Biomechanical Factors in Surgical Orthodontics
R. Scott Conley, Harry L. Legan
When using orthognathic surgery, application of appropriate diagnosis, treatment planning, biomechanical principles, surgical technique, and timing are all necessary to optimize individual patient treatment outcomes. From the discipline’s inception in the mid-nineteenth century through its peak in the 1980s and its continued evolution in the early 2000s, advances have been made in both diagnostic and treatment-related areas. Initial updates made orthognathic surgery a safer and more practical treatment alternative with the development of antibiotics, enhanced surgical techniques, and later rigid fixation. The improved surgical techniques enabled orthodontists to rethink the timing and type of surgical preparation and ultimately enabled orthognathic surgery to become increasingly predictable, with dramatic facial and occlusal improvement the normal outcome.
More recently, diagnostic advances such as cone beam computed tomography (CBCT) and computer aided surgical simulation (CASS) offer tremendous value and new planning opportunities to the orthodontist, the oral and maxillofacial surgeon, and their patients. Both techniques now enable the care team to truly understand the three-dimensional (3D) nature of patient jaw deformities to better simulate and plan treatment before undertaking care. While planning is essential, the greater benefit is the “failure analysis” from the 3D images, which enhances future patient care by preventing adverse outcomes.
Advances have not been restricted to the planning phase but have also included the development of new treatments such as distraction osteogenesis, surgery for the obstructive sleep apnea (OSA) patient, mandibular constriction, earlier surgical intervention, and skeletal anchorage with temporary anchorage devices (TADs) used before, during, and following surgery, each of which potentially alter the biomechanical approach and surgical intervention planned for the patient. While distraction osteogenesis is unlikely to replace traditional orthognathic surgery because, for example, it cannot be used in cases of excessive jaw growth, it offers a treatment alternative for correction of skeletal jaw deficiencies. Unfortunately, few papers describe the detailed biomechanical approach necessary to achieve the correct vectors and movements for optimal treatment results. Orthodontic appliance and TAD placement, localizing the center of resistance of the osteotomized segments, and location of force application are vitally important to minimize treatment complications and enhance treatment outcomes. The same efficient, effective, operator-determined biomechanical principles necessary to produce excellent orthodontic outcomes must be applied to both distraction osteogenesis and TADs. The biomechanical principles are the same whether focusing on tooth-to-tooth movement or bony segment–to–bony segment movement yet few recognize the merging of these disciplines.
Logical, sequential, and appropriate application of these and other related updates and their impact on diagnosis, treatment planning, surgical considerations, methods of efficient leveling, arch coordination, space closure, and other aspects of orthodontic mechanotherapy are discussed in this chapter.
Three-Dimensional Imaging: Cephalometrics
Cephalometric analysis is one critical step in proper diagnosis and treatment planning for patients with severe skeletal malocclusion. A myriad of analyses are available1 and preferences vary among orthodontists and surgeons. The most frequently used orthodontic analyses use angular measures,2,3 which are sufficient for assessing tooth position but are far less precise in accurately assessing jaw position and impossible to translate to the surgical setting. These analyses also use intracranial reference systems (such as porion and orbitale) that are difficult to locate, which has the potential to introduce more error than using an operator-assisted natural head position.4 To overcome these deficiencies, a cephalometric analysis was developed5,6 that uses predominantly linear measures that are directly transferable to the surgical setting, uses a natural head position, and can be altered surgically, orthodontically, or with a combined approach. Unfortunately, the improved lateral cephalometric analysis still neglected the transverse (or third dimension), which is highlighted by two significant publications on posterior-anterior (PA) cephalometry.7,8 While the PA view adds beneficial information, it is fraught with both exposure errors, such as minute patient rotations,9 and landmark identification and superimposition issues that make the PA cephalogram difficult to read and interpret.10
With CBCT, the deficiencies of both lateral and PA cephalometric analyses can be overcome. Several papers11–14 have shown that two-dimensional (2D) radiographs can be accurately created from the CBCT scan. Creating 2D projections of a 3D structure represents a diagnostic enhancement but developing a true 3D structural analysis that complements the 3D clinical exam and an improved asymmetry analysis would be a much more significant advancement. New 3D techniques such as “mirroring,” where the normal side is projected onto the abnormal side, enable the orthodontist and surgeon to precisely recreate what will be performed in the operating room (Fig. 23-1). While these methods are in their infancy and further validation is required,15–18 time will likely lead to substantial improvement in these areas.
