14
Alveolar Boundary Conditions in Orthodontic Diagnosis and Treatment Planning
Introduction
In orthodontics, alveolar boundary conditions are the depth, height, and morphology of alveolar bone relative to tooth root dimensions, angulation, and spatial position (Kapila et al., 2011). Boundary conditions are defined not only by dentoalveolar anatomy prior to treatment, but also by their adaptability during tooth movement and their morphology following the final positioning of teeth. As such, the pre-treatment status of boundary conditions and their potential adaptation may dictate the limits of planned tooth movement, as well as the final desired spatial position and angulation of the tooth. Alveolar boundary conditions are not static; in fact, their remarkable dynamism can be both good and bad. Without remodeling of alveolar boundary conditions, orthodontic tooth movement would not be possible. Conversely, applying inappropriate orthodontic forces to a tooth can affect alveolar boundary conditions adversely, resulting in dehiscences and fenestrations. Maximizing alveolar boundary condition remodeling and minimizing unwanted sequelae for each patient is a desired orthodontic goal. Although not established yet, it is possible that cone beam computed tomography (CBCT) may offer a useful tool to meet this objective.
Alveolar boundary conditions impact diagnosis and treatment of various malocclusions, such as mild to moderately crowded nonextraction cases, dentoalveolar compensations due to transverse (Figure 14.1A,B) or sagittal skeletal discrepancies, situations with severely compromised bone (Figure 14.1C,D), bimaxillary protrusion (Figure 14.2), and inadequate or missing bone such as in cleft lip and palate (CL/P). Moreover, bone boundary conditions can affect treatment decisions and diminish the ability to provide ideal treatment in situations where neighboring structures, such as sinuses or transposed teeth, are likely to interfere with the planned tooth movement (Figure 14.3).
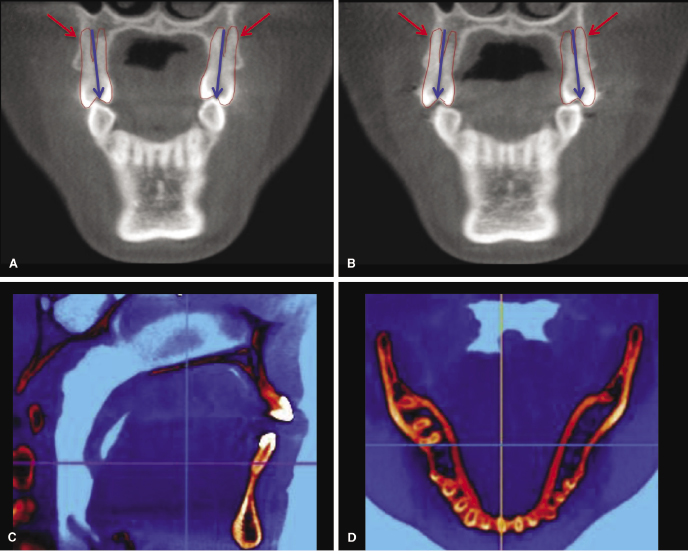
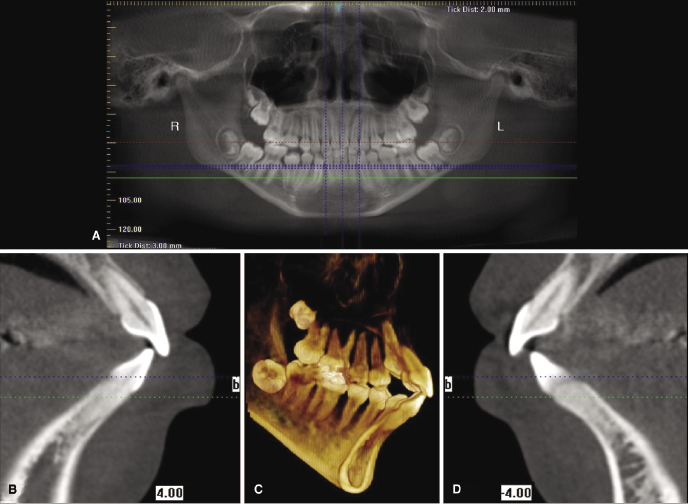
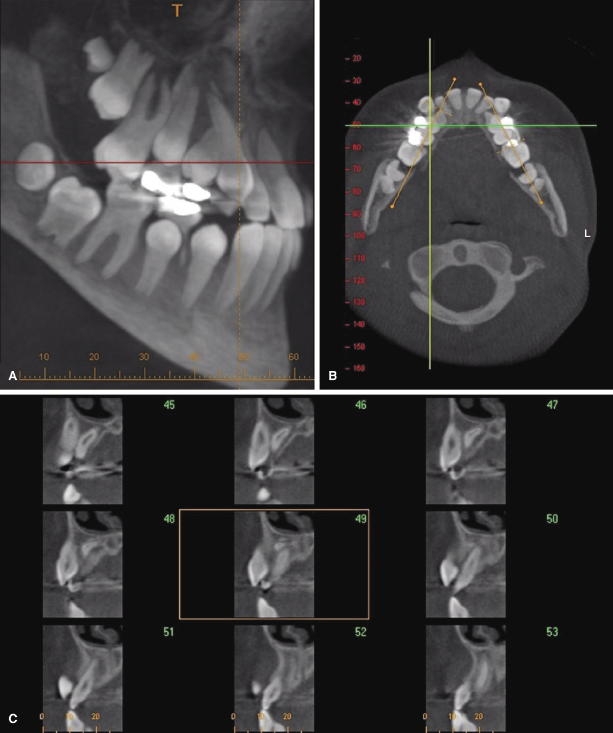
Prior to the introduction of CBCT, little was known about how alveolar boundary conditions restricted or responded to orthodontic treatment beyond the appearance of gingival recession or bony dehiscences. Because conventional two-dimensional (2D) radiographs do not image the dentoalveolar complex in all three planes of space, researchers often have drawn conflicting interpretations of morphological changes in the teeth and their supporting structures following orthodontic treatment. This chapter reviews the small and sometimes conflicting literature on alveolar boundary conditions with the goal of providing information on its implications to orthodontic diagnosis, treatment planning, and treatment outcomes. More specifically, we have delineated the use of CBCT to classify