23
3D Imaging in Diagnosis and Treatment Planning of Craniofacial Anomalies
Introduction
The goals of diagnosis and orthodontic treatment planning for patients with craniofacial anomalies (CFA) are no different from those for any patient. These objectives are to establish (1) an ideal, stable occlusion, (2) an esthetically pleasing smile, and (3) ideal facial proportions. What differs between craniofacial and non-craniofacial patients is the initial severity of the discrepancy in CFA patients from these ideals and the magnitude of change required to achieve relatively normal anatomy and relationships. Because of the magnitude of correction required in these patients, the maxim that orthodontics is a 6-millimeter profession lays bare the challenge faced by orthodontists in addressing skeletal and dental discrepancies that substantially exceed this magnitude often in all three planes of space. For this reason, orthodontists and surgeons who treat CFA patients readily adopt technological advances that improve the quality of care they can provide their patients. It is not surprising that these technologies subsequently find their way into everyday practice to the benefit of all orthodontic patients. Three-dimensional (3D) radiographic imaging is one of these technologies, of which cone beam computed tomography (CBCT) is used most widely in orthodontics.
CBCT provides 3D imaging that greatly improves the diagnosis, treatment planning, and assessment of treatment progress and outcomes for all orthodontic patients (Kapila et al., 2011). Moreover, CBCT imaging joins the rapidly expanding armamentaria of digital photography, radiography, and dental cast imaging that are replacing traditional film-based photographs and cephalometric, panoramic and intraoral radiographs and plaster models.
Clearly, there are many advantages to digital records. They can be viewed immediately to ensure they are taken properly and provide the needed information, and can be retaken immediately if needed. Backup copies can be made for storage, and the images can be printed immediately or sent electronically for communication with patients, parents, and other clinicians. In this chapter, we present the current uses of radiographic and non-radiographic 3D imaging in the treatment of patients with common and rare CFAs and syndromes, with a focus on how these technologies are improving diagnosis, treatment planning, and outcomes assessment for individuals with these disorders. We also discuss the importance of integrating 3D imaging data with accurate genetic testing to understand the array of craniofacial anomalies fully and to develop advanced treatments for these patients.
3D Imaging Modalaties for Craniofacial Patients
Multislice Computed Tomography
Multislice computed tomography (MSCT) scans produce excellent details of skeletal morphology, skeletal pathology, patency of sutures, and temporomandibular joint (TMJ) anatomy and ankylosis. While computed tomography (CT) scans are good for imaging mineralized tissues in particular, they also capture soft tissue anatomy. This is valuable for CFA patients who can present with anomalous soft tissue structures, such as soft palate clefting or velopharyngeal insufficiency.
Cone Beam Computed Tomography (CBCT)
CBCT has become a useful tool in diagnosis of and treatment planning for CFA patients. There are several advantages of CBCT imaging over other forms of radiography. The effective radiation dose from CBCT is dependent on the field of view (FOV) and can be as low as 20% of traditional MSCT. Another important advantage of CBCT imaging with CFA patients is the increased anatomic detail and measurement accuracy, which is critical for orthodontic and surgical treatment planning. Digital 3D images can be used to map the distances and directions of dental and skeletal movements precisely that are needed to optimize the treatment outcome (Kapila et al., 2011). In addition, CBCT is invaluable for assessing unerupted tooth position and identifying root resorption caused by unerupted teeth, which are common in CFA patients. As ever-growing numbers of graduate orthodontic programs and private practice orthodontists use CBCT and other forms of 3D imaging for diagnosis and treatment planning, the demand for user-friendly software packages for managing and analyzing Digital Imaging and Communications in Medicine (DICOM) images is increasing. In response to this demand, clinicians now have a wide selection of programs that ease the transition from conventional two-dimensional (2D) to 3D radiography. For both CBCT and MSCT the available software permits multiplanar reconstruction to view the craniofacial skeleton and soft tissues in all three planes of space. Furthermore, 3D models generated from CT scans allow clinicians to appreciate the extent of a patient’s craniofacial anomaly fully and can optimize treatment planning and sequencing among the craniofacial team members.