Figure 23-1 The patient’s left side is the “normal” side and the patient’s right side demonstrates a significant hemimandibular hypertrophy. For treatment planning purposes, the “mirror” function within Dolphin was used to overlay (in pink) the “normal” on the affected side to illustrate the amount of bone that will need to be removed for symmetry to be achieved. While this technique is extremely illustrative, it is typically not possible to remove this amount of bone surgically due to the location of the inferior alveolar nerve on the affected side.
Improved Three-Dimensional Surgical Planning
CBCT not only provides additional information during diagnosis, but also can be used to improve the operative treatment plan. Historically, virtual treatment objectives were created using lateral cephalometric radiographs, photos, dental models, and model surgery. However, this technique is subject to several different sources of error, including facebow registration, model mounting, landmark identification, and poor splint construction. To avoid these complications some surgeons took medical computed tomography (CT) scans, created 3D models, and performed surgery on a plastic model. Now, the presurgical CBCT can be merged with a scanned copy of the patient’s models and the surgery can be performed virtually on the computer.19–21 The surgeon is free to simulate several different surgical plans, including single-piece Le Fort I osteotomy, multisegment Le Fort I osteotomy, mandibular ramus osteotomies, and genioplasties. A 3D summary of the surgical movements is developed and the interim and final surgical splints can be fabricated. The surgical splints can also incorporate stents that will accommodate the precise positioning of the rigid fixation (Fig. 23-2).
Figure 23-2 A–C, This patient with juvenile idiopathic arthritis presented at the presurgical visit with extreme facial asymmetry. Using the computer aided surgical simulation (CASS) technique, the surgeon was able to accurately determine the final position of the maxilla and fabricate the large interocclusal splint required to set the maxilla in the proper position. The next step was to remove the interim splint, complete the mandibular osteotomy, and finally reposition the genial segment (if necessary). (Courtesy Dr. Sean P. Edwards, Ann Arbor, MI.)
Using immediate postoperative CBCT scans, the shape and form of the fracture that is propagated during the sagittal split ramus osteotomy (SSRO) can be evaluated (Fig. 23-3).22 This is important, as the muscular influence on the proximal and distal segments varies considerably based on where the fracture pattern occurs and directly influences the surgical stability and success. The authors report only about half of the fracture lines propagated along the line originally described by Hunsuck,23 even though the surgeon and surgical technique were consistent. They ultimately concluded that the enhanced imaging technique was suitable for analyzing surgical outcome and “has the potential to provide substantial data on the position of the proximal segments as a result of the lingual fracture line.”22
Figure 23-3 The lingual fracture pattern created during the sagittal split ramus osteotomy (SSRO) is considered to be one factor associated with relapse. It was impossible to observe the fracture pattern with conventional two-dimensional imaging techniques but this can be visualized clearly with cone beam computed tomography (CBCT). Additional information such as this can now be used to better understand the possible mechanisms behind unstable or poor treatment results. The goal will be to use this new information prospectively to minimize future negative outcomes. LSS1-4 indicates “lingual sagittal split” for patients 1 to 4. (Reprinted with permission from Plooij JM, Naphausen MT, Maal TJ, et al. 3D evaluation of the lingual fracture line after a bilateral sagittal split osteotomy of the mandible. Int J Oral Maxillofac Surg. 2009;38[12]:1244–1249.)
Another factor in surgical planning is maintaining condylar position during surgery, which is particularly difficult since it is affected by many factors, such as rotational movement of the proximal segment, tensional balance of the surrounding muscles, fixation method, and the surgeon’s experience.24 The ability to detect and assess for these changes following surgery is nearly impossible with 2D imaging but easy with CBCT. Proposed methods for avoiding condylar displacement include intraoperative monitoring by sonography, guiding splints, and intraoperative awakening.25–29 Because there is substantial evidence of the effect of condylar position on postoperative stability following SSRO,30–33 presurgical planning and accurate positional assessment of the condyle during and immediately following orthognathic surgery are vital to maximize surgical stability and achieve predictable and favorable treatment outcomes.
