Emerging Technologies
Emerging applications of dental biomaterials are primarily focused on the prevention and treatment of caries, periodontal disease, and oral cancer. The materials being used currently are useful for treating and detecting such diseases, but they need further improvement. Caries prevention treatments have reduced the need for initial restorations, replacement restorations, and removable dentures. Minimally invasive dentistry concepts have led to sealing defective or leaking margins of restorations with sealing resins rather than performing restoration replacement procedures that can destroy healthy adjacent tissue (Mertz-Fairhurst et al., 1998).
Over the past 30 years technology has advanced tremendously and has benefited dental materials science in a variety of ways, including laser applications, imaging technologies, composites technology, “smarter” and stronger ceramics, and minimally invasive dental procedures (Garcia and Tabak, 2009). CAD-CAM technology has reduced the need for impression materials and has also reduced the time required for fabricating and delivering both fixed and removable devices (Strub et al., 2006). At the time of this writing it has been almost 12 years since Feitas (2000) predicted that the first micron-sized dental nanorobots would be constructed within 10 to 20 years. Such advances are very futuristic and may still not be realized for many decades. However, some of the other technologies foreseen at that time have made substantial progress, including those involving the use of nanomaterials, biomimetics, tissue engineering, biotechnology, and regenerative dentistry. This chapter reviews technologies that have demonstrated their proof-of-concept potential and have either been recently introduced but not yet clinically proven for the long-term or are not far beyond the immediate time horizon. Several new materials that are certain to expand in use are also included.
In the following sections and subsections several technologies are discussed under various headings (Nanotechnology, Resin Restorative Materials, Tissue Engineering, Regenerative Dentistry and so on). However, substantial overlap exists among these approaches to various oral health–related problems; therefore any subcategorization is to a greater or lesser extent arbitrary. In the following discussions, the reader will often find that one subject area is necessarily intertwined with another. For example, a major thrust in developing restorative resin composites is to utilize ever smaller reinforcing filler particles. As explained in Chapter 13, this has led to the development of nanoparticle fillers, which overlaps with the field of nanotechnology. In another example, one of the major dental applications of regenerative dentistry uses stem cell biology to promote periodontal wound healing and tissue regeneration, which are also objectives of both biological delivery systems and tissue engineering. So, although tissue engineering does not always involve biological delivery systems or vice versa, it often does and is therefore, categorized separately.
Shown in Figure 21-1 is a flowchart of basic, translational, and clinical research links representing stages in the development and evaluation of emerging technologies and products. The farther to the right of the chart a technology or material is seen, the higher the probability that it will have a desirable clinical outcome.
Biomaterials
Nanotechnology
The introduction of microfill restoratives in the early 1970s ushered in the era of dental nanotechnology. As discussed in Chapter 13, microfilled composites contain silicon dioxide (SiO2) reinforcing particles about 40 nm in diameter that are dispersed in prepolymerized resins that have been ground to form so-called organic filler particles in the micron size range. Current microhybrid and nanofilled composites contain filler particles range from about 20 to 600 nm. This use of nanoparticle technology allows the formulation of dental materials with high translucency, excellent initial polish, and retention of gloss while maintaining mechanical properties and wear resistance equivalent to those of current clinically proven hybrid composites. Several 2- to 3-year clinical trials have investigated the nanohybrids performance in a variety of oral restorations and found that the nanohybrids show great promise for extending survival beyond the current 5 to 10 years. Currently, a variety of approaches designed to overcome the deficiencies of nanoparticle composites are in progress. None of these is likely to result in a breakthrough that will lead to a universal esthetic composite that can rival the durability of amalgam. However, there is a high likelihood that many will contribute incremental advantages that, when combined and optimized, will result in such a material by the next decade.
Other recent applications of nanotechnology in dentistry have focused on the delivery of molecules that promote hard tissue remineralization by noninvasive techniques. In this context, the most promising technology for the nanorestoration of tooth structure is biomimetics, the study and use of processes that mimic those that occur in nature, particularly those that involve self-assembly of components to form, replace, or repair oral tissues (Saunders, 2009). These concepts are discussed further later in this chapter.
For dental biomaterials, nanoparticulates have been shown to strongly influence the host response at both cellular and tissue levels, making their use particularly attractive for modifying dental implant surfaces. Electrophoretic, sol-gel and pulsed laser deposition, sputter coating, and ion beam–assisted deposition, among others, are approaches that have been utilized to develop nanotextured thin-film biocompatible coatings for implant surfaces. These technologies reduce the thickness and particle size of the coating layer and thereby increase its specific surface area and reactivity, thus improving the interaction with the surrounding living tissue. See, for example, Bayne (2005) and (Subramani and Ahmed, 2012).
