Physical and Chemical Properties of Solids
Terminology
Physical properties are based on the laws of mechanics, acoustics, optics, thermodynamics, electricity, magnetism, radiation, atomic structure, and nuclear phenomena. Hue, value, and chroma relate to color and perception and are physical properties based on the laws of optics, which is the science that deals with the phenomena of light, vision, and sight. Thermal conductivity, diffusivity, and expansion are physical properties based on the laws of thermodynamics. Mechanical properties, a subset of physical properties based on the laws of mechanics, are discussed in Chapter 4.
As examples, the physical properties of color and thermal expansion are of particular importance to the performance of dental ceramics (Chapter 18). Flow and viscosity (the resistance of a fluid to flow) are essential properties of impression materials (Chapter 8). Creep (slow deformation under a static load) is relevant to the clinical performance of amalgam (Chapter 15). Tarnish and corrosion are electrochemical properties that strongly affect the performance of metals and their alloys.
Key Terms
Rheology
Dilatant—Resistance to flow increases as the rate of deformation (shear strain rate) increases. The faster that fluids are stirred or forced through a syringe, the more viscous and more resistant to flow they become.
Pseudoplastic—Viscous character that is opposite from dilatant behavior in which the rate of flow decreases with increasing strain rate until it reaches a nearly constant value. Thus, the more rapidly that pseudoplatic fluids are stirred or forced through a syringe, the more easily they flow.
Rheology—Study of the deformation and flow characteristics of matter (see also viscosity, Chapter 6).
Thixotropic—Property of gels and other fluids to become less viscous and flow when subjected to steady shear forces through being shaken, stirred, squeezed, patted, or vibrated. When the shear force is decreased to zero, the viscosity increases to the original value. Also known as shear thinning, in which the greater the applied shear force, the less the resistance to flow.
Viscosity—Resistance of a fluid to flow (see also rheology).
Color and Optical Effects
Absorption—The extent to which light is absorbed by the material in an object.
Chroma—Degree of saturation of a particular hue (dominant color).
Color—Sensation induced from light of varying wavelengths reaching the eye.
Esthetics—Principles and techniques associated with development of the color and appearance required to produce a natural, pleasing effect in the dentition (Chapter 19). Aesthetics is an alternative spelling with the same meaning.
Hue—Dominant color of an object; for example, red, green, or blue.
Metamerism—Phenomenon in which the color of an object under one type of light source appears to change when illuminated by a different light source.
Opacity—The extent to which light does not pass through a material. No image and no light can be seen through an opaque object.
Reflection—The amount of light that reflects from the surface of an object.
Refract/Refraction—The degree to which light is bent when it passes from one medium to another. This makes a spoon appear bent in a glass of drinking water when light passes from air through glass into water, reflects off of the spoon, and then passes back through water and glass into air. The index of refraction is a measure of this effect.
Translucency—The quality of light passing through an object in a diffuse manner, only to reveal a distorted image that can be viewed through the material.
Transmit/Transmittance—The amount of light passing through an object.
Transparency—The extent to which light passes through a material and to which an undistorted image can be seen through it.
Value—Relative lightness or darkness of a color. Also known as the gray scale.
Thermal Properties
Coefficient of thermal expansion (linear coefficient of expansion)—Change in length per unit of the original length of a material when its temperature is raised by 1 K (1 °C).
K—The kelvin (K) temperature scale extends the degree Celsius scale such that zero degrees K is defined as absolute zero (0 K = −273.15 °C). Temperatures on this scale are called “kelvins,” not “degrees kelvin,” kelvin is not capitalized, and the symbol (capital K) stands alone with no degree symbol. 1 K = 1 °C, K = °C + 273.15.
Thermal conductivity (coefficient of thermal conductivity)—Property that describes the thermal energy transport in watts per second through a specimen 1 cm thick with a cross-sectional area of 1 cm2 when the temperature differential between the surfaces of the specimen perpendicular to the heat flow is 1 K (1 °C).