Three-Dimensional Surgical Outcome Evaluation: Managing Complications
Surgeons and orthodontists plan each case meticulously to optimize outcomes and minimize complications; however, complications are an unavoidable part of care. CBCT can enhance the clinician’s understanding of the root cause, enabling the team to select the most appropriate correction. Options range from adjusting the postsurgical orthodontic course, which is preferred when complications result from a less ideal presurgical orthodontic setup, to additional surgery when complications result from an unexpected skeletal change. Recently a small case series34 suggested that CBCT may be useful in the management of unfavorable fractures during mandibular surgery. Following unfavorable surgical outcomes, the authors reviewed both presurgical and immediate postsurgical CBCT to examine whether anatomic, surgical, or combined influences could explain the unfavorable splits. The case illustrated in Figure 23-4 involves a patient who was planned for a combined four first premolar extraction pattern and a surgical mandibular advancement. The initial surgical change was quite favorable; however, at the 12-week postoperative follow-up the patient had developed an open bite. To determine the cause, the presurgical, 6-week, and 12-week postoperative CBCT scans were superimposed, showing a dramatic and unfavorable proximal/distal segment rotation. Whether this resulted solely from the muscular influences, fixation difficulties, or poor segment positioning is unclear; however, it is clear that the change resulted at the surgical site rather than from the orthodontic treatment. The patient was given the option to undergo surgical revision to remove the existing fixation, reorient the segments, and replace the fixation or to undergo a potentially unstable orthodontic correction with elastics. Ultimately the patient opted for the “conservative” orthodontic approach but the CBCT enabled everyone to see the root cause and select the most ideal treatment plan.
Figure 23-4 A–C, Preoperative intraoral views of a patient prior to sagittal split ramus osteotomy (SSRO) to advance the mandible. Note the overbite present. D–F, Twelve-week postoperative intraoral views showing the anterior open bite that developed between the 8- and 12-week postoperative evaluations. G, Superimposed (pink) view of the presurgical (white) and 12-week postoperative (red) cone beam computed tomography (CBCT) scans. The superimposition depicts the amount of mandibular advancement but also clearly indicates (arrows) the rotation of the proximal and distal segments creating both a “V” notch deformity and the anterior open bite.
Maxillary Orthognathic Surgery
Several reasons exist for surgical manipulation of the maxilla in all three planes of space. Surgical procedures include maxillary impaction, maxillary advancement, segmentalization of the maxilla, and, more infrequently, maxillary inferior repositioning to lengthen the face. In addition to the direction of movement, the level of the osteotomy must be considered, with options ranging from Le Fort I to Le Fort III with several variations. With each type of surgical intervention, the presurgical and postsurgical orthodontic mechanics must be appropriate for the type of surgery planned, since inappropriate mechanics can reduce the facial improvement and in some cases prevent the proper surgery from occurring. Complete diagnostic records (CBCT or lateral and PA cephalogram, models, photographs, hand-wrist x-ray, temporomandibular joint examination) must be obtained and examined in conjunction with a detailed clinical examination to accurately develop the surgical orthodontic database and determine the appropriate mechanics plan for each case. The orthodontic biomechanical considerations for several of the more common surgical procedures are presented below.
Impaction
A common indication for maxillary surgery is vertical maxillary excess. Some of the cephalometric indicators within the cephalometric analysis for orthognathic surgery (COGS)5,6 are increased upper and lower facial height (N-ANS and ANS-Gn), increased mandibular plane angle (MP-HP), increased posterior facial height (N-PNS), increased gonial angle (Ar-Go-Gn), increased facial height ratio (N-ANS/ANS-Gn), and divergent occlusal planes. A typical clinical presentation includes an increased tooth-to-lip relationship, increased gingival display, an increased interlabial gap, and a relative mandibular deficiency. The patient will often have an anterior open bite but this is not always present due to dentoalveolar compensations and hypereruption of the teeth.
