11 Lasers in Restorative Dentistry
Caries Removal: Background and Debate
The U.S. National Health and Nutrition Examination Survey (NHANES), in an extrapolated survey (1999-2002) of the noninstitutionalized civilian population, found that 41% of children age 2 to 11 years had dental caries in their primary teeth, and that 42% of children and adolescents age 6 to 19 years and about 90% of adults had dental caries in their permanent teeth.1 Other studies reflect even greater prevalence of caries, as high as 96% of children 6 to 7 years old in Saudi Arabia.2 Such findings indicate a continued demand for interceptive restorative treatment.
In addition to such prevalence, there is growing skepticism toward the two basic mainstays of restorative dentistry: the cavity classification of G.V. Black (Classes I-V) and the continued use of amalgam as a restorative material.3 Black’s ideology, based on the dogma “extension for prevention,” has often resulted in the removal of large areas of otherwise healthy enamel and dentin. This has been exacerbated by the need to address the mechanical success of amalgam: outline form and retention form of cavity design. Studies assessing the iatrogenic damage to natural tooth tissue during restorative procedures report microfractures adjacent to cavity margins, damage to teeth adjacent to interproximal cavity design,4 and thermal injury to the pulp caused by rotary instrumentation.5
Despite the gradual reduction in amalgam restorations being placed (56 to 52 million in United States6; 8 to 6 million in United Kingdom7), there is still a considerable need to service existing metal restorations. However, the evolution of non–metal-based composite restorations as well as a desire for cosmetic treatment from a patient base with greater awareness and expectations has resulted in a shift toward smaller restorations, a change in cavity design (e.g., “tunnel” restorations8), and a greater reliance on acid-etch microretention techniques.9,10
Instrumentation and Lasers
The most common procedures for removing diseased dental hard tissue during restorative procedures involve the use of excavators and burs. The accuracy of such instrumentation is questioned,11 with minimal objective analysis of total removal of demineralized, infected enamel and dentin, as well as the possible removal of excessive amounts of sound tissue.12 On the other hand, the use of a suitable laser wavelength may allow a much more conservative approach to the preservation of sound, mineralized tooth tissue, with preferential removal of higher-water-content caries, greater precision, and reduced bacterial contamination of the laser-prepared cavity.13,14
A key factor in pulpal injury is thermal conduction from the range of instrumentation chosen.15 Studies have established that rotary instrumentation can cause conductive thermal elevation in excess of 20° C above 37.4° C.16,17 With regard to laser irradiation of dental tissue, the explosive defragmentation resulting from water-assisted mid-infrared (IR) wavelengths allows much of the heat to escape from the cavity (carried in ablated particles), resulting in pulpal thermal increase of less than 5° C.18–20 The affinity of mid-IR laser wavelengths for water allows the main absorption to take place in demineralized tissue richer in organic material and with a higher percentage of water, thus protecting any sound tissue overlying the pulp, with a reduced penetration of the beam (Figure 11-1). With adoption of operating parameters to standardize the normal clinical use of each instrument, evidence-based protocols now serve to provide what is best for the patient. As treatment shifts toward early diagnosis and interceptive action that is more selective in preserving healthy tissue, the choice of a laser may become more common, placing the dental profession in an increasingly responsible position to deliver patient-centered restorative care.
The claimed precision of laser use in many areas of surgery would appear to complement the demands of the restorative dentist. Also, although a tooth was the first tissue to be exposed to laser light in Maiman’s 1960 investigations,21 the first “dental” laser did not become commercially available until 1989. The chosen wavelength of this laser, neodymium-doped yttrium-aluminum-garnet (Nd:YAG), emitting at a wavelength of 1064 nm, has limited therapeutic action on hard dental tissue. More recent developments led to the erbium, chromium–doped yttrium-scandium-gallium-garnet (Er,Cr:YSGG, 2780 nm) and Er:YAG (2940 nm) laser wavelengths, for use in cavity preparation.
Table 11-1 summarizes the advantages of laser use in dental restorative procedures.
