13
Obstructive Sleep Apnea: Orthodontic Strategies to Establish and Maintain a Patent Airway
Summary
Obstructive sleep apnea (OSA) is a significant chronic condition that can occur in young and old patients. The etiology is complex and multifactorial in nature. Dental, medical, and surgical practitioners all have a role to play in the diagnosis and treatment planning of OSA. The responsibility of the orthodontist is to recognize patients, either at risk of or those who may already have OSA. The diagnosis and appropriate modalities of treatment are established in consultation with medical and surgical colleagues. Although the gold standard treatment of continuous positive airway pressure (CPAP) can be applied to both groups of patients, compliance with its long-term use can be challenging. Besides CPAP, there are other OSA treatment modalities that can be considered. In growing children, dentofacial orthopedic treatment for maxillary expansion and mandibular growth, in conjunction with otolaryngological management of hyperplastic lymphoid tissues, may alleviate or cure apnea. The orthodontist should always consider pre-existing narrow, posterior airway space in adult patients so that orthodontic and orthognathic treatment does not further embarrass a compromised airway. Mandibular advancement splints are efficacious in relieving mild to moderately severe OSA. Long-term follow-up is a necessary part of the interdisciplinary care of OSA patients and involves review and management of medical comorbidities and obesity.
Introduction
Apnea is derived from the Greek words, a (without), and pnoia (wind) (Lechner, 1990). There are three distinct types of sleep apnea: central, obstructive, and complex, with an estimated frequency of 0.4%, 84%, and 15%, respectively. Complex apnea is a combination of apneas with a transition of features from central to obstructive apnea (Morgenthaler et al., 2006).
Obstructive sleep apnea (OSA) is defined as interrupted airflow despite persistent respiratory effort. It occurs several times every hour during sleep. Breathing continues but the airflow is blocked. This is due to the complete or partial collapse, and/or complete or partial obstructions, of the upper airway during sleep but not during wakefulness. With reduced airflow, gaseous exchange is impaired. Sleep is fragmented due to recurrent arousals (American Academy of Sleep Medicine, 2005).
OSA is a common sleep-breathing disorder. It affects approximately 2–4% of the middle-aged American population; men are twice as likely as women to have OSA. Among habitual snorers between the ages of 30 and 60 years, approximately 25% of men and 10% of women have OSA. The gender difference diminishes with age, and by 50 years of age, the incidence of OSA is similar in both sexes.
Body mass index (BMI) is an independent factor that correlates with severe OSA. In the morbidly obese, the incidence of OSA increases by at least 12–30 times (Young et al., 1993; Kyzer and Charuzi, 1998; Resta et al., 2001).Weight gain and obesity are important factors in the development and progression of OSA in middle-aged patients. The World Health Organization (WHO) recommends cut-off points to indicate obesity at BMI ≥30 kg/m2 for Caucasian adults and ≥28 kg/m2 for Asian adults; Asians have higher body fat percentage at a lower BMI (WHO Expert Consultation, 2004). Other than age, gender, and obesity, general characteristics of OSA patients are low waist-hip ratio and high serum cholesterol concentration (Tishler et al., 2003).
The estimated prevalence of pediatric OSA is 1–3%, affecting children at around 2–6 years of age with no gender predilection (Rosen et al., 2004). Among the morbidly obese children between the ages of 6 and 19 years, OSA prevalence increases to 55% (Chay et al., 2000). There is evidence linking OSA with cardiovascular and metabolic diseases, affecting not only children with OSA, but also in children with primary or habitual snoring (Ali et al., 1993; Brunetti et al., 2001; Ferreira et al., 2000; Gozal and Pope, 2001; Chervin et al., 2002, 2006; Chng et al., 2004; Gottlieb et al., 2004; Kennedy et al., 2004; Montgomery-Downs and Gozal, 2006). Approximately 6–11% of children in Asian and Caucasian populations snore habitually. In a Caucasian group of 3–6-year-olds who were habitual snorers, 13% had OSA, whereas in an Asian population of 7-year-olds who were habitual snorers, 9% developed OSA after 3 years. In most children, habitual or primary snoring does not progress to OSA and resolves spontaneously (Castronovo et al., 2003; Anuntaseree et al., 2005).