Just as the surgical plan must include all three planes of space, the orthodontic biomechanics must evaluate the patient’s needs and plan the mode of correction in three dimensions. Of special consideration with vertical maxillary excess is minimizing the orthodontic extrusion that can occur quite rapidly with mechanics, such as the placement of low modulus continuous archwires. Segmented arch mechanics provide an excellent way to predictably control the point of force application and magnitude of force applied and to produce an operator-determined, not appliance-determined, force system. The segmented arch technique has the additional advantage of being able to level each arch without requiring additional arch length.35
When divergent occlusal planes exist, a treatment occlusal plane must be selected first and then the appropriate force system designed. Typically a functional occlusal plane (perpendicular to the maxillary posterior teeth) is drawn. Once selected, the anterior teeth may be on, significantly above, or significantly below the treatment occlusal plane. Multiple options regarding arch leveling procedures exist. Should the arches be leveled before, during, or after surgery? Should the leveling be accomplished orthodontically, surgically, or using a combination of the two? The surgical approach to arch leveling is discussed below in the section on maxillary arch segmentalization.
If the maxillary anterior teeth have erupted significantly beyond the treatment occlusal plane, an extremely efficient orthodontic mechanism for leveling the arch is the intrusive base arch. Two stainless steel posterior segments containing the second molar to the first premolar and an anterior stainless steel segment from the lateral incisor to the lateral incisor are fabricated. Either a 0.017-inch × 0.025-inch beta-titanium or a 0.017-inch × 0.025-inch stainless steel base arch with helices is activated to deliver approximately 20 to 25 g of intrusive force per maxillary incisor (Fig. 23-5). The extrusive forces in the posterior segment and the moment of the force created by the base arch are balanced by using a high-pull headgear oriented through a point above and in front of the center of resistance of the maxilla. Without the high-pull headgear setup to balance the adverse effects of the intrusive base arch, a significant steepening of the maxillary posterior occlusal plane can be observed, which can exacerbate the initial clinical presentation of a patient with vertical maxillary excess. In addition, no true anterior intrusion at the level of the center of resistance may occur. Once the anterior and posterior segments are oriented along the same plane (the treatment occlusal plane), a continuous archwire is placed to maintain the arch leveling.
Figure 23-5 A–C, A 0.017-inch × 0.025-inch stainless steel intrusive base arch has two and a half helices immediately adjacent to the maxillary first molar. To minimize the side effect of incisal proclination resulting from the application of the intrusive force labial to the center of resistance of the tooth, the base arch is tied back with a stainless steel ligature to the molar band. A Dontrix gauge is used to accurately measure the force magnitude.
In other vertical maxillary excess patients, the maxillary anterior segment may be more superiorly positioned than the posterior occlusal plane. In this situation the exact opposite orthodontic force system is designed. The segments of teeth are set up in the exact same orientation. The difference is that instead of an intrusive force being applied to the maxillary anterior teeth, an extrusive force of approximately 50 g per tooth is applied to extrude the anterior teeth to the level of the posterior occlusal plane. Once again, when all the teeth are oriented along the treatment occlusal plane, the extrusive base arch is removed, and a continuous stainless steel archwire is placed (Fig. 23-6).
Figure 23-6 Extrusive base arch is essentially the same as intrusive base arch, merely turned upside down. A, Extrusive base arch activated but not ligated to the anterior segment. B, Ligated to the anterior segment. C, Helices in a lateral view. The helix is made so that when the arch is activated, the loop is wound rather than unwound, which results in better load deflection and more constant force delivery.
Many surgeons will perform intermaxillary fixation, orienting the maxilla to the mandible and autorotating the entire maxillomandibular complex to the desired vertical position, and then use rigid fixation. This will typically cause a slight maxillary advancement in addition to the impaction. With vertical maxillary excess, a mandibular osteotomy may or may not be required but attention must still be directed to the setup of the mandibular dental arch to ensure appropriate arch coordination, arch form, and leveling. Within the mandible, attention is directed to the depth of the curve of Spee, lower incisor–menton measurement, and the relative amount of prominence desired at both the soft and the hard tissue pogonion. For specific information on leveling of the mandibular arch, see the section “Mandibular Surgery” later in the chapter.