Thermal diffusivity—Measure of the speed with which a temperature change will proceed through an object when one surface is heated.
Electrochemical Properties
Concentration cell—Electrochemical corrosion cell in which the potential difference is associated with the difference in concentration of a dissolved species, such as oxygen, in solution along different areas of a metal surface. Pitting corrosion and crevice corrosion are types of concentration cell corrosion.
Crevice corrosion—Accelerated corrosion in narrow spaces caused by localized electrochemical processes and chemistry changes, such as acidification and depletion in oxygen content. Crevice corrosion commonly occurs when microleakage takes place between a restoration and the tooth.
Corrosion—Chemical or electrochemical process in which a solid, usually a metal, is attacked by an environmental agent, resulting in partial or complete dissolution.
Electromotive series—Arrangement of metals by their equilibrium values of electrode oxidation potential. Used to judge the tendency of metals and alloys to undergo electrochemical (galvanic) corrosion.
Galvanic corrosion (electrogalvanism)—Accelerated attack occurring on a less noble metal when electrochemically dissimilar metals are in electrical contact within a liquid corrosive environment.
Galvanic shock—Pain sensation caused by the electrical current generated when two dissimilar metals are brought into contact in the oral environment.
Pitting corrosion—Highly localized corrosion occurring at metal surface defects such as pits, scratches, and cracks in which the region at the bottom is oxygen-deprived and becomes the anode while the surface around it becomes the cathode. Thus metal at the base preferentially ionizes and goes into solution, causing the defects to enlarge.
Stress corrosion—Degradation caused by the combined effects of mechanical stress and a corrosive environment, usually exhibited as cracking.
Tarnish—Process by which a metal surface is dulled or discolored when a reaction with a sulfide, oxide, chloride, or other chemical causes surface discoloration through formation of a thin oxidized film.
Rheology
Rheology is the study of the deformation and flow characteristics of matter, whether liquid or solid. Viscosity is the resistance of a fluid to flow. Dental professionals must manipulate a wide variety of dental materials in a fluid state in order to achieve successful clinical outcomes. Moreover, the success or failure of a given material may be as dependent on its manipulation and handling properties in the liquid state as it is on its performance properties as a solid. Most dental materials are initially in a fluid state so that they can be placed and shaped as required; then they undergo transformation to a solid state, in which they are durable and perform their function. Cements and impression materials undergo a fluid-to-solid transformation in the mouth. Gypsum products used in the fabrication of models and dies are transformed extraorally from fluid slurries into solids (see Chapter 9). Amorphous materials such as waxes and resins appear solid but actually are supercooled liquids that can flow plastically (irreversibly) under sustained loading or deform elastically (reversibly) under small stresses (see Chapters 6 and 10). The ways in which these materials flow or deform when subjected to stress are important to their use in dentistry. The study of flow characteristics of materials is the basis for the science of rheology (also see Chapter 6).
This concept is illustrated in Figure 3-1. A liquid occupies the space between two flat surfaces, as, for example when a spatula is moved through a pasty fluid such as a dental cement to blend two components on a mixing pad. The mixing surface is fixed, and the upper surface (e.g., a spatula blade) moves to the right at a given velocity (V). A force (F) is required to overcome the frictional resistance within the fluid (i.e., the viscosity) and cause the fluid to flow. As discussed in Chapter 4, stress is the force per unit area that develops within a structure when an external force is applied. This stress causes a deformation, or strain, to develop. Strain is calculated as a change in length divided by the initial reference length. If the two surfaces have an area (A) in contact with the liquid, a shear stress (τ) can be defined as τ = F/A. The shear strain rate, or rate of change of deformation, is ε = V/d, where d is the shear distance of the upper surface relative to the fixed lower surface and V is the velocity of the moving surface. As the shear force F increases, V increases, and a curve can be obtained for force versus velocity. This type of curve is analogous to the load-versus-displacement curves discussed in Chapter 4, which are derived from static measurements on solids.