patients by their alveolar boundary condition biotype and to identify patient- and treatment-specific risk factors that may compromise alveolar boundary conditions. Also discussed are advances in our understanding of extreme variations in morphologic boundary conditions most commonly associated with developmental anomalies as revealed through CBCT imaging. In addition, we describe an approach to analyzing and biotyping alveolar boundary conditions using CBCT images.
Alveolar Boundary Condition Biotype: A Potential New Diagnostic Criteria
Orthodontic forces move teeth within the alveolar bone of the maxilla and mandible. Failure to stay within the alveolar bone has significant and often irreversible negative sequelae, such as dehiscences and fenestrations (Wennstrom, 1996). Assessing a patient’s periodontal status before, during, and after orthodontic treatment generally is considered standard of care (Machen, 1989). While bleeding on probing and pocket depths are reliable indicators of active periodontal inflammation, they do not indicate if the alveolar bone is normal. There now is abundant evidence that bony dehiscences and fenestrations may be present in individuals who otherwise have unremarkable gingiva and who never had orthodontic treatment (Nauert & Berg, 1999; Mostafa et al., 2009; Evangelista et al., 2010; Lund, 2011; Ehnos et al., 2012; Nahm et al., 2012; Patcas et al., 2012; Yagci et al., 2012; Figure 14.4). Failure to diagnose compromised alveolar bone prior to treatment and to factor this into the treatment plan likely will lead to worsening of the problem if the involved teeth are moved orthodontically. Even if tooth movement does not necessarily position the teeth into more compromised bone sites, some bone loss can be expected during routine orthodontic treatment (Johnson, 2011; Welmerink, 2012). Thus, the more data we obtain on pre-treatment alveolar boundary conditions and on the magnitude of expected change in bone support during orthodontic tooth movement, the better our orthodontic diagnosis and treatment planning for our patients will be.
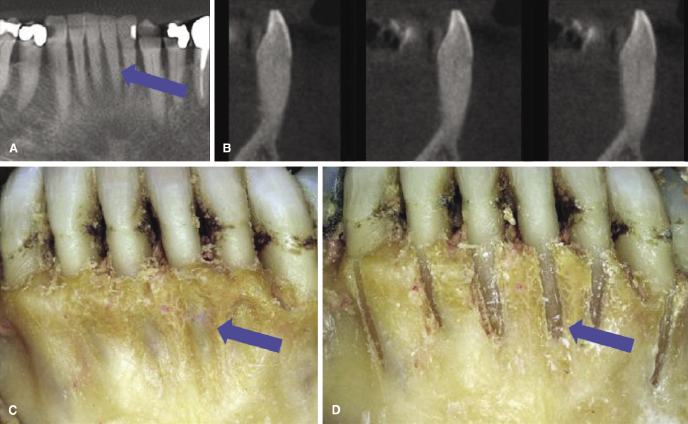
Studies demonstrating pre-existing dehiscences or fenestrations also demonstrate a considerable range of cortical bone thickness overlying tooth roots and total alveolar bone width in subjects with intact alveolar bone. This suggests that patients could be categorized into thin, medium, or thick alveolar bone biotypes based on CBCT images taken as part of the pre-treatment database, as discussed later (Figure 14.5). These data could improve orthodontic diagnosis and treatment planning greatly, especially in borderline non-extraction or camouflage patients where the treatment plan may require moving teeth close to the alveolar boundary or, in some cases, beyond the existing alveolar boundary with the hope or expectation that it will remodel to keep up with the repositioned teeth.
