lipid-soluble or water-soluble configurations. Once the anesthetic is injected into the tissue, the quaternary amine dissociates into uncharged tertiary amine base and a hydrogen ion inorder to penetrate the lipid rich nerve membrane. The tertiary amine following penetration of the nerve will once again gain a hydrogen ion found within the axoplasm to successfully bind to the receptor sites. The tertiary and quaternary forms exhibit vital roles in the sequence of events leading to the conduction block1 (discussed later, see Figure 3-1).
Pharmacodynamics of Local Anesthetic Drugs
Pharmacodynamics refers to the physiological effects of drugs on the body and the mechanisms of drug action and its relationship between drug concentration and effect. Synthetic local anesthetics are prepared as weak bases and during manufacturing precipitate as powdered unstable solids that are poorly soluble in water. They are combined with an acid to form a salt (hydrochloride salt) to render them water-soluble; these can be dissolved in sterile water or saline, creating a stable, injectable anesthetic solution. The molecules exist in a quaternary water-soluble state in the cartridge. In the cartridge, the solution contains an equilibrium of positively charged (ionized) molecules, the acid or cation (RNH+)2 and uncharged (unionized) molecules, the base or anion (RN)1. The formula RNH+ ↔ RN + H+ represents the equilibrium between the two ions and H+ as the hydrogen ion2 (Figure 3-2). The equilibrium is dependent upon the pH of the solution and the pKa (how easily the compound becomes charged). Discussed next, also see Box 3-2.
The pH (the acid-base balance) of the solution is manipulated by the manufacturer to complement the specific molecular structure of each anesthetic. However, all local anesthetic solutions are acidic before injection. The lower the pH, the more acidic the solution, and the higher the pH, the more alkaline (basic) the solution. Local anesthetic solutions without vasoconstrictors range in pH from approximately 5 to more than 6; generally, preparations with vasoconstrictors are more acidic than plain formations because of the presence of the preservative (antioxidant) sodium bisulfite (see Chapter 4), and the pH ranges from approximately 3.3 to 5.5.3 Once injected, the hydrophilic (ionized) cation component which is acidic facilitates diffusion through the extracellular fluid to the nerve. However, the cation form will not penetrate the nerve. Therefore the time of onset of the local anesthetic is based on the proportion of the molecule that converts to the tertiary, lipid-soluble (unionized) base structure when exposed to the normal physiologic pH (7.4) of the body. Once injected into the tissues (pH 7.4), the amount of local anesthetic in the free-base unionized form will increase to provide greater lipid penetration of the nerve. The increase in the base molecules is due to the dissociation of the H+ ion from the quaternary molecule, now rendering it a tertiary base molecule that can penetrate the nerve. The dissociation constant (pKa) for the anesthetic predicts the proportion of molecules that exist in each of these states. By definition, the pKa of a molecule represents the pH at which 50% of the molecules exist in the lipid-soluble tertiary anionic (uncharged base) form and 50% in the quaternary water-soluble cationic (charged acid) form2 (see Box 3-2). As the pH of the tissues differs from the pKa of the specific drug, more of the drug exists either in its charged or uncharged form. This is expressed in the Henderson-Hasselbalch equation: pKa − pH = log [RH+] / [R] where [R] is the concentration of unionized (uncharged) drug and [RH+] the concentration of ionized (charged) drug. This is important because the molecular form of the anesthetic that allows diffusion through the lipid rich nerve membrane is the free base (RN) (anionic) portion of the molecule. Once the free base of the molecule reaches the axoplasm inside the nerve membrane, the amine gains a hydrogen ion and reverts back to the ionized quaternary (cationic) form (RNH+), the active form of the molecule that binds to the receptor site preventing the influx of sodium (see Figures 3-1 and 3-3).