Magnetic Resonance Imaging (MRI)
MRI produces precise images of intracranial soft tissue structures without radiation. In the craniofacial region, it is used extensively in diagnosing brain and spinal pathology. For example, MRI has been used to study brain morphology in patients with Van der Woude Syndrome and cleft lip and palate (CL/P; Nopoulos et al., 2007, 2010). MRI also is the imaging modality of choice for diagnosing TMJ abnormalities, especially disc displacement (Hamada et al., 2000; see also Chapter 12). Interestingly, MRI use is expanding into prenatal diagnosis (Munch et al., 2009). One limitation of MRI is that it cannot be used on patients with orthodontic appliances in place because the metal in the appliances interferes with the powerful magnetic field needed to generate the images. However, improvements in MRI technology are reducing this limitation (Proffit et al., 2003).
3D Photography
3D photography records soft tissue surfaces of craniofacial structures without any radiation. Although useful, its accuracy still may not be adequate to diagnose craniofacial anomalies (Plooij et al., 2011). However, it can add to the assessment of surgical outcomes such as cleft lip repair and has been used to evaluate variations and phenotypes of syndromes such as trisomy 21. 3D virtual models and shape changes in the noses of CL/P patients after secondary repair have been studied using 3D stereophotogrammetry (van Loon et al., 2010; Sforza et al., 2012). Furthermore, 3D color maps have been used to provide an objective assessment of craniofacial treatment changes (Jayaratne et al., 2010).
3D motion analysis systems historically were developed for gait analysis and now are being adapted for facial motion capture, as represented by the 3dMD Face Dynamic Imaging System (Lubbers et al., 2010). This technology recently has been used for studying facial movements in cleft and non-cleft children (Trotman, 2011).
3D Dental Models
Dental impressions or casts can be laser-scanned or intraoral scans taken to recreate 3D digitized dental models. The 3D images have the advantage of allowing viewing by rotating on a 2D screen, which also allows for accurate measurements. Physical casts can be produced from the digital data through a computer-aided design/computer-aided manufacturing (CAD/CAM) system (Figure 23.1) and also can be merged with CBCT data (see also Chapter 4).
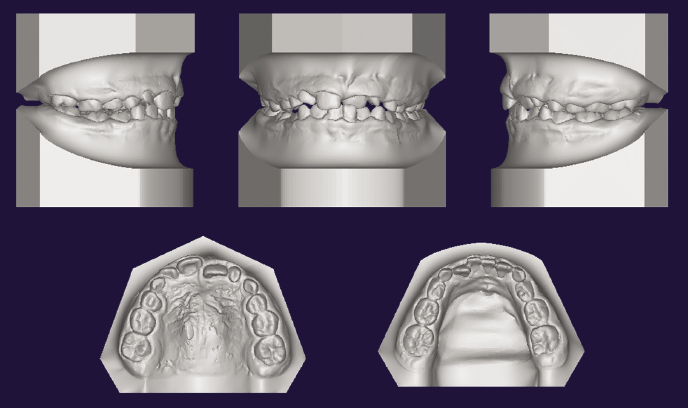
3D Surgical Simulation Models
Treatment planning for CFA patients can be difficult when relying on conventional 2D lateral and frontal cephalograms because 3D structures are superimposed within two dimensions. This complicates estimations of actual size and shape of anomalies and consequently introduces a higher potential for discrepancy between surgical planning and surgical outcome. While surgical simulation models greatly improve surgical outcomes in CFA patients, these models traditionally have been developed from conventional 2D radiographs. 3D surgical simulation models overcome many of the limitations associated with traditional model surgery, most notably the simultaneous access to information on the patient’s skeletal anatomy during the planning process (Swennen et al., 2009; see also Chapters 4, 20, and 21). Concomitant with improved 3D imaging is the rapidly increasing availability of computer software that is designed to mitigate these problems by combining craniofacial imaging modalities to produce a 3D composite model that can be used to simulate surgical procedures. While the imaging techniques used to generate 3D models vary, they typically employ a combination of CT imaging, soft-tissue surface scanning, and digitized tooth molds (see Chapter 4). Simulation software is used to plan individual procedures and assess outcomes. Because this technology allows more predictable surgical treatment planning for complex manipulation of craniofacial structures, it is well suited for use in craniofacial anomalies (Xia et al., 2007). In fact, recent studies indicate that such fusion techniques can predict actual post-operative results more precisely than single treatment planning approaches alone (Gateno et al., 2007; Plooij et al., 2011). These studies indicate that mean linear differences between simulation and surgical results in patients with complex craniofacial deformities were a mere 0.85 mm, with only 1.7° angular discrepancy (Donatsky et al., 1997; Xia et al., 2007). Considering previous data suggesting that differences up to 2.0 mm and 4.0° are insignificant clinically, it is clear that the 3D image analyses and manipulation by the software contribute to the highly predictable results in these cases (Donatsky et al., 1997).