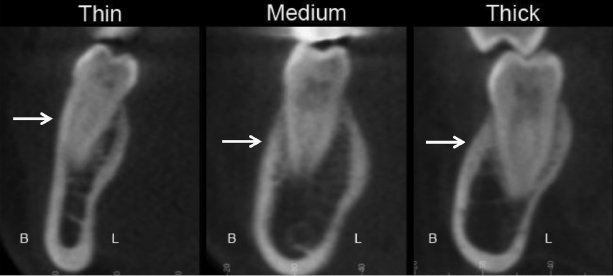
Patients with moderate crowding may be candidates for non-extraction treatment if sufficient arch length can be achieved by expansion, incisor proclination/protrusion, or molar distalization, provided that the boundary conditions will undergo the desired change concurrently. Estimates of how much arch length can be gained in non-extraction treatment vary. For every 1 mm of incisor proclination/protrusion, 2 mm of arch length can be gained. Canine expansion results in a 1:1 increase in arch length. The expected increase in arch length from the increases in both the incisor position and canine expansion is considered to be an overestimation of the actual change (Noroozi et al., 2002 and references therein). Estimates also vary regarding rapid palatal expansion with reported increases in arch length of 0.5–0.7 times the amount of posterior expansion (Adkins et al., 1990; Akkaya et al., 1998; Claro et al., 2006). While dental alignment certainly is achievable in crowded cases, as has been demonstrated over the past more than a century of orthodontics, the ability and limits of bone adaptability to follow suit has not been documented well. Furthermore, although conventional 2D radiograph studies are able to provide some insights into the ability of the alveolar bone to adapt or not adapt around incisors in the sagittal plane when using lateral cephalograms (Figure 14.6), they are unable to depict changes in periodontal status in most other dimensions and cases; this is important particularly for the borderline non-extraction or camouflage cases (Demir et al., 2005; Isik et al., 2005; Xu et al., 2006; Kinzinger et al., 2009; Troy et al., 2009; Burns et al., 2010; Germec-Cakan et al., 2010; Turkoz & Iscan, 2011). Given the lack of consensus on the arch length changes in borderline non-extraction cases, it should not be a surprise that these cases generate significantly different treatment approaches among orthodontists (Baelum et al., 2012). CBCT may offer additional relevant data for evidence-based treatment planning and better predict treatment outcomes for these patients.
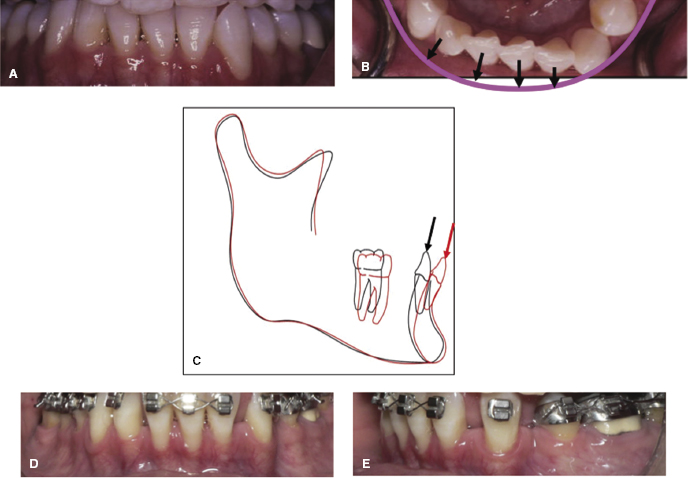
Although, in general, a thin gingival biotype is likely to be an indicator of a similarly thin underlying bone biotype (Fu et al., 2010; Cook et al., 2011), the reverse, namely, that a thick gingival biotype is associated with a thick bone phenotype, may not always hold true. While little research has been performed to determine whether the latter is indeed the case, individuals with a thick and dense soft tissue sometimes can present with fenestrations in the underlying bone. This is demonstrated in a patient presenting with a bimaxillary anterior openbite associated with a long-standing thumb-sucking habit (Figure 14.7). The treatment involved extractions with corticotomies to facilitate tooth movement and bite closure. On reflecting the flap for the corticotomy, substantially compromised periodontal boundary conditions with fenestrations on the lateral incisors and canines were noted. Due to the thick gingival biotype, it was not possible to detect any root prominences clinically. It is plausible that the poor bone support around the roots of several teeth in this patient may have resulted from the high forces generated from the thumb-sucking habit. Thus, long-standing finger habits may be another indicator for CBCT imaging to determine the status of bone boundary conditions and for appropriate planning the treatment in these patients.