Because the pH of the body tissue is 7.4, the ideal pKa of an anesthetic should be 7.4, indicating that 50% of the molecules are uncharged base and quick diffusion through the lipid membrane would occur. However, the pKa of all local anesthetics have values greater than 7.5 except topical benzocaine which is 3.5, and have a pH of approximately 5 to 6 in plain solutions and lower with vasoconstrictors; therefore a greater proportion of the molecules exist in the quaternary water-soluble form when injected into tissue having normal pH of 7.4.1 The higher the pKa of the anesthetic, the lower the concentration of uncharged base molecules. This causes slower diffusion into the nerve cell and a slower onset of action of the local anesthetic. Table 3-1 lists factors affecting local anesthetic induction time and action, and Table 3-2 lists dissociation constants (pKa) of commonly used local anesthetic drugs. So, the proportion of cations and base molecules are determined by the pH of the anesthetic, pKa of the anesthetic, and the pH of the tissue. In addition, the uncharged tertiary amine base and the positively charged quaternary amine cation each have different important biophysical characteristics that influence the ability of the local anesthetic to block the nerve impulse.
TABLE 3-1
Characteristics Affecting Local Anesthetic Induction and Action
CHARACTERISTICS | ACTION AFFECTED | EXPLANATION |
Concentration of local anesthetic | Diffusion and onset | Higher concentration = more readily molecules diffuse through the nerve = rapid onset. |
Dissociation constant (pKa) | Onset | Determines the portion of administered dose in the lipid-soluble state (RN). Lower pKa more rapid the onset of action. More RN molecules are present to diffuse through the nerve, decreasing the time of onset. |
Lipid solubility | Potency | Greater lipid solubility enhances diffusion through the nerve, allowing a lower effective dose. |
Protein binding | Duration | Increased protein binding allows more cations (RNH+) to bind to the receptor sites within the sodium channels prolonging the presence of anesthetic at the site of action. Bupivacaine is a good example of this characteristic. |
Perineurium thickness | Onset | Perineurium binds individual neurons together to form fasciculi. The thicker the perineurium, the slower the rate of diffusion and onset of action. |
Nonnervous tissue diffusibility | Onset | Increased diffusibility = decreased time of onset. |
Vasodilator activity | Anesthetic potency and duration | Greater vasodilator activity = increased blood flow to region = rapid removal of anesthetic molecules from injection site; thus decreased anesthetic potency and decreased duration. |
TABLE 3-2
Dissociation Constants (pKa) of Commonly Used Local Anesthetic Drugs
AGENT | PKA | PERCENT BASE (RN) AT PH 7.4 | APPROXIMATE ONSET OF ACTION (MIN) |
Lidocaine | 7.7 | 29 | 2–4 |
Mepivacaine | 7.7 | 33 | 2–4 |
Prilocaine | 7.7 | 25 | 2 |
Articaine | 7.8 | 29 | 2–4 |
Bupivacaine | 8.1 | 17 | 5–8 |
Procaine | 9.1 | 2 | 14–18 |
Topical benzocaine | 3.5 | 100 | 1 |
Topical tetracaine | 8.6 | 7 | 10–15 |
Source Malamed S: Handbook of local anesthesia, ed 5, St Louis, 2004, Mosby.