Curves depicting shear stress versus shear strain rate are used to characterize the viscous behavior of fluids. The rheologic behaviors of four types of fluids are shown in Figure 3-2. An “ideal” fluid produces a shear stress proportional to the strain rate. That is, the greater the force applied, the faster the fluid flows and the plot is a straight line. This is known as Newtonian viscosity. Because viscosity (h) is defined as the shear stress divided by the strain rate, τ/ε, a Newtonian fluid has a constant viscosity and exhibits a constant slope of shear stress plotted against strain rate, as seen in Figure 3-2. The plot is a straight line and resembles the elastic portion of a stress-strain curve (see Chapter 4), with viscosity the analog of the elastic modulus (elastic stress divided by elastic strain). Viscosity is measured in units of megapascals (MPa) per second, or centipoise (cP). Pure water at 20°C has a viscosity of 1.0 cP, whereas the viscosity of molasses is approximately 300,000 cP. This value is similar to that of tempered agar hydrocolloid impression material (281,000 cP at 45 °C). Of the elastomeric impression materials, light-body (“thin” consistency) polysulfide has a viscosity of 109,000 cP, compared with 1,360,000 cP for heavy-body (“thick” consistency) polysulfide at 36 °C.
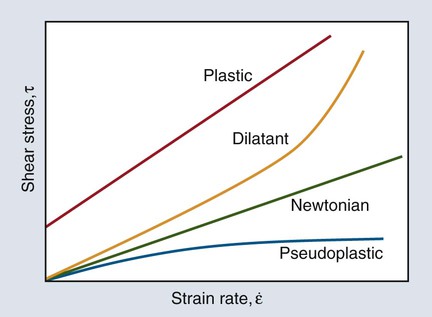
The viscosity of many dental materials decreases with increasing strain rate until it reaches a nearly constant value. That is, the faster they are stirred, forced through a syringe, or squeezed, the less viscous and more fluid they become. This is pseudoplastic viscosity and is illustrated by the change in slope of the plot in Figure 3-2. Liquids that show the opposite behavior are dilatant and become more rigid as the rate of deformation (shear strain rate) increases. That is, the faster they are stirred, etc., the more viscous and resistant to flow they become.
Structural Relaxation
Stress Relaxation
The displaced atoms are not in equilibrium positions and are therefore unstable. Through a solid-state diffusion process driven by thermal energy, the atoms can slowly return to their equilibrium positions. The result is a change in the shape or contour of the solid as the atoms or molecules change positions. The material warps or distorts. Such stress relaxation is a problem with elastomeric impression materials and can lead to distortions in the impression and subsequent lack of fit, as discussed in Chapter 8.
Creep and Flow
If a metal is held at a temperature near its melting point and is subjected to a constant applied stress, the resulting strain will increase over time. Creep is defined as the time-dependent plastic strain of a material under a static load or constant stress. The related phenomenon of sag occurs in the permanent deformation of long-span metal bridge structures at porcelain-firing temperatures under the influence of the mass of the prosthesis. For a given thickness, a greater bridge mass is related to greater flexural stress and greater flexural creep. Metal creep usually occurs as the temperature increases to within a few hundred degrees of the melting range. Metals used in dentistry for cast restorations or substrates for porcelain veneers have melting points (for pure metals) or melting ranges (for alloys) that are much higher than mouth temperatures, and they are not susceptible to creep deformation intraorally. However, some alloys used for metal-ceramic prostheses can creep at porcelain veneering temperatures. This phenomenon is discussed further in Chapter 18.
Dental amalgams contain from 42% to 52% of mercury by weight and begin melting at temperatures only slightly above room temperature. (The melting range of an alloy is discussed in Chapter 5.) Because of its low melting range, dental amalgam can undergo creep at a restored tooth site under periodic sustained stress, such as would be imposed by patients who clench their teeth. Because creep produces continuing plastic deformation, the process can, over time, be very destructive to a dental amalgam filling. The relationship of this property to the behavior of amalgam restorations is discussed in Chapter 15. A creep test is required in American National Standards Institute/American Dental Association Specification No. 1 for amalgam alloy and in International Organization for Standardization (ISO) standard No. 1559.