Advancement
The correction of any severe jaw deformity involved a mandibular osteotomy until the mid-1970s when Bell et al.36 performed a series of microangiographic studies. Those studies demonstrated the biological basis for surgical manipulation of the maxilla and since then the maxilla has been diagnosed much more frequently as part of the etiology of the Class III malocclusion.37 Some of the COGS indicators of a maxillary sagittal deficiency with a normal mandibular position include glabella-subnasale (G-Sn), nasion–A point (N-A), nasion–B point (N-B), nasion-pogonion (N-Pg), and glabella–soft tissue pogonion (G-Pg′). Other clinical indications for performing a maxillary advancement in lieu of a mandibular setback include decreased pharyngeal airway, excessive submental adipose tissue, decreased malar convexity, and increased nasolabial grooves on smiling. A helpful clinical diagnostic aid involves using an object, such as a hand, fingers, or cardboard, to “block out” the midface while examining the projection of the lower face. If the chin position is acceptable, it is most likely that the patient has a maxillary sagittal deficiency.
Patients with maxillary sagittal deficiency also frequently exhibit varying degrees of transverse deficiency and may present with a posterior crossbite as part of their Class III malocclusion. The crossbite must be evaluated critically to determine the magnitude and etiology using PA cephalogram (or CBCT) in addition to the clinical examination and models analysis. During the analysis, the transverse dimension must be evaluated in not only the current but also the final sagittal position because the crossbite may not need to be addressed once the maxilla is brought forward. If the patient exhibits an absolute crossbite38 or has a crossbite in both the presenting and the final position, the magnitude and classification (skeletal versus dental) of the transverse deficiency must be determined. Skeletal crossbites are best corrected skeletally (rapid palatal expansion [RPE], transverse maxillary distraction osteogenesis, or segmented maxillary surgery), while dental crossbites may be corrected skeletally or dentally (RPE, palatal arch, cross-arch elastics, or expansion of the maxillary archwire).
Occlusogram analysis,39,40 in addition to model analysis, can assist in the differential diagnosis of the transverse dimension. The occlusal aspect of the teeth is traced on a piece of acetate and articulated in the current anteroposterior occlusion. To simulate the surgery, the maxillary member is brought forward the same amount as the anticipated surgical maxillary advancement. Then, using the mandible as the template arch, the appropriate arch form, arch width, and arch length analysis is performed, with the difference between the current and predicted arch width representing the required amount of maxillary expansion or mandibular constriction.
If the etiology of the crossbite is dental or small in magnitude, a transpalatal arch (TPA) provides an efficient method of dental arch expansion and does not require patient compliance. The clinician needs to fabricate a 0.036-inch stainless steel heat-treated TPA (Fig. 23-7). First, the appliance should be fitted passively to ensure that no unintended forces, moments, or vertical couples are present. To activate the TPA, the appliance should not only be expanded but also have buccal root torque placed to reduce the tipping tendency. It is essential to apply an equal amount of buccal root torque bilaterally to prevent a significant vertical couple being produced, which if left in place long enough can result in an iatrogenic occlusal plane cant that can be resolved only by differential vertical positioning of the maxilla as it is brought forward. Iatrogenic cants transform a straightforward maxillary advancement to a more technically precise surgery and, worse, can result in an unfavorable outcome and a dissatisfied patient.
Figure 23-7 A, For expansion, a transpalatal arch (TPA) with an omega adjustment loop can be used. The loop introduces more flexibility to the TPA but the advantage is that the wire can be maintained at the same depth within the palate after each activation. Without an adjustment loop the TPA will be brought more occlusally after each activation and increase the potential for tongue irritation and alteration in speech. B, Activated TPA.
For the patient with excess transverse dimension, using a TPA with constriction is an efficient way to reduce arch width. Archwires are far less efficient in these cases because of their smaller cross-sectional dimension and because much arch activation is lost in the act of inserting the wire into the buccal tubes. To be most effective, the TPA must be “overconstricted” and heat treated to prevent a similar loss of activation upon insertion. Palatal root torque must also be placed to minimize tipping of the posterior teeth or posterior segments palatally.
Skeletal maxillary transverse deficiency is typically larger in magnitude and can be measured from the PA cephalogram or CBCT by evaluating both the jugal process (J point) width and the axial inclination of the posterior teeth. In a skeletally mature patient the most stable method41,42 of correcting the maxillary transverse deficiency is maxillary transverse distraction osteogenesis (also referred to as surgically assisted RPE) or segmentalization of the maxilla (see discussion below).