These technologies are at an advanced stage and their use to develop nanosized preparations of various components in dental materials and improve both performance properties and durability in the near future is quite likely. However, it should be noted that nanosized particles and surface features with very high surface area–to-volume ratios are usually different in their bioactivity, solubility, and antimicrobial effects compared with larger particles. Thus, these changes in properties cannot be extrapolated by an inverse linear analysis of particle size, but must be determined through in vitro and in vivo testing of the nanomaterials. Potentially important nanoparticles will range from metals, such as silver, to ceramic powders, such as titanium dioxide. For example, in situ–generated silver nanoparticles have been reported to be highly effective in restorative resins, bonding resins, and prosthetic resins for inhibiting a variety of biofilm-forming bacteria while not interfering with manipulation, curing, mechanical properties, or other performance properties (see Fan et al., 2011, and Oei et al., 2012).
Resin Restorative Materials
Longevity and survival studies have shown that dental resin composites available during the past 10 years or so have an average replacement time of 5.7 years. The failures were mainly caused by three primary factors: (1) surface loss due to two- and three-body wear; (2) marginal deficiencies due to breakdown and/or gap formation and (3) secondary marginal caries (secondary caries). Whereas the relatively recent innovations discussed earlier (“Innovations in Dental Composites” in Chapter 13) have likely increased the service life of currently marketed resin composites, there is still an incomplete understanding of all factors that lead to failures. For example, there remains significant uncertainty on the interactions among several variables including bacterial biofilms, such as dental plaque and the mechanisms of wear, fatigue, fracture, and secondary caries. Current ongoing research is making progress to gain better insights into how interactions among physical and oral environmental factors and the composition of composites initiate and influence failure mechanisms (Petrovic et al., 2010). Future research should lead to developments that further extend durability and survivability, possibly rivaling that of amalgam.
G-aenial Universal Flo is based on 4,8-di(methacryloxy methylene)-tricyclodecane (TCDDMA), a bulky, space-filling dimethacrylate monomer (see Figure 13-22 in Chapter 13). TCDDMA has a tricyclodecane three-ring central group that prevents monomers from aligning and thus offsets polymerization shrinkage. This “steric hindrance” effect slows the rate of polymerization and lengthens the time needed for the curing reaction to reach the point of solidification. This facilitates the ability of adjacent polymer chain segments to slip among themselves and relax stresses that develop before the resin paste solidifies.
These two highly flowable composite products also contain a reinforcement filler loading on a par with highly loaded, nonflowable, even “packable” hybrids such as those described in Chapter 13. This high loading, together with low curing stress and a high level and depth of cure, account in large part for the expectation of their high durability and long service life. At this time the means whereby this combination of high filler loading and a flowable working consistency are achieved have not been disclosed. However, given the intensity of research and development, other products of this type can be expected and, with further improvements, significant progress toward the goal of ensuring reliably placed restorative resins with the durability and forgiveness of amalgam combined with the esthetics of porcelain can be expected in the near future.
Remineralizing Agents and Materials
The remineralization of tooth surfaces with incipient lesions, prevention of secondary caries, and durable bonding to etched dentin are key areas of research for future dental materials (Donly et al., 1994 and Lippert et al., 2004) because of their great public health importance in preventing sustained enamel demineralization and prolonging the service life of composite restorations without repair or replacement. In the future, nanoparticles of hydroxyapatite may be helpful for remineralization because they are on the same crystalline scale as naturally occurring hydroxyapatite crystals in teeth. A promising example of this approach was reported by (Li et al., 2008) who found that 20-nm nanohydroxyapatite particles provided an anticaries repair effect while larger hydroxyapatite particles did not.
New remineralizing dentin bonding agents should ensure wetting and flow and preferably completely fill the demineralized zone created by etching prior to or simultaneous with bonding to the restorative material. For instance, new MTA-type* products have been tested in the lab with polyacrylic acid and sodium tripolyphosphate as a dentin-bonding layer. When it was applied under a restorative material, the combined material infiltrated the demineralized collagen fibrils with precursors that restored the calcium phosphate at the interfaces of the dentin and the restorative material. These and other remineralizing materials are needed to move forward from the laboratory to clinical trials of new dental adhesives for composites. Incorporation of some of these technologies into primers and dentin bonding agents are expected to both inhibit caries activity and increase the service life of composite restorations.
Low concentrations of fluoride are known to promote enamel and dentin remineralization (Gelhard and Arends, 1985). However, after treatments with topical fluoride gels, rinses, or dentifrices, salivary fluoride concentrations decrease exponentially to low concentrations within a few hours.
Stimulus-Responsive “smart” Materials
“Smart” materials are designed for interaction with external stimuli such as light, temperature change, stress, moisture, pH, or electric/magnetic fields (McCabe et al., 2009). Currently, these dental materials include (1) zirconia ceramics that transform from a tetragonal to monoclinic crystal form when tensile stress is induced at crack tips, leading to an increase in crystal volume and compressive stress that tends to prevent propagation of the cracks; (2) composites designed for initiation of curing by irradiation with particular wavelengths of blue light or composite cements that change color when irradiated (for use in orthodontics); (3) glass ionomer cement that when desicccated, weakens the cement to make it easier to remove orthodontic bands; and (4) glass ionomer restoratives that increase the release of fluoride when the pH in plaque fluid decreases (ten Cate et al., 1995).Nickel-titanium wires that soften when chilled below body temperature for bending are “smart” and very useful for threading together orthodontic brackets at the earliest treatment stages with the most misaligned teeth. Future smart materials may include cements that can be triggered to soften and allow debonding by a temperature change or irradiation process. Cements such as this would be very attractive for use with temporary crowns, orthodontics, and some implant restorations.
