2 Laser Fundamentals
Light
Light is a form of electromagnetic energy that exists as a particle and that travels in waves at a constant velocity. The basic unit of this radiant energy is called a photon.1 The waves of photons travel at the speed of light and can be defined by two basic properties: amplitude and wavelength (Figure 2-1). Amplitude is defined as the vertical height of the wave from the zero axis to its peak as it moves around that axis. This correlates with the amount of intensity in the wave: the larger the amplitude, the greater the amount of potential work that could be performed. For a sound wave, amplitude correlates to loudness. For a wave emitting light, amplitude correlates to brightness. A joule (J) is a unit of energy; a useful quantity for dentistry is a millijoule (mJ), or one thousandth (10−3) of a joule ( J; 0.001 J).
Using a household fixture as an example, a 100-watt lamp will produce a moderate amount of light for a room area, with some heat. On the other hand, two watts (2 W) of laser light can be used for precise excision of a fibroma while providing adequate hemostasis at the surgical site, without disturbing the surrounding tissue.2
Amplification
Surrounding this active medium is an excitation source, such as a flashlamp strobe device, electrical circuit, electrical coil, or similar source of energy that pumps energy into the active medium. When this pumping mechanism pumps energy into the active medium, the energy is absorbed by the electrons in the outermost shell of the active medium’s atoms. These electrons have absorbed a specific amount of energy to reach the next shell farther from the nucleus, which is at a higher energy level. A “population inversion” occurs when more of the electrons from the active medium are in the higher energy level shell farther from the nucleus than are in the ground state (Figure 2-2). The electrons in this excited state then spontaneously give off that energy in the form of a photon (Figure 2-3). This is called spontaneous emission (Figure 2-4).
Completing the laser cavity are two mirrors, one at each end of the optical cavity, placed parallel to each other; or in the case of a semiconductor, two polished surfaces at each end. These mirrors or polished surfaces act as optical resonators, reflecting the waves back and forth, and help to collimate and amplify the developing beam. A cooling system, focusing lenses, and other controlling mechanisms complete the mechanical components. Figure 2-5 shows a schematic of a gas or solid active-medium laser (e.g., CO2, Nd:YAG). Figure 2-6 shows a schematic of a semiconductor diode device.
Stimulated Emission
Stimulated emission is the process by which laser beams are produced inside the laser cavity. The theory of stimulated emission was postulated by Albert Einstein in 1916.3 He based his work on some earlier work by physicists from Germany (Max Planck) and Denmark (Niels Bohr), who theorized a model of the atom as well as the quantum theory of physics, which described a quantum as the smallest unit of energy emitted from an atom.4,5 Einstein used this concept and further theorized that an additional quantum of energy may be absorbed by the already-energized atom and would result in a release of two quanta (Figure 2-7). This energy is emitted, or radiated, as identical photons, traveling as a coherent wave. These photons in turn are then able to energize more atoms in a geometric progression, which further causes the emission of additional identical photons, resulting in an amplification of the light energy, thus producing a laser beam (Figure 2-8).
Radiation
The light waves produced by the laser are a specific form of radiation, or electromagnetic energy.6 The electromagnetic spectrum is the entire collection of wave energy, ranging from gamma rays, with wavelengths of 10 to 10−12 m, to radio waves, with wavelengths of thousands of meters. All currently available dental laser devices have emission wavelengths of approximately 0.5 µ, or 500 nm, to 10.6 µ, or 10,600 nm, which places them in either the visible or the invisible nonionizing portion of the electromagnetic spectrum, as shown in Figure 2-9. It is important to note that the dividing line between the ionizing, cellular DNA mutagenic, portion of the spectrum and the nonionizing portion is at the junction of ultraviolet and visible-violet light. Thus, all dental lasers emit either a visible-light wavelength or an invisible, infrared-light wavelength in the portion of the nonionizing spectrum called thermal radiation.7
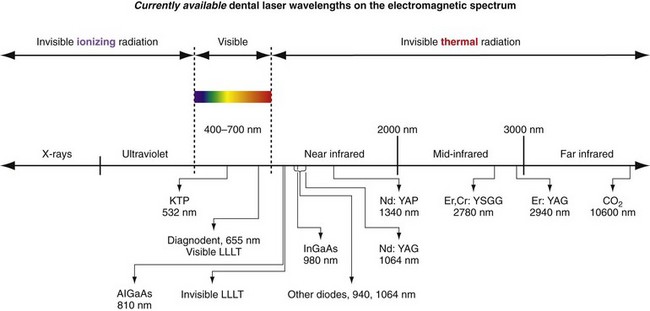
FIGURE 2-9 • Part of electromagnetic spectrum showing division of ionizing, visible portion and nonionizing portion.