Patients with maxillary sagittal hypoplasia may also have significant arch length deficiency, which can be assessed using the previously discussed occlusogram analysis, which further allows the clinician to determine the anchorage requirements should extractions be necessary. Occlusogram analysis will provide different anchorage requirements based on where the upper member is placed. If the upper member is left in the pre-treatment position, simulating an “orthodontics-only treatment approach,” the anchorage requirements will be the opposite of what is required when the maxilla is advanced surgically. Extractions are performed in surgical orthodontic cases primarily for two reasons: to ideally position the teeth within the basal bone and to resolve significant arch length deficiency. The extraction mechanics should not be directed toward orthodontic correction of the malocclusion; instead, the extraction mechanics should be directed toward making the occlusion reflect the magnitude of the skeletal discrepancy.
The type of extraction mechanics selected should be tailored to meet the specific needs of each case. Cases with significant maxillary anterior crowding are best managed with early extraction of the first bicuspids and alignment of the posterior segments and canines only, bypassing the incisors using a 0.017-inch × 0.025-inch stainless steel track. Once aligned, a segmental precalibrated 0.017-inch × 0.025-inch beta-titanium “A” anchorage T-loop is placed from the first molar to the canine to perform initial canine retraction. “A” anchorage is achieved by placing four 30- to 40-degree moment activations in the posterior leg of the T-loop and three moment activation bends in the anterior leg. The differential moments produce a moment/force ratio of 10 : 1 (translation) at the canine versus a moment/force ratio of 13 : 1 (root movement) at the posterior segment. In critical anchorage cases, a high-pull headgear, TPA, or both will be placed to assist in maintaining the position of the posterior teeth and to prevent anchorage loss. If all of the extraction space is required for arch length resolution once the canine is brought back, the maxillary incisors are aligned and there is minimal to no space remaining. If the anchorage requirements are less stringent, the canines may be brought back only enough to allow adequate space for alignment of the maxillary anterior teeth with any residual space closed with a new segmental T-loop activated for “B” or “C” anchorage (Fig. 23-8).43,44
Figure 23-8 A, Preactivated 0.017-inch × 0.025-inch beta-titanium T-loop. The anterior leg is more gingival due to the placement of a gingival horizontal tube on the canine. The loop is contoured to the arch and checked for passivity both anteriorly and posteriorly. B, Then, depending on the anchorage requirements, the moments are placed within the anterior and posterior legs. C and D, Clinical use of an active segmental T-loop.
Inferior Repositioning (Down Graft)
Vertical maxillary deficiency is much less common than its counterpart, vertical maxillary excess. Some of the cephalometric indicators within COGS are decreased lower facial height (ANS-Gn), decreased mandibular plane angle (MP-HP), decreased gonial angle (Ar-Go-Gn), increased facial height ratio (N-ANS/ANS-Gn), and deep overbite. A typical clinical presentation includes a decreased tooth-to-lip relationship, decreased gingival display, no interlabial gap, and a relative mandibular prognathism and/or prominent chin button.
Much as with vertical maxillary excess, most attention is directed to the vertical relationship of the jaws, with posterior extrusion the primary goal of the presurgical orthodontic mechanics. Continuous low modulus archwires may be used to facilitate orthodontic eruption; however, in many of these short face, low angle, or convergent patients presurgical or nonsurgical orthodontic extrusion is extremely difficult to achieve. Extrusive force systems may be placed with continuous low modulus archwires but often the heavy occlusal bite force of the patient will exceed the eruptive force capabilities of the archwire. Instead, extrusive base arches (see Fig. 23-6) can be fabricated to produce an extremely efficient eruptive force (often 200 g or more) to the posterior maxillary and/or posterior mandibular segments while hyalinization of the anterior teeth occurs, producing minimal to no anterior intrusion. Additional benefits derived from the extrusive base arch force system include possible arch length increase resulting from the crown distal or counterclockwise moment and, if not tied back, space will develop between the segments (Fig. 23-9). Once leveling has occurred, this space can be used to retract the maxillary incisors using an “A” anchorage segmented arch T-loop. While the anterior crossbite will worsen presurgically, the maxillary anterior teeth will be appropriately positioned and uprighted within the maxillary basal bone. If simultaneous uprighting of the maxillary incisors during leveling is desired, the extrusive base arch can also be fabricated as a “split” base arch where the force can be applied behind the center of resistance of the maxillary anterior segment.