Application of 3D Imaging in Craniofacial Anomalies
Prenatal Diagnosis
Advances in fetal ultrasound and MRI have increased the range and diagnostic accuracy of fetal anomalies. This is valuable because the presence of a structural anomaly in the fetus greatly increases the likelihood of a syndrome or abnormal karyotype (Estroff, 2012). Genetic testing then can be performed to verify the ultrasound or MRI findings.
Ultrasound is used widely to diagnose clefts prenatally and, not surprisingly, 3D ultrasound is superior to 2D ultrasound in detecting cleft lip accurately (Chen et al., 2001). The 3D reverse face view allows a relatively straightforward view of the fetal palate, enabling accurate diagnosis of cleft palate (Campbell et al., 2005). During the past two decades, 3D/4D ultrasound has expanded diagnostic abilities, especially in the prenatal diagnosis of facial clefts, brain anomalies, and spinal defects. In contrast to conventional 2D ultrasound, which allows imaging only of single planes, 3D/4D ultrasound offers a precise demonstration of the normal and abnormal anatomy of the fetus, displaying anatomy in ways not possible with 2D imaging. Digital storage of volumes permits virtual examinations by reloading of volumes and navigating through them at any time (Merz & Abramowicz, 2012). In a study to detect CL/P, prenatal 3D axial ultrasound showed 100% sensitivity and 90% specificity (Baumler et al., 2011).
While ultrasound is excellent for assessing prenatal craniofacial morphology, the views can be limited by shadowing from ossified bone. Thus, MRI is being used increasingly to overcome this problem and to supplement ultrasound. In fact, MRI provides better detection and classification of facial clefts than does ultrasound alone (Mailath-Pokorny et al., 2010). While MRI shows excellent soft tissue contrast with no radiation dose, it is not used routinely in 3D reconstruction due to long scan times, motion artifacts, and poor contrast in anatomically complex regions (Rathnayaka et al., 2013). Motion artifacts particularly are problematic, as they distort 3D reconstruction from the scan data (Rathnayaka et al., 2012).
Research is ongoing in the prenatal detection of craniosynostosis by using 3D ultrasonography. A case report that compared 2D and 3D ultrasound showed that while craniosynostosis was diagnosed with 2D ultrasound and positional molding with 3D ultrasound, the patient indeed had positional molding as confirmed by neonatal radiographs (Krakow et al., 2001). 3D ultrasound enables the visualization of the full length of the cranial suture, which is not possible with conventional 2D ultrasound. Ultrasonography also can be useful in detecting bony abnormalities associated with the syndrome-related causes of craniosynostosis. While a detailed account of prenatal detection of various anomalies is beyond the scope of this chapter, interested readers are referred to several case reports that used both 3D ultrasound and MRI to improve prenatal diagnosis of craniofacial anomalies (Robson & Barnewolt, 2004; Elia et al., 2008; Ginsberg et al., 2011; Luedders et al., 2011; Beegun et al., 2012).
Craniosynostosis and Craniosynostosis Syndromes
Craniosynostosis is characterized by early closure of one or more cranial sutures. Early diagnosis and treatment are essential to release synostosed sutures and allow for skull expansion as the brain grows (Parisi et al., 1989). 3D CT, particularly maximum intensity projection (MIP), permits clinicians to view the degree of cranial suture closure and deformity due to its ability to separate imaging of blood vessels from soft tissue (see “Vascular Malformations” below; Medina, 2000) and is useful for pre-operative planning and assessing post-operative progress (Boyle & Rosenblum, 1997).