The following four dental laser instruments emit visible light:


Laser Delivery Systems
Laser energy should be delivered to the surgical site by a method that is ergonomic and precise.8 Shorter-wavelength instruments (e.g., KTP, diode, Nd:YAG) have small, flexible fiberoptic systems with bare glass fibers that deliver the laser energy to the target tissue (Figure 2-10). Because the erbium and CO2 laser wavelengths are absorbed by water, which is a major component of conventional glass fibers, these wavelengths cannot pass through these fibers. Erbium and CO2 devices therefore are constructed with special fibers capable of transmitting the wavelengths, with semiflexible hollow waveguides, or with articulated arms (Figure 2-11). Some of these systems employ small quartz or sapphire tips that attach to the laser device for contact with target tissue; others employ noncontact tips (Figure 2-12). In addition, the erbium lasers employ a water spray for cooling hard tissues. Lasers may have different fiber diameters, handpieces, and tips (Figure 2-13). The diameter of the fiber, handpiece, and tip plays a significant role in the delivery of energy (Figure 2-14).

FIGURE 2-10 • An assembled fiberoptic delivery system consisting of the bare fiber, a handpiece, and a disposable tip.
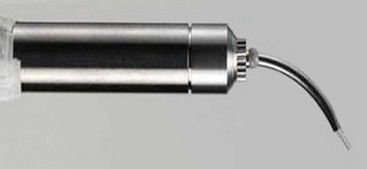
FIGURE 2-12 • Quartz tip assembled onto the end of a handpiece for a fiber optically delivered erbium laser.
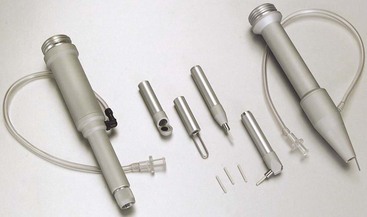
FIGURE 2-13 • Variety of handpieces available with most CO2 laser systems offer a variety of spot sizes and focal distances.
All conventional dental instrumentation, either hand or rotary, must physically touch the tissue being treated, giving the operator instant feedback. As mentioned, dental lasers can be used either in contact or out of contact. The fiber tip can easily be inserted into a periodontal pocket to remove small amounts of granulomatous tissue (Figures 2-15 to 2-17). In noncontact use, the beam is aimed at the target some distance away (Figure 2-18). This modality is useful for following various tissue contours, but with the loss of tactile sensation, the surgeon must pay close attention to the tissue interaction with the laser energy. All the invisible-light dental lasers (Nd:YAG, CO2, diode, erbium) are equipped with separate aiming beams, which can be either a laser or a conventional light. The aiming beam is delivered coaxially along the fiber or waveguide and shows the operator the exact spot where the laser energy will strike the tissue.
Spot Size
The active beam is focused by lenses. With hollow-waveguide or articulated-arm delivery systems, there is a precise spot at the point where the energy is the greatest. This focal point is used for incision and excision surgery. For fiberoptic delivery systems, the focal point is at or near the tip of the fiber, which again has the greatest energy. For CO2 lasers, which are used out of contact, the focal point may be anywhere from 1 mm to 12 mm from the tissue surface, depending on the handpiece being used (Figure 2-19). When the handpiece is moved away from the tissue and away from the focal point, the beam is defocused (out of focus), becomes more divergent, and therefore delivers less energy to the surgical site (Figure 2-20). At a small divergent distance, the beam can cover a wider area, which would be useful in achieving hemostasis. At a greater distance, the beam will lose its effectiveness because the energy will dissipate.
