chapter 13 Pharmacology, Anatomy, and Physiology
NITROUS OXIDE
Preparation
The gas is then chemically scrubbed to remove any alkaline and acid substances and is then compressed in stages so that the less easily liquefied gases, such as nitrogen (N2) and oxygen (O2), are separated out. Finally, it is compressed and stored in metal cylinders, in which approximately 30% of the N2O in the full cylinder is liquefied. According to the U.S. Pharmacopeia, N2O must be 97% pure; however, with the manufacturing processes in use today, the gas usually approaches a purity of 99.5% (Figure 13-1).1
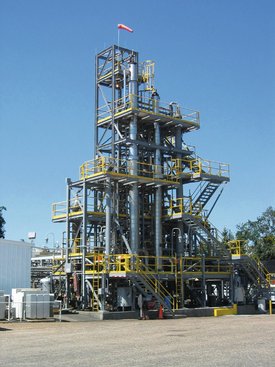
Figure 13-1 A typical manufacturing plant in which N2O is prepared.
(Courtesy Airgas Nitrous Oxide Corp.)
As prepared, N2O is anhydrous. The absence of water in the gas is of importance because water vapor would freeze as it passes through the reducing valve (see Chapter 14), leading to a drop in the gas pressure.
Properties
Solubility
When an anesthetic gas is first inspired, blood entering the alveolus by the pulmonary artery contains none of it. When reaching the pulmonary capillary, the blood is suddenly exposed to the tension of the gas present in the alveolus. If the gas is totally insoluble in the blood (blood-gas partition coefficient of 0), none of the agent will be taken up by the circulation and the alveolar concentration will rise rapidly and will soon equal the inspired concentration (Figure 13-2).
If, on the other hand, the anesthetic is slightly soluble in blood, only small quantities will be carried by the bloodstream. Alveolar concentration will again rise rapidly (Figure 13-3).
N2O and desflurane are examples of anesthetic gases with low blood solubility (Table 13-1). On inhalation, these gases rapidly diffuse across the alveolar membrane into the blood. Because of their poor blood solubility, only a small quantity is absorbed and the alveolar tension rises rapidly so that the tension of the gas in the blood is also increased quickly (see Figure 13-3). Because of the rich cerebral blood supply, the tension of these gases within the brain also rises rapidly and the onset of clinical actions is quickly apparent. Likewise, the rate of recovery from sedation or anesthesia produced by these gases is equally rapid once delivery of the anesthetic ceases.2,3
Table 13-1 Blood-Gas Partition Coefficients of Inhalation Anesthetics
Agent | Blood-Gas Solubility Coefficient |
---|---|
Desflurane | 0.42 |
Nitrous oxide | 0.47 |
Sevoflurane | 0.068 |
Isoflurane | 1.40 |
Enflurane | 1.91 |
Halothane | 2.36 |
Chloroform | 10.30 |
Diethyl ether | 12.10 |
Methoxyflurane | 13.00 |
Conversely, gases with high blood solubility require longer periods of time for the onset of action to develop. Large volumes of the gas are absorbed by the blood (as by a piece of absorbent paper) so that the alveolar tension rises quite slowly (Figure 13-4). Tension of the gas within the blood also rises slowly in this case, and the induction of sedation or anesthesia is noticeably slower, as is the return to the preanesthetic state after termination of drug administration.
Potency
N2O is the least potent of the anesthetic gases; however, it remains the most frequently administered inhalation anesthetic. At one time, it was thought that any anesthetic effects of N2O were a result of the exclusion of O2 from cells in the brain because N2O is 35 times more soluble in plasma than N2 and 100 times more soluble than O2.4 It has since been demonstrated that N2O can, in the presence of adequate O2, produce CNS depression. Guedel classification stage II anesthesia (delirium) is often produced if the patient is not monitored properly.