The sensitivity of CT scans approaches 100% when combined with physical examination and conventional 2D radiography. Danelson and associates (2009) investigated the benefits of using 3D models in pre-operative planning for craniosynostosis surgery, specifically spring-mediated cranioplasty and cranial vault reconstruction. They showed that the surgical procedure was less invasive using 3D models in planning surgery because less of the skull had to be exposed. Additionally, the force characteristics of the spring could be quantified better. The addition of this pre-surgical planning tool resulted in a less invasive approach and shorter procedure time.
Sagittal synostosis is the most common form of isolated craniosynostosis. It results in a dolicocephalic head shape, ridging over the sagittal suture, frontal bossing, and occipital protrusion due to compensatory growth along the coronal, metopic, and lambdoid sutures. 3D CT demonstrates focal or generalized suture absence with ridging over the sagittal suture (Figure 23.2A).
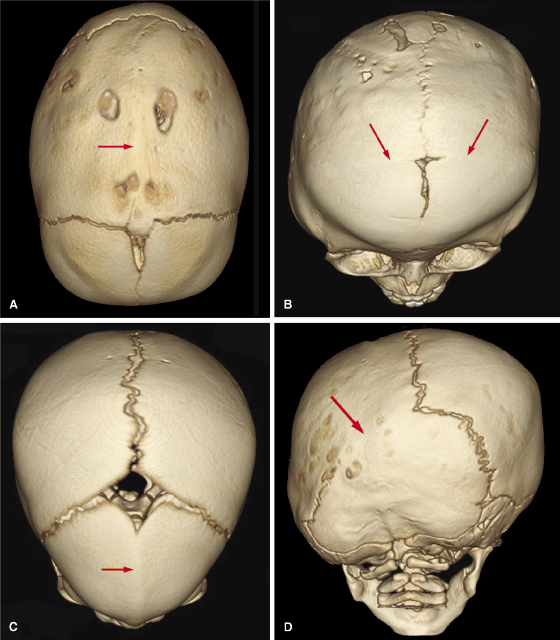
Coronal synostosis, the second most common isolated synostosis (18–29%), results in flattening of the ipsilateral parietal and frontal bones and compensatory bulging of the frontal and temporal regions on the opposite side of the deformity (Figure 23.2B). Metopic synostosis represents 10% of all craniosynostosis. It presents as narrowing of the anterior cranial fossa with a prominent midfrontal ridge resulting in a pointed forehead (trigonocephaly; Figure 23.2C). Lambdoid synostosis (LS) is the least frequent type of craniosynostosis (<5%). It most often is unilateral, resulting in asymmetry, which is referred to as plagiocephaly. It is important to distinguish LS from positional plagiocephaly, which is a flattening of the back of the skull that develops when babies sleep on their backs. Importantly, as opposed to LS, the lambdoidal suture remains patent in positional plagiocephaly. The rates of positional plagiocephaly have increased since the National Institute of Child Health and Development initiated the Back-to-Sleep (BTS) program in 1994 in which parents are encouraged to monitor their baby’s sleep position and to keep the baby on his or her back while asleep to reduce the risk of Sudden Infant Death Syndrome (SIDS; Willinger et al., 1994). A 3D radiograph can distinguish the two conditions based on the patency of the lambdoidal sutures. Both right and left lambdoidal sutures are patent in babies with positional plagiocephaly, whereas one or both of these sutures are fused in LS. Also in LS patients, 3D CT shows a flattening of the skull on the side of suture fusion with an ipsilateral mastoid bulge (Figure 23.2D).
Craniosynostosis syndromes usually are associated with fusion of facial sutures as well as cranial sutures and present with underdevelopment of the midface (Figure 23.3). The midface hypoplasia often compromises vital functions such as respiration and feeding. At some stage of development, surgical correction will become necessary in these patients. Functional, psychological, and esthetic factors play a role in determining the best time for the midface advancement (Vargervik et al., 2012).