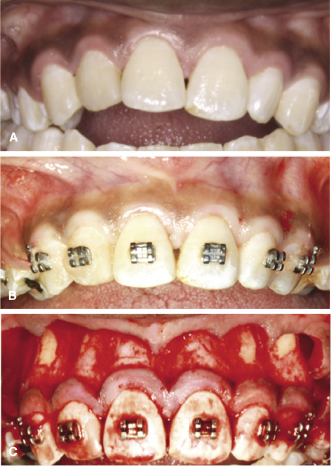
Technical Considerations for Using CBCT to Assess Alveolar Boundary Conditions
It is important to point out that there are several technical variables that alter the sensitivity and specificity of CBCT for diagnosing alveolar boundary condition biotypes (Leung et al., 2010; Ising et al., 2012; Patcas et al., 2012). While details of these variables are beyond the scope of this chapter and are discussed elsewhere (see Chapter 2), it is sufficient to say that these affect all CBCT images and include machine-specific settings such as milliampere (mA), peak kilovoltage (kVp), exposure time, and sensor size, and patient-specific variables including age, bone density, and periodontal biotype (Molen, 2010). Of these variables, sensor size is the most logical candidate for manipulation if the objective of imaging is to obtain accurate measurements of alveolar boundary conditions. Sensor size influences the image resolution; thus, smaller sensors are indicated for alveolar bone imaging, yet clinicians should be aware that the highest available resolution images currently overestimate dehiscences and fenestrations (Patcas et al., 2012). As sensor and software technologies improve, limited field of view (FOV) CBCTs possibly could provide adequate diagnostic information for this purpose and may be indicated for patients who are considered at risk for developing alveolar defects during orthodontic treatment.
Risk Factors for Compromising Alveolar Boundary Conditions
In general, risk factors for negative sequelae in orthodontics are categorized as patient- or treatment-specific. Potential risk factors that may compromise alveolar boundary conditions are no exception to this rule. Despite the limited research in this area, especially for CBCT-based studies, initial studies are providing significant insights into patient- and treatment-specific changes in alveolar boundary conditions following orthodontic treatment.
Patient-Specific Risk Factors for Compromised Alveolar Boundary Conditions
Facial pattern and skeletal malocclusion have been the focus of early CBCT studies as potential risk factors for compromised alveolar boundary conditions. Several studies show that retraction or proclination of incisors in patients presenting with specific malocclusions or pre-existing alveolar boundary conditions are associated with adverse bone support at the end of treatment. Thus, a spiral CT-based study supports the conclusion that bimaxillary protrusive patients undergoing extraction treatment are at risk for significant alveolar bone loss by demonstrating that significant crestal and mid-root alveolar lingual bone loss occurs routinely around maxillary and mandibular incisors of these patients following incisor retraction (Sarikaya et al., 2002). The maxillary lateral incisors lost 87% and 50% of their crestal and mid-root lingual alveolar bone, respectively. Also, maxillary central incisors lost 61% and 42% of their crestal and midroot alveolar lingual bone, respectively. Likewise, the mandibular lateral incisors lost 70% and 37%, and mandibular central incisors lost 94% and 44% of their crestal and mid-root lingual alveolar bone, respectively. Moreover, of the 19 subjects in this study, eight had at least one palatal dehiscence in the maxilla and 11 had at least one lingual dehiscence in the mandible after treatment. Patients with bialveolar protrusion also were shown to start treatment with less than 1 mm of buccal bone covering over as much as 80% of the root length (Nahm et al., 2012; Figure 14.7). Taken together, these studies strongly suggest that bimaxillary protrusive patients may be at risk for dehiscences following incisor retraction. Thus, if the treatment plan entails decreasing the bimaxillary protrusion through incisor retraction, pre-treatment CBCT images may be warranted to determine if sufficient palatal or lingual alveolar bone width is available to retract the incisors fully without compromising the alveolar boundary conditions (Figure 14.2).