Table 11-1 Summary of Comparable Benefits of Laser Use over Rotary Instrumentation (“Airotor”) in Tooth Cavity Preparation
Restorative Procedure | Rotary | Laser* |
---|---|---|
Cutting enamel/dentin | Yes | Yes |
Selective removal of caries | No | Yes |
Precision | Precise >1000-2000 µ | Precise <300 µ |
Smear layer | Smear layer produced | No smear layer |
Thermal rise | Thermal rise >15° C | Thermal rise <5° C |
Risk of iatrogenic damage | Greater | Less |
Noise/vibration | >120 dB/vibration | <120 dB/no vibration |
Bactericidal action | No | Surface decontamination |
Speed of cutting enamel | Fast | <30% rotary speed |
Speed of cutting dentin | Fast | Comparable |
Contact with tooth tissue | Contact required | Noncontact possible |
Pain response | High | Less pain/no pain |
Laser Photonic Energy–Hard Tissue Interaction
Healthy coronal hard tissue comprises enamel and primary and secondary dentin. By volume, enamel is 85% mineral (predominantly carbonated hydroxyapatite), 12% water, and 3% organic proteins; most free water exists in the periprismatic protein matrix. Dentin has higher water content, being 47% mineral (carbonated hydroxyapatite), 33% protein (mostly collagen), and 20% water. Water content in carious dentin may be as high as 54%.22
With such a breadth of target elements, several laser wavelengths have been investigated as possible adjuncts in cutting tooth cavities and removing carious tissue. The absorption coefficients of water and mineralized dental tissue are shown in Figure 11-2.
Early investigation into the Nd:YAG laser, first marketed for soft tissue laser dentistry in the United States, was done to establish its utility for hard tissue.23–27 This included the ablation of pigmented and diseased tissue, the antibacterial effect of this laser wavelength, and the possible effects on the vital dental pulp. Although studies established safe and effective parameters, the Nd:YAG’s clinical significance was of only marginal benefit to the restorative dentist because of its very low absorption in sound enamel or dentin. Further, several studies concluded that the Nd:YAG wavelength could cause unwanted heating effects, such as cracking and melting of mineral structures28–30 (Figure 11-3).
Early studies on enamel ablation also used the other available laser wavelength, carbon dioxide (CO2, 10,600 nm), but this laser gave poor interactions, with reports of charring, cracking, and damaging heat buildup in tooth and bone structure.31,32 Although examination of absorption data of this wavelength with hydroxyapatite shows an effective interaction, current CO2 lasers employ continuous-wave (CW) emission with no cooling water, which results in very high energy deposition in the hard tissue (Figure 11-4).
In the mid-1990s, use of a more suitable laser wavelength by Keller and Hibst33,34 led to the development of the Er:YAG (2940 nm) wavelength, a free-running (inherently microsecond-pulsed) mid-IR laser for effective ablation of dental hard tissues. This was followed by similar investigations into the use of another mid-IR free-running wavelength, the Er,Cr:YSGG (2780 nm), which could effect photothermic vaporization of interstitial water and ablative disruption of target tooth mineral safely and precisely.35–38
Relatively, enamel, dentin, bone, cementum, and carious tissue have descending mineral density and ascending water composition.39,40 Both Er,Cr:YSGG and Er:YAG laser wavelengths are absorbed well in water, with the Er:YAG slightly more strongly absorbed in water than the Er,Cr:YSGG (see Figure 11-2). This absorption is several orders of magnitude greater than seen with the Nd:YAG wavelength (Figure 11-5).
The absorption in water with the erbium wavelengths results from a relatively broad water band of about 3000 nm, together with small absorption at about 2800 nm by the hydroxyl group of the (carbonated) hydroxyapatite mineral of the tissues.41–44
When incident laser energy directed onto hard dental tissue is absorbed by the prime chromophores (either water or carbonated hydroxyapatite), one of two effects occurs. For both Er:YAG and Er,Cr:YSGG wavelengths, this energy is absorbed primarily by the water and is rapidly converted to heat, which causes superheating and a phase transfer in the subsurface water, resulting in a disruptive expansion in the tissue. Through this mechanism, whole tissue fragments are ejected and a hole is cut in the tooth, with little or no alteration to the mineral itself. A common term for this effect is spallation (Figures 11-6 to 11-8, pp. 186-187).
Relatively high fluences (energy density–laser photonic energy per unit area) are needed at these wavelengths for spallation to occur. The emission mode of current mid-IR lasers is defined as free-running pulsed. Currently available lasers emit a pulse train of 50 to 250 microsecond pulses on average, which, when delivered in rates of 3 to 50 Hz (pulses per second), represent high peak-power values sufficient to ablate tooth mineral tissue. Although pulse durations are close to the thermal relaxation times of enamel and dentin, further examination of ultrashort pulsewidths (and associated high peak-power values) is needed to create sufficient ablative force without inducing collateral thermal damage.45,46
The rate of ablation of dental hard tissue depends on the amount of incident laser energy delivered to the tissue, as well as the effects of wavelength, pulse duration, pulse shape, repetition rate, power density, thermal relaxation time of the tissue, and delivery mode.47,48 In addition, heat buildup in the tissue (and unwanted heat conduction to the pulp) must be avoided and accumulation of products of ablation (char), prevented.