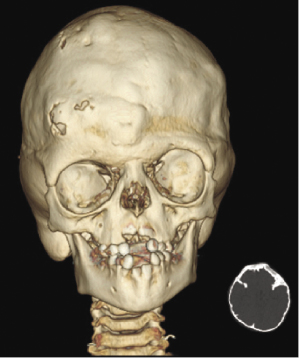
Management of craniosynostosis syndromes requires a team of experienced specialists and extends over the entire growth period from infancy to adulthood. Both the traditional Le Fort III and distraction techniques are effective surgical techniques in correcting the midface hypoplasia (Oberoi et al., 2012). Both CT and CBCT are used to assess malformations and functional impairments, to plan the surgical approach, and to perform long-term follow-up studies after major surgical interventions (Figure 23.4; Meazzini et al., 2012; Ohtani et al., 2012).
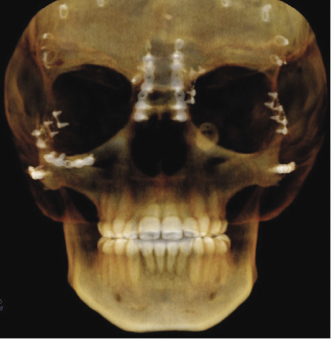
Cleft Lip and Palate (CL/P)
Assessment of the Alveolar Defect and Planning for Alveolar Bone Grafting
With relatively easy access to CBCT, this imaging approach has become standard for our CL/P patients at the start of Phase I orthodontic treatment. Maxillary expansion and protraction, if needed to correct crossbites in preparation for alveolar bone grafting, is a common treatment for individuals with complete CL/P. A successful alveolar bone graft results in continuity of the maxillary arch, facilitates eruption of permanent teeth, provides adequate bone support for erupting teeth, preserves periodontal health of teeth adjacent to the cleft, permits orthodontic tooth alignment, allows placement of osseointegrated implants, and improves alar base symmetry (Bergland et al., 1986; Trindade et al., 2005). Diagnostic imaging is essential before alveolar bone grafting to evaluate the size of the alveolar cleft defect, position and level of bone on adjacent teeth, and presence of supernumerary teeth. After bone grafting, radiographic evaluation helps to determine the outcome of the procedure by evaluating the bone fill of the defect, eruption status of the lateral incisor or canine adjacent to the cleft, and adequacy of bone for placement of endosseous implant (Figure 23.5).
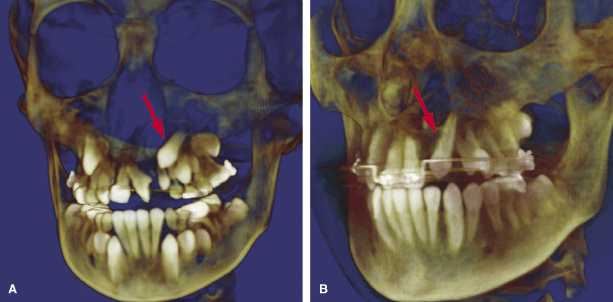
In our recent study to assess the radiographic outcome of alveolar bone grafting in 21 individuals with CL/P using CBCT, the average volume of the pre-operative alveolar cleft defect in unilateral CL/P was 0.61 cm3, and the combined average volume of the right and left alveolar cleft defects in bilateral CL/P was 0.82 cm3 (Oberoi et al., 2009). The average percentage bone fill in both unilateral and bilateral CL/P was 84%. The CBCT also enabled the determination of the adequacy of bone height and width for placement of an implant to replace a missing lateral incisor. In a study to assess accuracy of CBCT measurements on bone height and thickness, buccal bone height and thickness were measured on CBCT scans and compared with direct measurements on human cadavers. The mean differences between CBCT and direct measurements were nearly zero (Timock et al., 2011).