Just as with bialveolar protrusive cases where bone boundary conditions may fail to adapt to the substantial orthodontic retraction of incisors, increases in incisal proclination are known to be a risk factor for development of dehiscence (Fuhrmann, 1996). Recession caused by proclination of the incisors has been hypothesized to be worse in patients with thin pre-treatment symphysis width (Wehrbein et al., 1996; Artun & Grobety, 2001; Figure 14.8). These findings suggest that there may be limits to adaptability of bone on incisors undergoing proclination, particularly in patients with specific pre-treatment bone biotypes, and that it may be best to maintain incisal angulation during treatment, especially when thin bone is present.
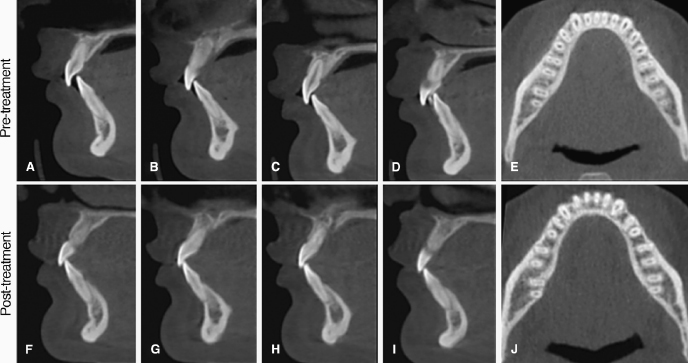
Other possible patient-specific variables that might be related to presence of dehiscences and fenestrations are angle and skeletal classifications of the malocclusion. Two CBCT studies provide conflicting data regarding the effect of skeletal classification on the presence of fenestrations, but not dehiscences in patients without bidentoalveolar protrusion (Evangelista et al., 2010; Yagci et al., 2012). Both studies confirm that pre-treatment dehiscences are present across the spectrum of Class I, II, and III patients. Only the study by Evangelista and colleagues (2010) found that Class I patients have significantly more dehiscences compared with Class II division 1 patients. In this study, 51.1% of all teeth (n = 4319) had dehiscences, most of which are on the mandibular teeth (57.4%). Interestingly, dehiscences are equally likely on the buccal and lingual aspects of the mandibular incisors in Class I and Class II patients, but buccal dehiscences alone are present in 69.4% of the remaining teeth. In contrast, Yagci and colleagues (2012) found that dehiscences are common across all angle classifications, although they are more prevalent in Class II patients. In each Angle Class, dehiscences are found most often on the mandibular incisors (27.0%), and, unlike the findings by Evangelista and coworkers, buccal dehiscences are significantly more common than lingual dehiscences for all maxillary (86% buccal vs. 14% lingual) and mandibular (98.5% buccal vs. 1.54% lingual) teeth (n = 3,976).
Evangelista and associates (2010) and Yagci and coworkers (2012) also assessed fenestrations and noted that pre-treatment fenestrations are found in each angle class, but there were differences between the two studies in the locations of the fenestrations. Yagci’s group found more fenestrations on mandibular teeth in Class II (18.3%) compared with Class I (1.73%) or Class III (2.38%) malocclusions. In contrast, Evangelista’s group found no difference in fenestrations between Class I and Class II patients. Moreover, they found a greater prevalence of maxillary fenestrations (68.4%) compared with mandibular fenestrations (31.6%). There are no obvious reasons for the variations in the data collected in these studies, which points to the need for continued research on the presence of dehiscences and fenestrations in untreated Class I, II, and III patients.
Vertical facial patterning also may contribute to the occurrence of dehiscences, but not fenestrations. Hyper-, normo-, and hypo-divergent patients all have dehiscences, especially on the mandibular incisors. However, hypo-divergent patients have significantly fewer dehiscences than hyper- or normo-divergent patients (Ehnos et al., 2012). This study also found that fenestrations are rare, but, when present, they are most common on maxillary first premolars, which confirms the findings of Yagci and colleagues (2012).