With a MAC (the minimal alveolar concentration of anesthetic that prevents movement in 50% of subjects in response to a standard surgical incision) of 105%, N2O is unable to produce adequate anesthesia unless it is administered under hyperbaric conditions. More realistically, surgical-depth anesthesia is usually not obtainable unless a more potent inhalation or intravenous (IV) anesthetic is combined with N2O. Such IV agents include the barbiturates, propofol, and opioids, and inhalation anesthetics include sevoflurane, isoflurane, and desflurane. These are discussed in Section VI.
That N2O in subanesthetic doses produces analgesia, a change in the patient’s perception of pain, is no longer doubted. It is estimated that a 20% : 80% mixture of N2O-O2 produces the analgesic effectiveness of 10 to 15 mg of morphine.5 However, biologic variability can significantly alter these figures in individual patients.
Pharmacology
After N2O is inspired through the mouth and/or nose, the gas is transported through the respiratory tract into alveolar sacs, where it is rapidly absorbed into the pulmonary circulation. Because of the high inspired concentration of N2O and the large gradient of N2O between the alveolar sacs and the blood, up to 1000 ml of N2O may be absorbed every minute. N2O replaces N2 in the blood; the N2 is eliminated as the N2O-O2 mixture is inhaled. Because N2O is 35 times as soluble in the blood as the N2 it replaces, large volumes of N2O may be absorbed over prolonged periods of administration.4,6
Potential changes may occur within air-filled body cavities during the administration of N2O because of the extent of its absorption. During the induction of N2O-O2 sedation or anesthesia and during long procedures, N2O enters a closed air-filled space 35 times more rapidly than N2 leaves the cavity. This produces an increase in the pressure or volume of that cavity or space. Specific examples of this include increased intestinal distention if bowel obstruction is present, increased pressure in the pleural space aggravating a pneumothorax, and expansion of the middle ear airspace to the point of actually displacing a tympanoplasty graft.7
Because of its rapid uptake, two interesting phenomena—the so-called concentration effect and the second-gas effect—are seen when N2O is administered. The concentration effect occurs when high concentrations of a gas are administered. The higher the concentration of the gas inhaled, the more rapidly the arterial tension of the gas increases. For example, a patient receiving N2O-O2 in a ratio of 75% : 25% will absorb up to 1000 ml/min of N2O during the initial stages of induction. As the volume of N2O is removed from the lungs into the blood, fresh gas is literally sucked up into the lung from the anesthesia machine, thereby increasing the rate at which the N2O arterial tension increases. If, however, a patient receives only 10% N2O (a figure more appropriate in dentistry than 75%), the uptake of N2O by the blood will be only 150 ml/min, which results in no significant change in the rate at which the agent is absorbed or the rate of rise of N2O arterial tension.8
The second-gas effect occurs when a second inhalation anesthetic is administered along with N2O-O2. The second-gas effect is also related to the rapid uptake of as much as 1000 ml/min of N2O-O2 during the induction of anesthesia. Because of the extremely rapid uptake of a large volume of N2O, a form of vacuum develops in the alveoli that forces even more fresh gas (N2O-O2 plus other inhalation anesthetics) into the lungs. For example, if halothane (1%) is administered along with N2O-O2 in a ratio of 75% : 25%, its uptake will be more rapid than predicted. This is the second-gas effect.9
N2O is absorbed rapidly from the alveolar sacs into the pulmonary circulation. Primary saturation of the blood and brain with N2O is accomplished by the displacement of N2 from the alveoli and the blood and occurs within 3 to 5 minutes of the onset of N2O-O2 administration.10 Clinically, this is significant because the patient should be permitted to remain (ideally) at a given level of N2O for 3 to 5 minutes before the inspired N2O concentration is increased. This permits the full clinical effect of the given concentration of N2O to develop before additional gas is added. In actual clinical practice, the 3- to 5-minute wait is not necessary. A thorough discussion is presented in Chapter 15. Tissues with a greater blood flow, including the brain, heart, liver, and kidneys, will receive more N2O and consequently absorb greater volumes of the gas. The remaining tissues, with a relatively poor blood supply, fat, muscle, and connective tissues, absorb only a small portion of N2O until primary saturation is completed. At this time, these tissues play a major role in N2O absorption. Because the uptake and absorption of N2O by these tissues is slow (denitrogenation may require 6 to 7 hours), there is no reservoir of N2O present in them to impede recovery when N2O delivery is terminated.