Assessment of Individual Teeth
CBCT is useful in assessing and treatment planning for teeth adjacent to the cleft as illustrated in a case in which the left maxillary permanent central incisor is impacted and erupting horizontally (Figure 23.6A). With the aid of the CBCT scans, exposure and bonding was carried out and the tooth was brought into the arch with appropriate force application (Figure 23.6B). CBCT studies to measure the length of the maxillary central incisor adjacent to the cleft have revealed that the cleft side incisor is shorter than the contralateral one (Zhou et al., 2013). A pilot study on two individuals with van der Woude Syndrome using CBCT showed abnormal morphology of both crown and roots, which was not apparent on the standard panoramic radiograph. Further analyses using both the volumetric and linear measurements of the ratio of crown body to root were indicative of taurodontism in this patient (Figure 23.7; Nawa et al., 2008).
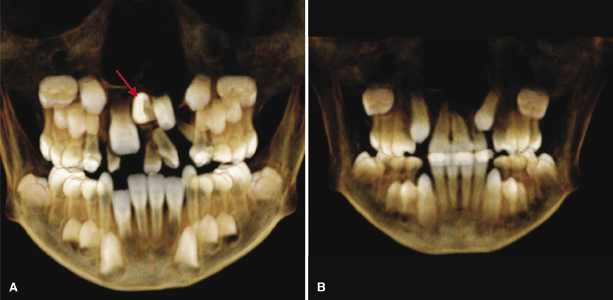
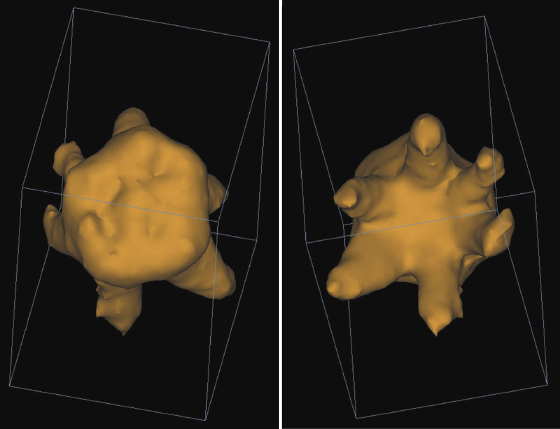
Eruption Status and Eruption Path of the Maxillary Canine in Non-Cleft and Grafted Cleft Side
Several studies have found that the percentage of canines that erupt spontaneously through the bone-grafted site can vary widely, from a low of 27% to a high of 97% (Turvey et al., 1984; da Silva Filho et al., 2000). A recent CBCT study on 21 consecutively treated unilateral CL/P patients showed that 12% of the canines did not erupt spontaneously (Oberoi et al., 2010). Most canines on both the grafted cleft and non-cleft side moved incisally, facially, and mesially. Presence or absence of the lateral incisor did not affect the vertical eruption of the canine. The study showed that alveolar bone grafting after orthodontic expansion and before eruption of the permanent canine crown into the defect allows a normal path of eruption of the canine, predominantly without impaction, thereby enhancing alveolar bone development and facilitating orthodontic management.
Airway Characteristics
Anatomical abnormalities associated with CL/P increase the risk of airway complications. Nasal abnormalities such as septal deviation, nostril atresia, turbinate hypertrophy, and maxillary constriction are frequent findings (Hairfield et al., 1988). These abnormalities are attributed, in part, to the congenital defect itself and partly to the surgeries done to repair the orofacial defect. Collectively, the nasal abnormalities tend to reduce the dimensions of the nasal cavity and overall airway function.
Airway patency has been evaluated by 2D radiographic imaging, such as lateral and postero-anterior cephalometric films, and functional studies such as rhinomanometry and plethysmography (Smahel et al., 1991; Drake et al., 1993; Imamura et al., 2002; Fukushiro & Trindade, 2005; see also Chapter 13). In contrast to 2D methods, MSCT or CBCT provide dimensional and volumetric airway data for accurate assessment of the airway in all three planes—coronal, sagittal, and axial (Figure 23.8). Due to the high radiation dose for standard MSCT scanning, however, CBCT is preferred for repeated longitudinal studies. Studies have shown that 3D imaging using CBCT is a simple and effective method to analyze the airway accurately (Abramson et al., 2009; Iwasaki et al., 2009; Olszewska et al., 2009; Kim et al., 2010; Hatcher, 2012; see also Chapter 13), and a systematic review of the literature indicates that 3D analysis of the upper airway using CBCT can provide accurate and reliable results (Guijarro-Martinez & Swennen, 2011). Nevertheless, important limitations including the impact of respiratory phase, influence of tongue position and mandibular morphology, and 3D CBCT definition of the anatomical boundaries of the upper airway must be taken into account for such an analysis.