Mid-IR ablation of dental hard tissue has led to the concept of two wave fronts of interaction: an ablation front and a thermal front. It is important that the ablation front always precedes the thermal front, to avoid the risk of a damaging heat increase through the accumulation of ablation debris within a deep cavity.49 Studies therefore have examined the effects of excessive incident power and the buildup of ablation products, or their removal by means of a coaxial water spray.50 The vital dental pulp is acutely sensitive to thermal change. The explosive defragmentation of mineralized dental tissue, resulting from water-assisted erbium wavelengths, allows much of the heat to escape from the cavity, carried in the ablated particles and resulting in pulpal thermal increases of less than 5° C.51–53
Laser Use vs. Conventional Instrumentation
To any clinician who chooses to use a laser in restorative dentistry, the high-speed rotary drill is seen as the “gold standard.” Ease of use and speed are often accepted as plausible, despite several studies showing that high-speed drilling leads to surface and pulpal temperature rise, tissue cracking, and unnecessary removal of healthy surrounding tissue during cavity preparation54–56 (Figure 11-9).
The precise and selective ablation of tooth tissue with the Er,Cr:YSGG and Er:YAG laser wavelengths is well documented. Generally, the only drawback appears to be the lower “speed” of cutting compared to a slow-speed drill,57–59 with claims of 80% slower in enamel and comparable speed in dentin. Also, the desire to match laser cutting speeds with those of rotary instruments has led to power delivery greatly in excess of that postulated by Keller and Hibst, relative to the ablation threshold of enamel. Coexistent with such power levels and heat conversion, studies have shown that by reducing the pulse duration of the laser energy (pulsewidth), peak-power values rise, ablation is more efficient, and heat transfer is minimized,33,60–62 to establish a clinically acceptable rate of interaction commensurate with treatment time.
Different handpiece tips have been designed to address the needs of energy delivery, efficiency of cutting, and access. Round cross-sectional tips may vary in diameter from 200 to 1300 µm. Care should be exercised in assessing the power density of the emitted beam; as the spot size (diameter) is reduced, the energy per exposed target area increases dramatically, assuming all other variables remain the same, and places a high risk of tissue overheating. Most delivery tips are made of quartz and provide efficient transmission of laser energy to the target. With the spallation effect of hard tissue ablation, impact damage from ejected ablation products may cause irregularity in both the beam configuration and the cutting dynamics. Thus, the tips should be regularly inspected for damage, and the ends can be repolished using fine discs and diamond paste. Tips made of sapphire offer marginal improvements in energy delivery, as do hollow-bore tips.63,64 However, sapphire tips are more expensive and less able to be reconditioned, and the rigidity places these tips at greater risk of fracture during use.
Laser Use in Cavity Preparation
The use of Er:YAG and Er,Cr:YSGG lasers for more than a decade in clinical practice has resulted in protocols governing their use in restorative dentistry, from simple fissure sealing to complete cavity management and surface preparation for direct composite-resin veneers. The spallation effect of exposing the enamel surface to laser energy results in a microcavitation; although ideal for further acid etching and direct bonding, it is considered impractical to use such a surface to place indirect ceramic veneers. Nonetheless, some advocate laser use in crown and veneer preparation65,66 despite the lack of peer-reviewed literature to validate the use of these lasers for crown preparation. (Figures 11-10 and 11-11).
Early erbium lasers had rudimentary handpieces that were comparatively heavy. In addition, the delivery of laser energy through a noncontact sapphire window proved inaccurate in the delivery of precise cutting action. Newer developments have resulted in balanced waveguides or low-OH− fibers (e.g., germanium oxide), together with handpieces that are similar to turbines, use contact tips, and have coaxial illumination and water spray. This allows more precise interaction with tooth tissue, using instrumentation familiar to the surgeon (Figure 11-12, p. 190).