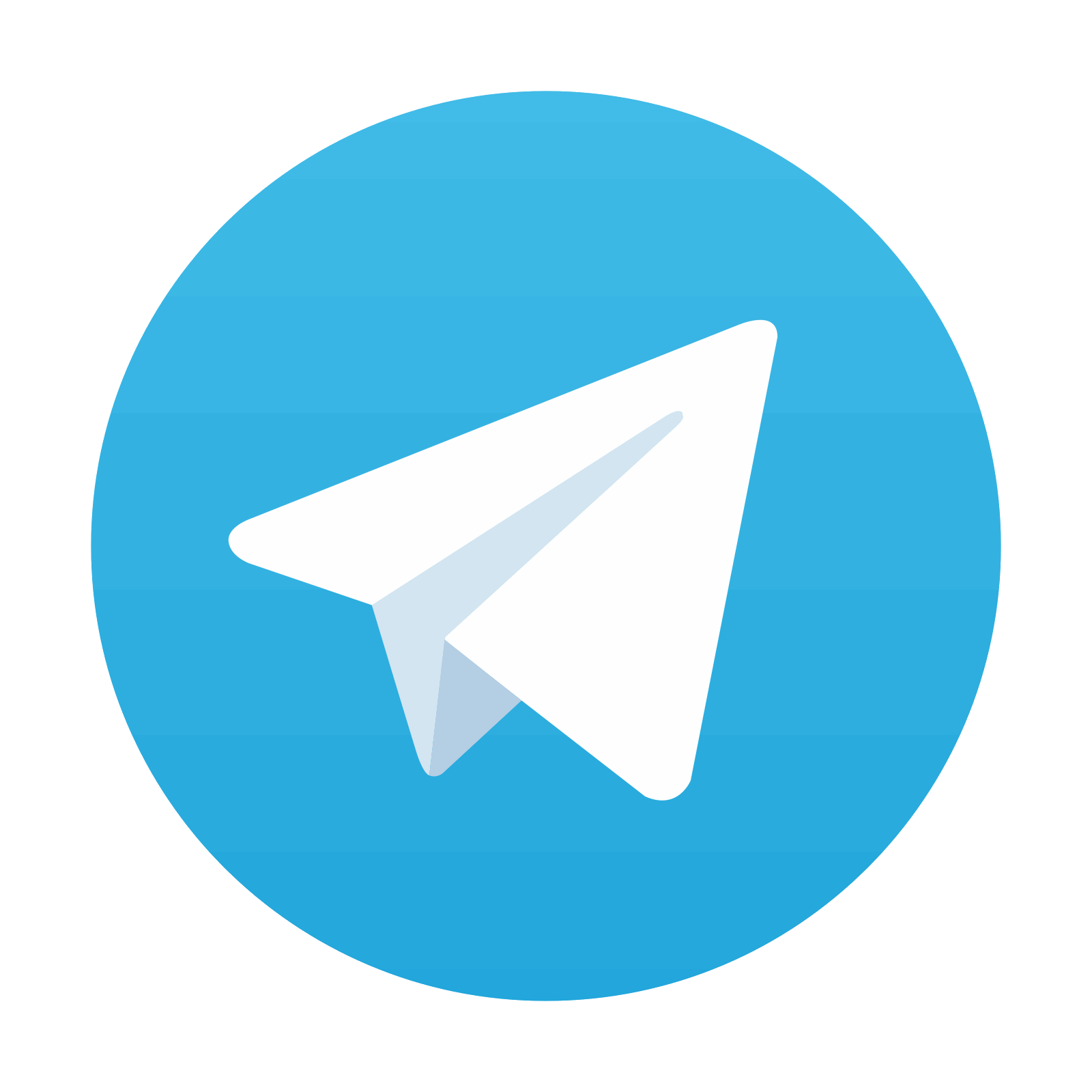
Stay updated, free dental videos. Join our Telegram channel

VIDEdental - Online dental courses
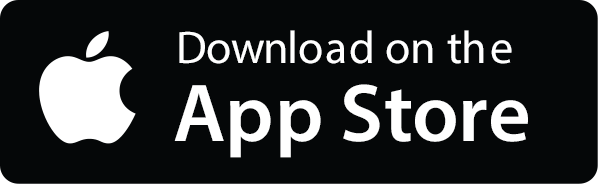
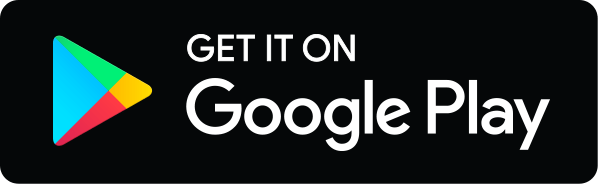