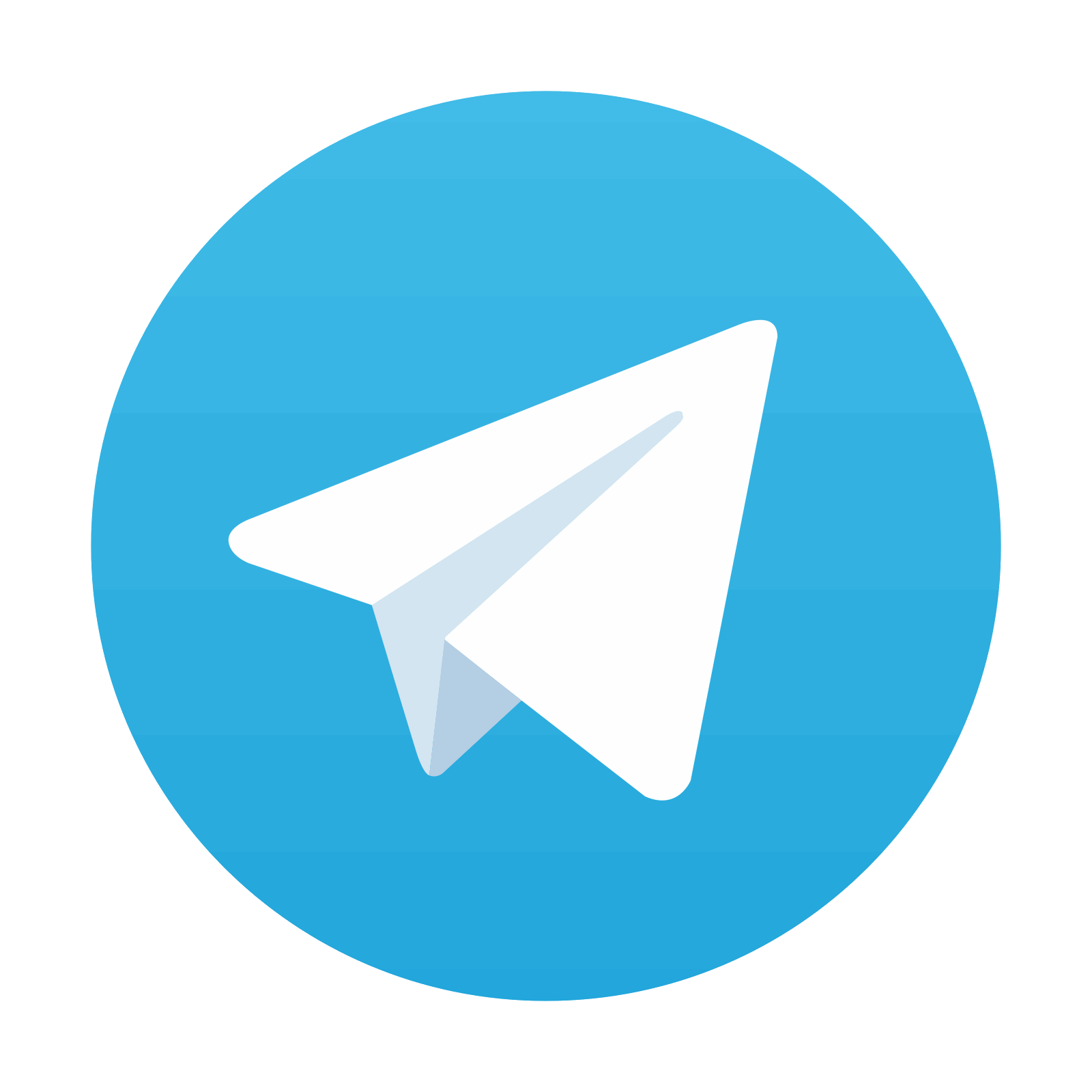