For years it was believed that N2O did not undergo biotransformation in the body. However, it is demonstrated that anaerobic bacteria in the bowel metabolize N2O through a reductive pathway with the production of free radicals. There is no convincing evidence that these free radicals cause any specific organ damage.11 Despite this, the vast majority of inhaled N2O is exhaled through the lungs within 3 to 5 minutes after termination of its delivery. Approximately 1% of the inhaled N2O will be eliminated more slowly (over 24 hours) through the lungs and skin.12
At the completion of the procedure, the N2O flow is terminated. N2O diffuses out of the blood and into the alveoli as rapidly as it diffused into the blood during induction. If the patient is allowed to breathe atmospheric air at this time, a phenomenon known as diffusion hypoxia (and the Fick principle) may develop.13 Diffusion hypoxia is responsible for most reports of headache, nausea, and lethargy occurring after N2O administration—a hangover effect. The alveoli of the patient breathing atmospheric air become filled with a mixture of N2, O2, CO2, water vapor, and N2O. During the first few minutes the patient breathes atmospheric air, large volumes of N2O diffuse through the blood into the lungs and are exhaled. As much as 1500 ml of N2O may be exhaled in the first minute by a patient having breathed N2O-O2 in a ratio of 75% : 25%. This figure falls to 1200 ml in the second minute and 1000 ml in the third. The concentration effect, discussed previously, is now reversed, and gases rush out of the lungs. More CO2 is removed from the blood than usual because of this effect, lowering the CO2 tension of the blood. Decreased CO2 tension of the blood reduces the stimulus for breathing and produces a depression of respiration.