The term flow, rather than creep, has generally been used in dentistry to describe the rheology of amorphous materials such as waxes. The flow of wax is a measure of its potential to deform under a small static load, even that associated with its own mass. Although creep or flow may be measured under any type of stress, compression is usually employed in the testing of dental materials. A cylinder of prescribed dimensions is subjected to a given compressive stress for a specified time and temperature. The creep or flow is measured as the percentage decrease in length that occurs under these testing conditions. Creep may cause unacceptable deformation of dental restorations (such as low-copper dental amalgam) made from a material that is used clinically at a temperature near its melting point for an extended period. Creep may also lead to an unacceptable fit of fixed dental prosthesis frameworks when a cast alloy with poor creep (sag) resistance is veneered with porcelain at relatively high temperatures (about 1000 °C; see Chapter 18).
Color and Optical Effects
Since esthetic dentistry imposes severe demands on the artistic abilities of the dentist and technician, knowledge of the underlying scientific principles of color and other optical effects is essential. This is especially true for the increasingly popular restorations that involve ceramic materials (see Chapter 18). More comprehensive treatments of this subject can be found in the “Selected Readings” at the end of this chapter.
Nature of Light and the Role of Human Vision
Light is electromagnetic radiation that can be detected by the human eye. The eye is sensitive to wavelengths from approximately 400 nm (violet) to 700 nm (dark red), as shown in Figures 3-3 and 3-4. The reflected light intensity and the combined intensities of the wavelengths present in incident and reflected light determine the appearance properties of hue, value, and chroma, which are shown in Figure 3-5 and discussed in the next section. For an object to be visible, it must reflect or transmit light incident on it from an external source. The incident light is usually polychromatic; that is, a mixture of the various wavelengths, commonly known as “white” light. Incident light is selectively absorbed or scattered (or both) at certain wavelengths. The spectral distribution of the transmitted or reflected light resembles that of the incident light, although certain wavelengths are reduced in magnitude.
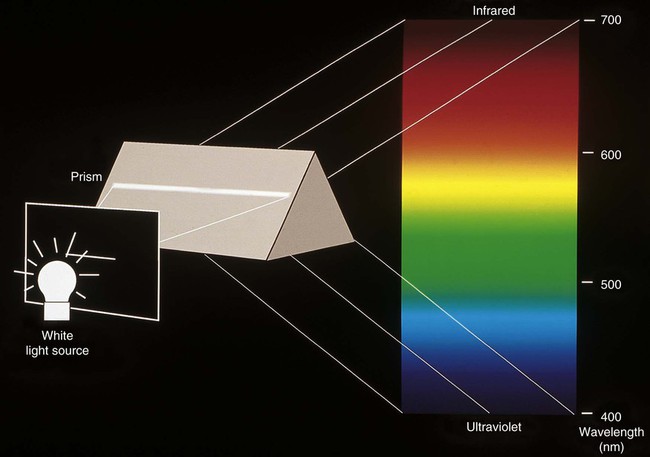
The phenomenon of vision, and certain related terminology, can be illustrated by considering the response of the human eye to light reflected from an object. Light from an object that is incident on the eye is focused in the retina and is converted into nerve impulses, which are transmitted to the brain. Cone-shaped cells in the retina are responsible for color vision. These cells have a threshold intensity required for color vision and respond to wavelengths as shown in Figure 3-4 for both normal color vision and color-deficient vision. Someone with normal vision has maximum sensitivity in the green-yellow region at about 550 nm and is least sensitive in the red and blue-violet regions of the spectrum.