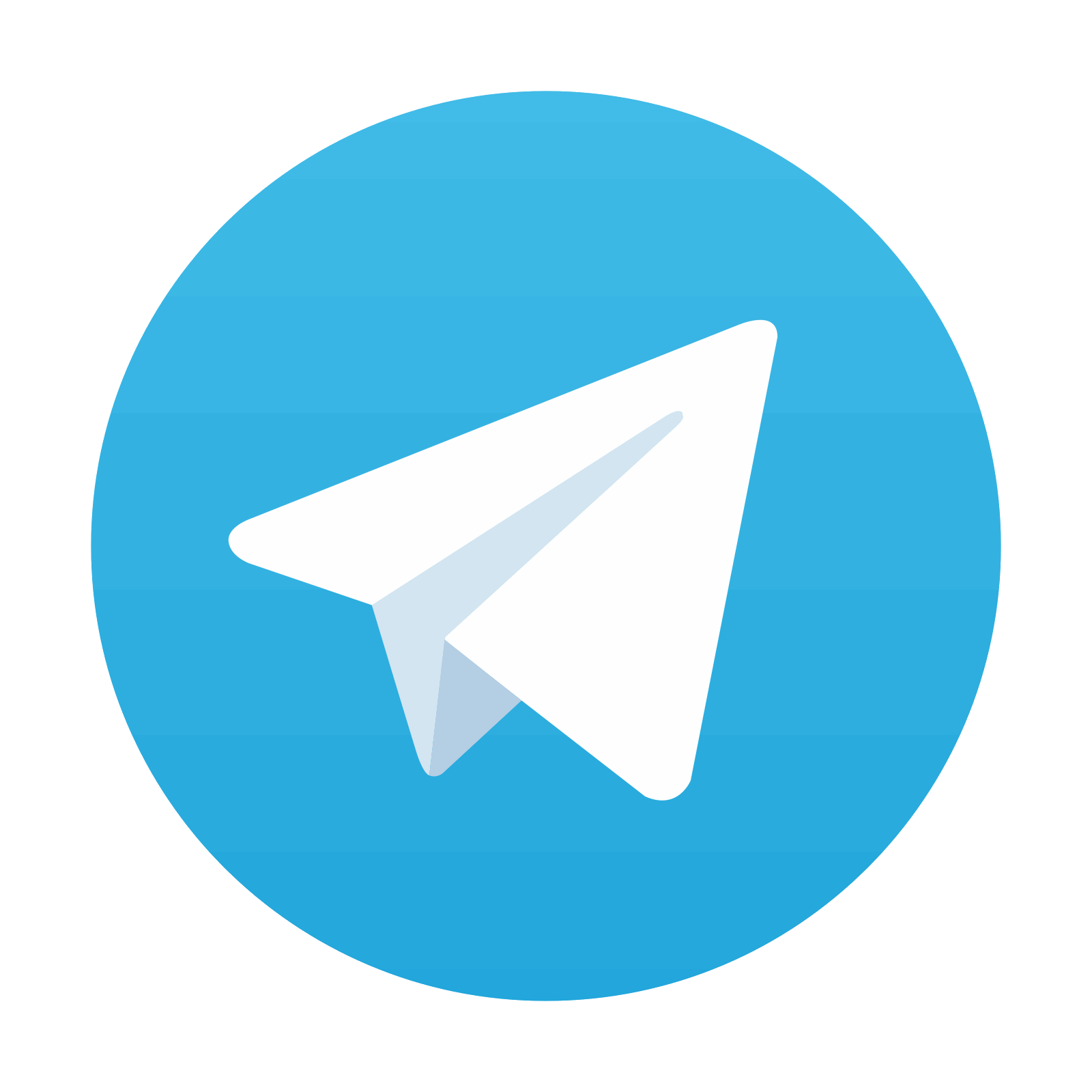
Stay updated, free dental videos. Join our Telegram channel

VIDEdental - Online dental courses
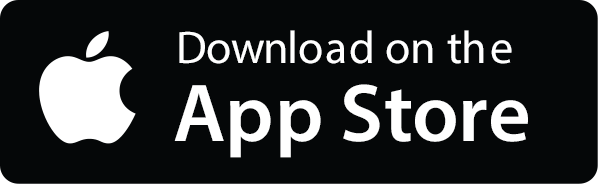
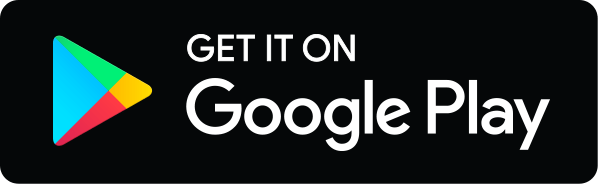