Figure 23-9 A–C, Split intrusive base arch allows for precise activation when intruding segments of teeth. The distal extension from the anterior segment allows the operator to place the intrusive force anterior to, at, or posterior to the center of resistance for the segment. This allows for simultaneous intrusion with proclination, intrusion with no axial change, or intrusion with simultaneous uprighting of the anterior segment for increased efficiency, respectively.
An alternative method is performing the orthodontic leveling postsurgically, especially when a significant mandibular curve of Spee is present. Immediate postsurgical leveling is extremely effective in part because of the surgically induced change in muscle fiber orientation, which results in a decreased mechanical advantage and decreased bite force (Fig. 23-10). In addition, Frost described a regional accelerative phenomenon due to healing and increased vascularization.45,46 To assist eruption of the teeth, a continuous light stainless steel archwire with vertical box elastics in the midarch, segmented arch mechanics with an extrusive base arch, or an overlay extrusive base arch can be placed with a light continuous main archwire. Once complete arch leveling is obtained, a rigid continuous stainless steel archwire can be placed to maintain the arch leveling.
Figure 23-10 A–C, This patient is 6 weeks post–mandibular advancement with tripoding. The posterior occlusion remains open because the interocclusal splint was just removed. D–F, Six weeks later, following inter-arch vertical elastics with a heavy 0.019-inch × 0.025-inch stainless steel maxillary wire and a “light” 0.016-inch × 0.022-inch stainless steel wire, the patient has nearly completely interdigitated posterior teeth and can begin final finishing orthodontic care.
Segmentalization
Two main reasons exist for segmental maxillary osteotomies, one being multiple maxillary occlusal planes. The discrepant occlusal planes can be managed orthodontically or surgically and the decision regarding which method is better is largely philosophical and based on the stability of the surgical intervention versus the stability of the vertical orthodontic movement.47,48 Segmentalization allows for differing amounts of superior or inferior repositioning of the segments surgically. When surgical closure of an anterior open bite is planned, orthodontic eruption of the maxillary anterior teeth is undesirable because this will introduce potential instability to the case and reduce the amount of vertical surgical repositioning. To facilitate segmentalization of the maxilla, segmental or continuous wires with vertical steps that maintain the discrepant occlusal planes are preferred over traditional continuous archwire mechanics because light continuous archwires (nickel-titanium [Ni-Ti]) will generally lead to unwanted extrusion of the maxillary anterior teeth, posterior teeth, or both. Typically, the maxillary arch is segmented either between the lateral incisor and the canine or between the canine and the first premolar depending on the point at which the occlusal planes diverge (Fig. 23-11). One advantage that a four-tooth incisor segment has over a six-tooth anterior segment is that the former can be set deeper surgically without the presence of the maxillary canine and there is a natural root divergence between the maxillary canine and the lateral incisor. To augment this root divergence, a segmental root spring can be placed from the molar to the canine to obtain the necessary root divergence for the osteotomy (Fig. 23-12). For segmental surgery, it is much less important to have space between the crowns than between the roots.49 A continuous arch technique to obtain root divergence involves placing a centered V-bend with the apex of the “V” pointing apically, creating equal and opposite moments. One common mistake with continuous arch mechanotherapy is placing an open coil spring to create an osteotomy space. The open coil spring is effective in opening space at the level of the crown but will bring the roots of the adjacent teeth into closer proximity and increase rather than decrease the risk of damage during the interdental osteotomy, as seen in Figure 23-13.