Three Dimensions of Color
Verbal descriptions of color are not precise enough to describe the appearance of teeth. For example, the definition of puce is “a brilliant purplish-red color,” according to Microsoft’s Encarta World English Dictionary, while Webster’s Third New International Dictionary defines it as “a dark red that is yellower and less strong than cranberry, paler and slightly yellower than average garnet, bluer, less strong, and slightly lighter than pomegranate, and bluer and paler than average wine.” These definitions are too variable, complex, and imprecise to describe a desired color of a dental crown to a laboratory technician. Such a written description is subjective and does not clearly and unambiguously allow one to perceive the color. To overcome this problem, color perception is described by three objective variables: hue, value, and chroma. These three parameters constitute the three dimensions of “color space,” as shown in Figure 3-5.
• Hue: The dominant color of an object, for example red, green, or blue. This refers to the dominant wavelengths present in the spectral distribution. The continuum of these hues creates the 3-D color solid shown in Figure 3-5.
• Value: Value is also known as the gray scale. It is the vertical, or Z-axis, of Figure 3-5. Value increases toward the high end (lighter) and decreases toward the low end (darker). Value is also expressed by the “lightness” factor (L* in Figure 3-6), with varying levels of gray between the extremes of white and black. Teeth and other objects can be separated into lighter shades (higher value) and darker shades (lower value). For example, the yellow of a lemon is lighter than the red of a cherry. For a light-diffusing and light-reflecting object such as a tooth or dental crown, value identifies the lightness or darkness of a color, which can be measured independently of the hue.
The components of a color space can be more easily visualized by its individual parts in Figure 3-5, B, here seen as discs stacked along the value axis (lightness, L*) on a scale of 0 to 10 from black to white. Around the periphery are 10 basic hues (dominant wavelength/color). Chroma (strength, saturation) radiates out from the value axis like the spokes of a wheel (illustrated by green).
Figure 3-6 represents a horizontal plane, perpendicular to the L* (value) axis, through the color solid in Figure 3-5. This color chart is based on the Commission Internationale de l’Éclairage L*a*b* color space, in which L* represents the value of an object, a* is the measurement along the red-green axis, and b* is the measurement along the yellow-blue axis. The color of a red apple is shown by the letter A in the upper and lower charts. Its color appearance can be expressed by L* = 42.83, a* = 45.04, and b* = 9.52. In comparison, a dental body (gingival) porcelain of shade A2 can be described by a higher (lighter) L* of 72.99, a lower a* of 1.00, and a higher b* of 14.41.
• Chroma: Chroma is the degree of saturation of a particular hue. For example, red can vary from “scarlet” to light pink, where scarlet has a high saturation and pink has a low saturation. The yellow color of a lemon is a more saturated, “vivid,” color than that of a banana, which is a less saturated, “dull” yellow. Chroma varies radially, perpendicular to the value/L* axis (see Figure 3-6, A, near the bottom right). Colors in the center are dull (gray). In other words, the higher the chroma, the more intense the color. Chroma is not considered separately in dentistry. It is always associated with the hue and value of dental tissues, restorations, and prostheses. One can see the relationship among these dimensions of color in the adjustments on a color television set, which use the same principles of hue, value, and chroma.