While there currently are only a small num/>
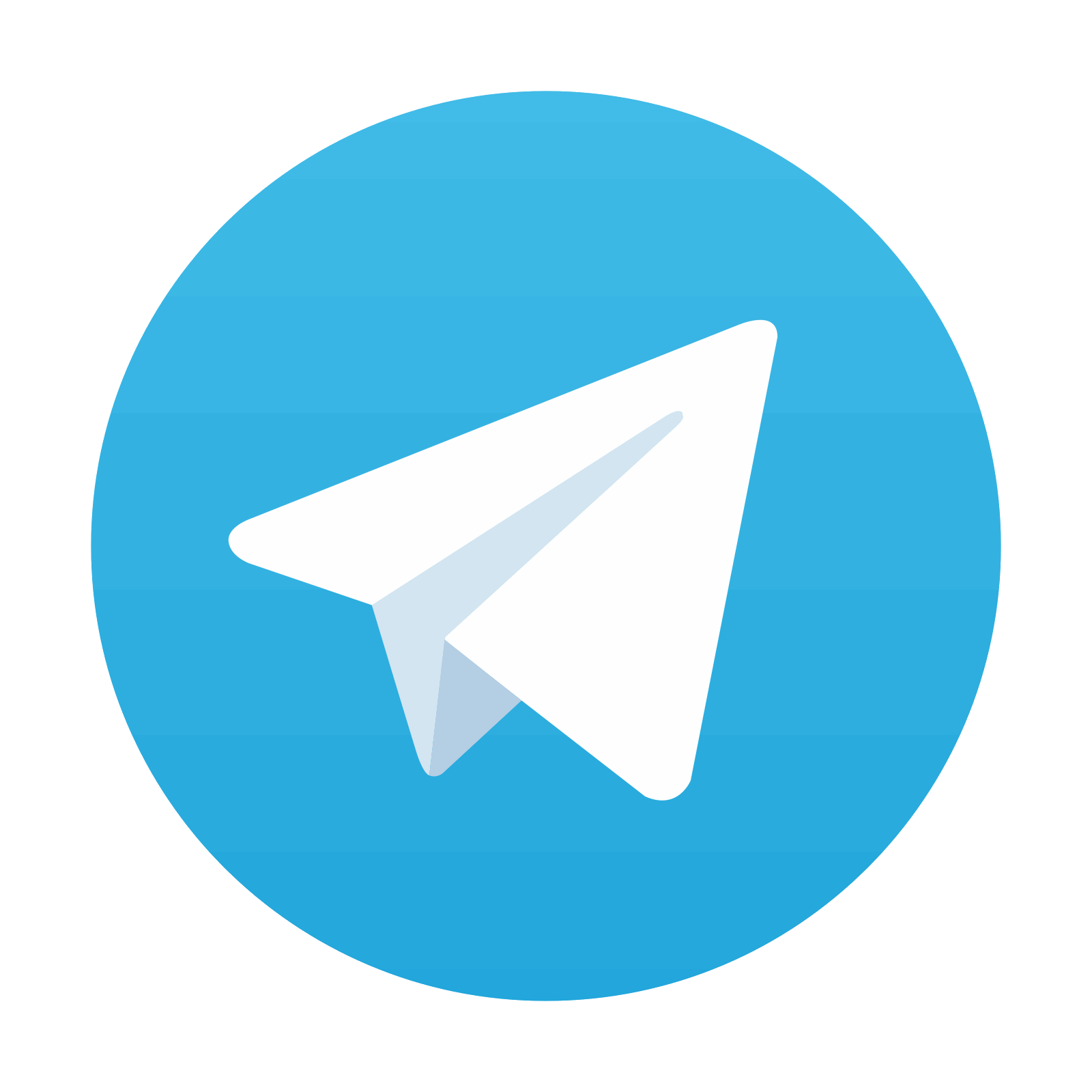
Stay updated, free dental videos. Join our Telegram channel

VIDEdental - Online dental courses
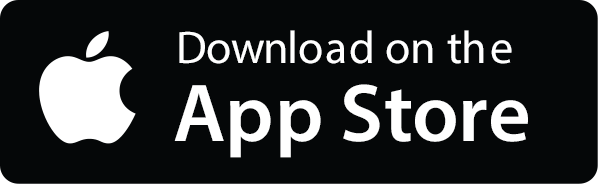
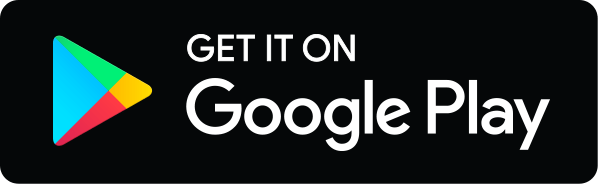