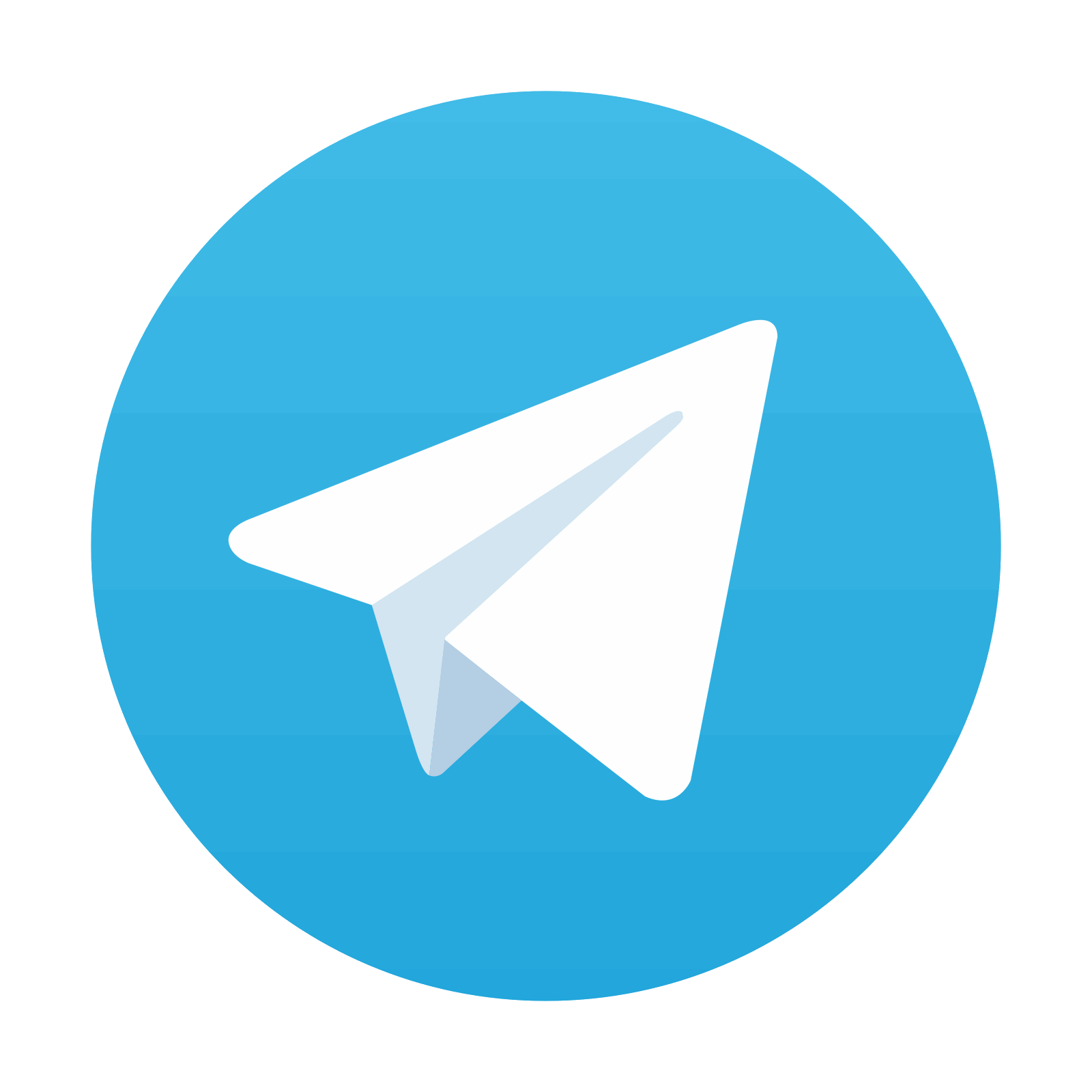
Stay updated, free dental videos. Join our Telegram channel

VIDEdental - Online dental courses
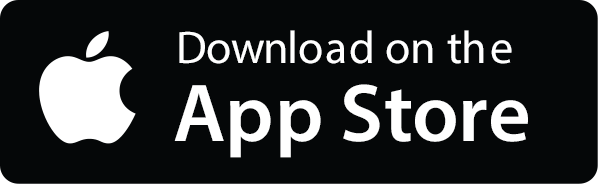
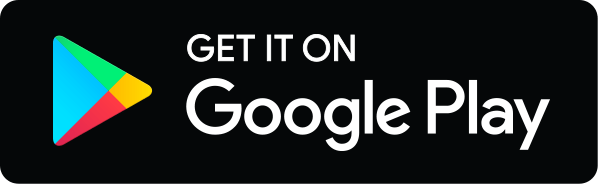
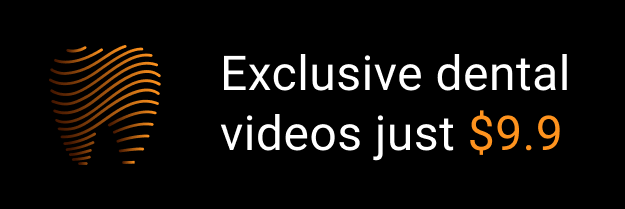