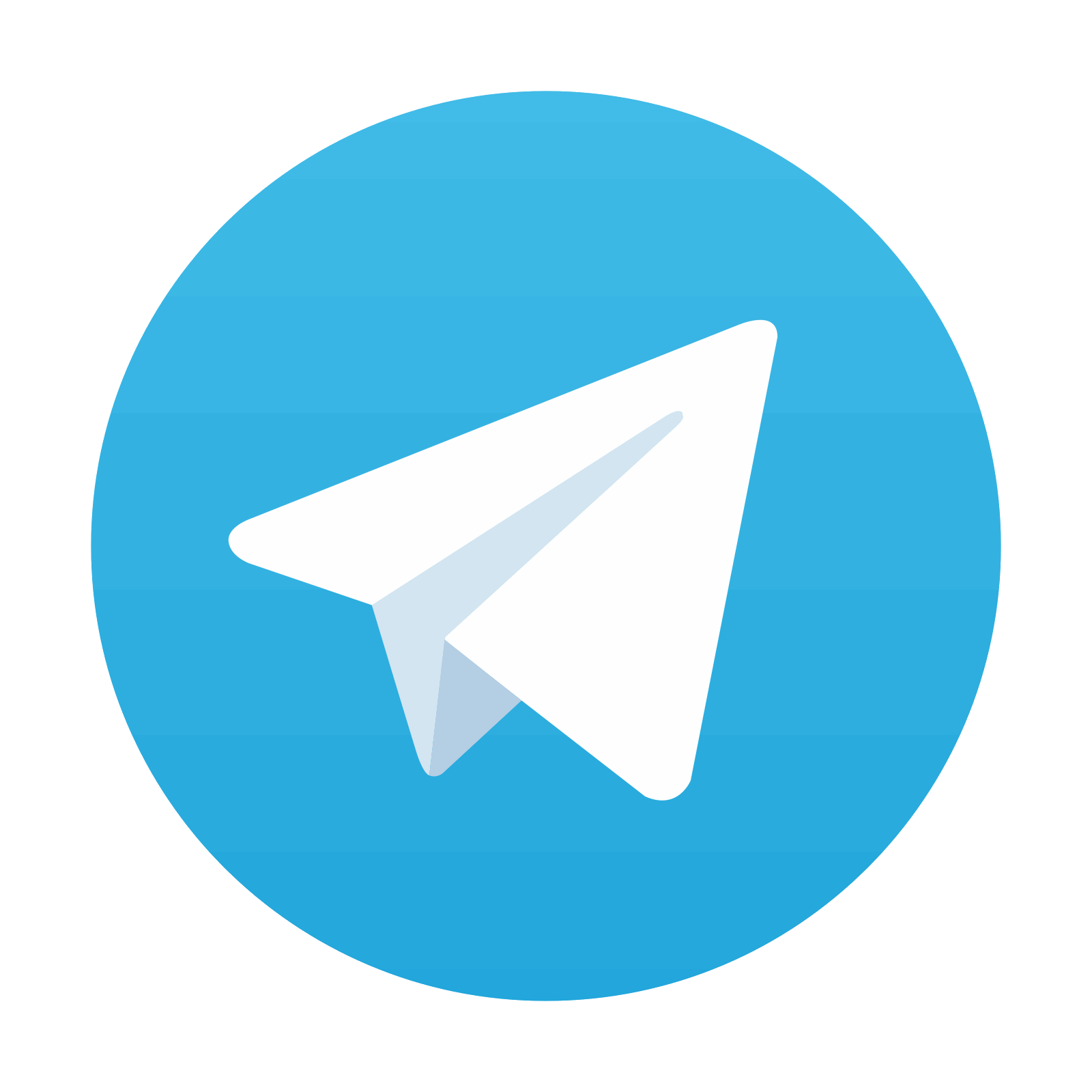
Stay updated, free dental videos. Join our Telegram channel

VIDEdental - Online dental courses
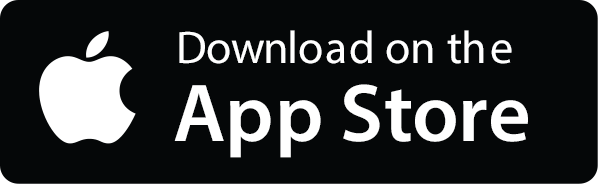
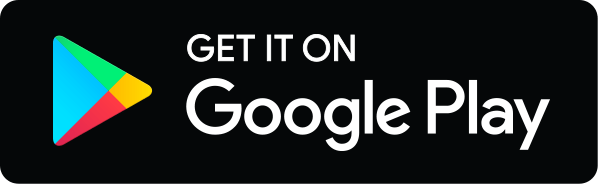