The adverse effects of diffusion hypoxia may be prevented through the routine administration of 100% O2 for a minimum of 3 to 5 minutes at the termination of the procedure.14 After N2O-O2 inhalation sedation as usually employed in dentistry, diffusion hypoxia is unlikely to develop, and when it does, it is usually clinically insignificant.
Recovery from the effects of N2O is usually rapid and complete. If in the opinion of the drug administrator the patient has fully recovered, the patient may be permitted to leave the office unescorted, to drive his or her motor vehicle, and to return to normal activities with no prohibitions. This vitally important aspect of N2O-O2 sedation is discussed thoroughly in Chapter 15.
Central Nervous System
The actual mechanism of action of N2O is unknown, but almost all forms of sensation are depressed (sight, hearing, touch, and pain). Memory is affected to a minimal degree, as is the ability to concentrate or perform acts requiring intelligence.15 When administered in conjunction with physiologic levels of O2 (greater than 20%), N2O produces a mild depression of the CNS, primarily the cerebral cortex. At therapeutic levels, N2O does not exert any other actions on the CNS. The area postrema (the vomiting center) of the medulla is not affected by N2O unless hypoxia or anoxia is present. Nausea and vomiting occurring after the administration of N2O are uncommon in the absence of anoxia or hypoxia.16
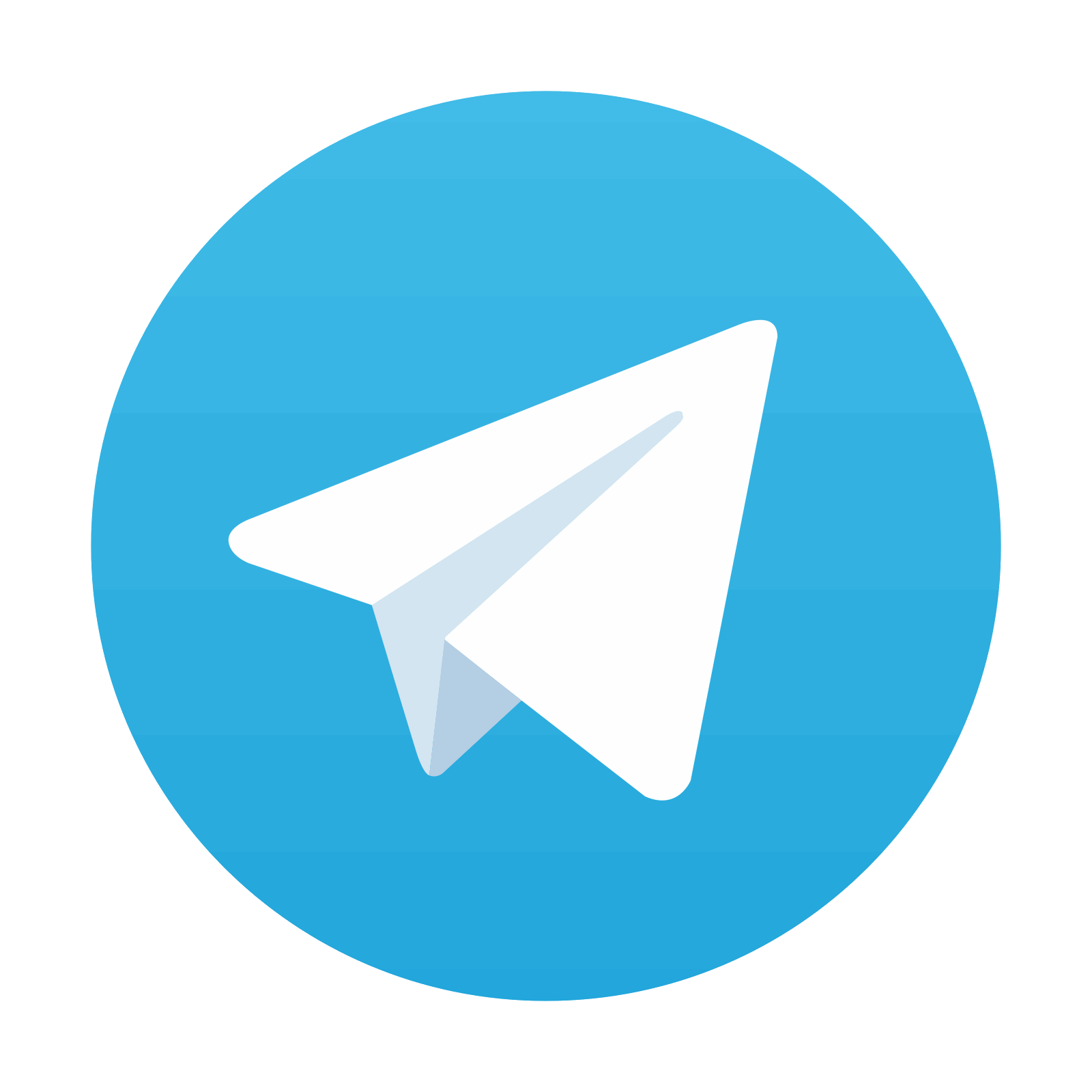
Stay updated, free dental videos. Join our Telegram channel

VIDEdental - Online dental courses
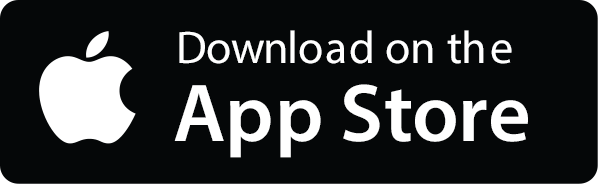
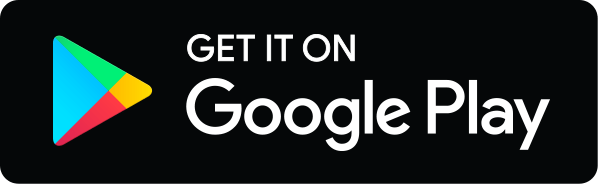