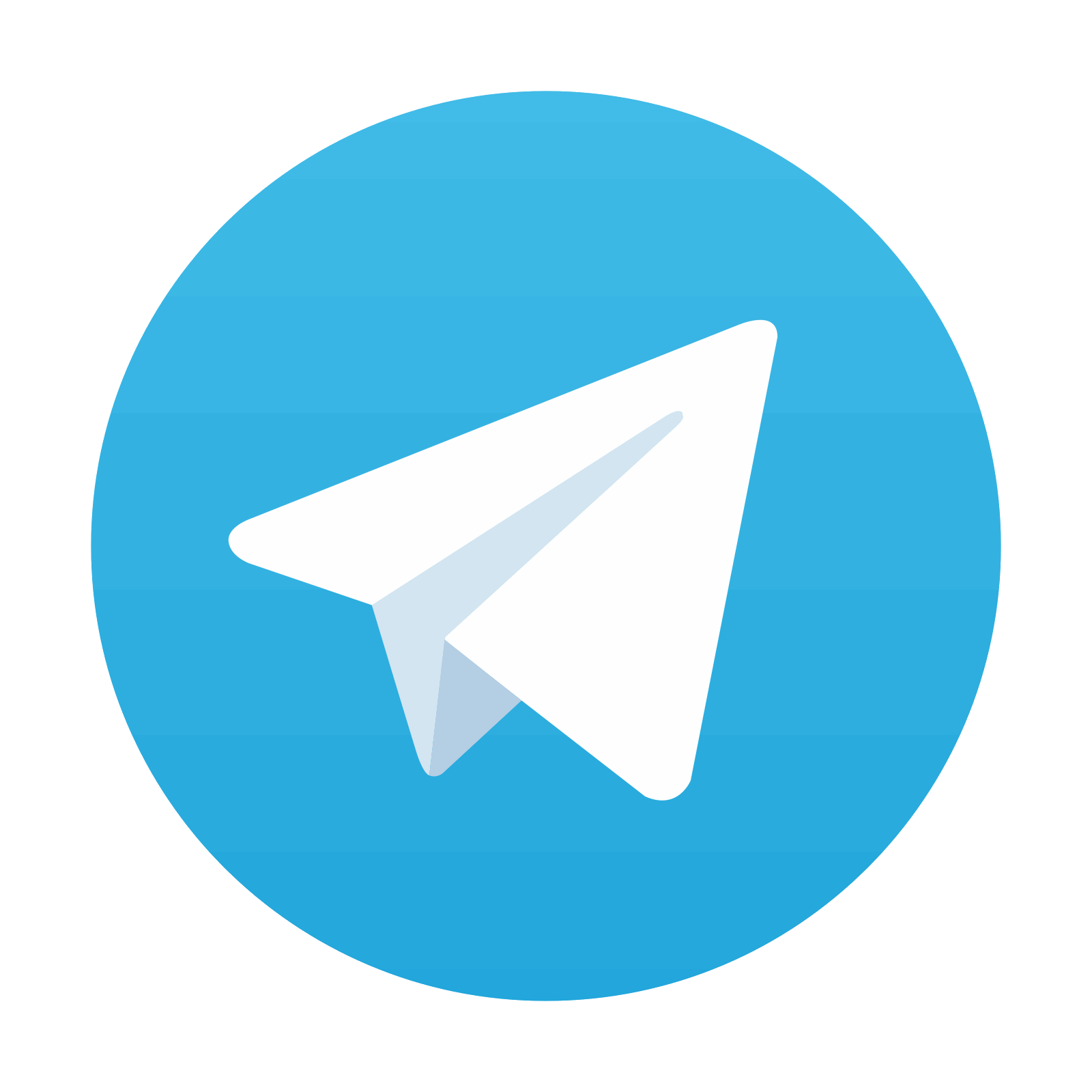
Stay updated, free dental videos. Join our Telegram channel

VIDEdental - Online dental courses
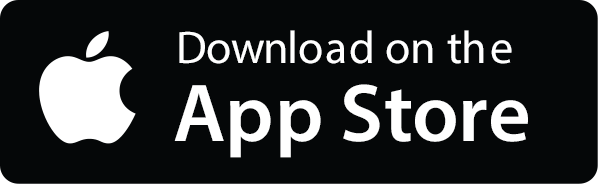
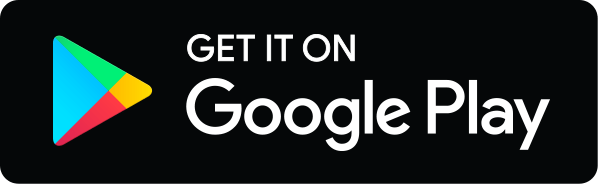