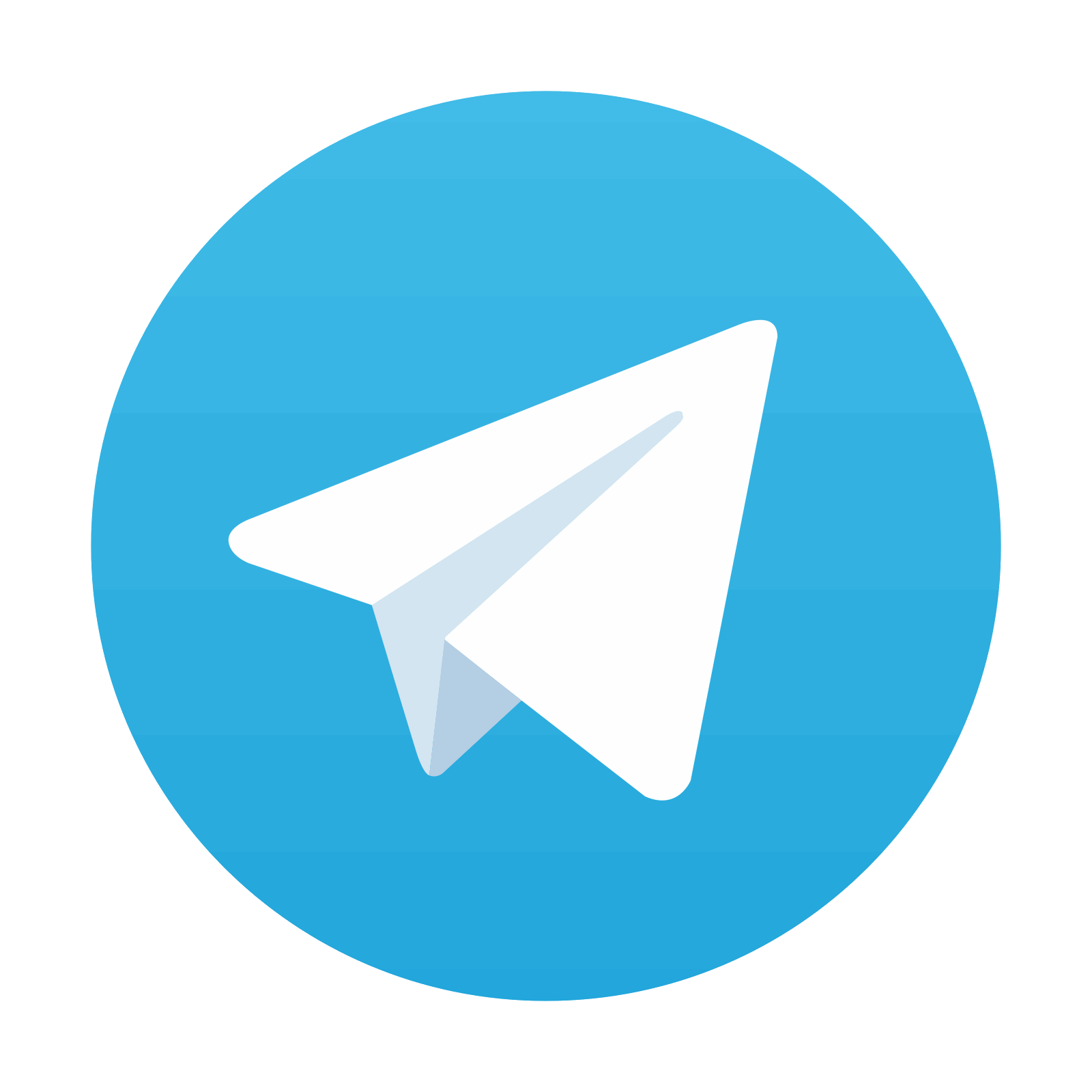
Stay updated, free dental videos. Join our Telegram channel

VIDEdental - Online dental courses
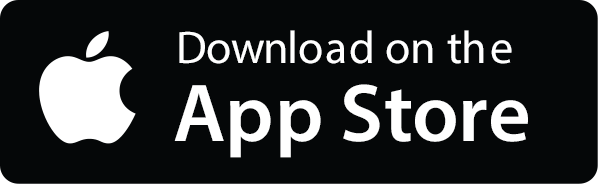
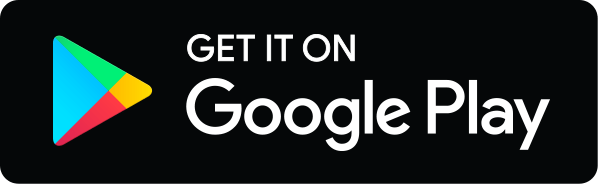