Regardless of the type of sleep apnea, the patient is usually unaware of the problem. Commonly, symptoms may be present for years without recognition, and over time, the patient may become accustomed to daytime sleepiness related to respiratory-related sleep disturbances at night. Sleep apnea is more often than not reported by bed-partners who witness the patient’s sleep-breathing problems or there may be suspicion of OSA when sequelae of chronic OSA are noticed by the patient or physician.
There is still much to understand about the etiology, pathogenesis, and progression of OSA, despite decades of studies into the nature of the condition and ability to view the awake and asleep airway tract with modern imaging technologies. Clinicians face many, as yet unanswered, questions:
- What is the relationship between OSA in childhood and later on in life? Does childhood OSA progress to adult OSA?
- What are the risk factors and how much time does it take for childhood OSA to progress to adult OSA?
- Does a small-sized pharyngeal airway and mandible predispose to OSA?
- Why do age-related changes in anatomy and muscle physiology lead to development of OSA in some people but not in others?
- What is best practice in orthodontic and orthognathic treatment with regard to OSA patients with malocclusions?
The Spectrum of Obstructive Sleep-Disordered Breathing
The signs and symptoms of what we now know as the sleep-disordered breathing spectrum were recognised many centuries ago. They were described in literary works by William Shakespeare and Charles Dickens, in the seventeenth and nineteenth century, respectively. In Shakespeare’s last play, The Tempest, Sebastian says to Antonio as they circle the sleeping bodies, ‘Thou dost snore distinctly; there is meaning in thy snores’ (Shakespeare circa 1611). In his first novel, the Posthumous Papers of the Pickwick Club, Dickens (1837) described one of his characters, Joe, as ‘a wonderfully fat boy – habited as a serving lad, standing upright on a mat, with his eyes closed as if in sleep’. Obesity-hypoventilation or Pickwickian Syndrome, named with reference to fat Joe, was medically recognised in the 1950’s as severe obesity associated with hypoxia from OSA, hypercapnia from hypoventilation leading to excessive daytime sleepiness, fatigue and heart failure.
OSA is but one entity in a wide-ranging SDB spectrum of conditions with variable severity and overlapping symptoms (Figure 13.1). There are different grades of OSA; simple snoring without arousals or symptoms is at the lower end of the spectrum, whereas upper airway resistance syndrome (UARS) is considered more severe and is associated with multiple respiratory effort-related arousals (RERAs). Sleep hypopnea syndrome is characterized by snoring with symptoms increasing in severity but with the absence of apneas. Mild, moderate, and severe OSA increases with severity in the continuum. At the extreme end of the spectrum is the obesity-hypoventilation syndrome or the Pickwickian syndrome. The Pickwickian syndrome is classified as an extreme form of OSA and is associated with severe morbidity and very high mortality (Burwell et al., 1994; American Academy of Sleep Medicine Task, 1999; Moore, 2000).
Figure 13.1 Spectrum of sleep-disordered breathing: UARS-upper airway resistance syndrome; SHS-sleep hypopnea syndrome; OSA-obstructive sleep apnea.
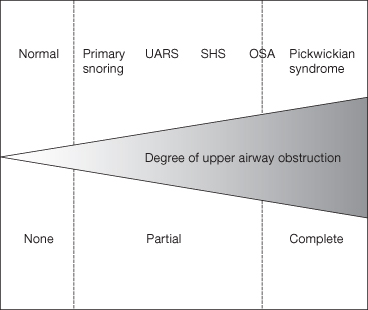
Decoding OSA
A thorough physical examination of the patient with OSA is necessary to establish an accurate medical status. Coexistence of a wide range of medical conditions such as obesity, hypertension, cardiac dysfunction, chronic obstructive pulmonary disease, restrictive lung diseases, metabolic disease, muscular dystrophy, kyphoscoliosis, hypothyroidism, and pituitary tumors highlights the importance of interactive collaboration in the overall management of a patient with OSA (Davila, 1995; Mathur and Douglas, 1995).
The gold standard test for confirmation of OSA is an attended overnight polysomnography (PSG). This is a hospital-based test that records specific physiological parameters during sleep, which enables the diagnosis of OSA and other types of sleep disorders to be made. The total number of respiratory events in an hour is expressed as the apnea-hypopnea index (AHI). AHI is derived from the sum of apneas and hypopneas in an hour of sleep.
An adult apneic event is defined as complete cessation of airflow, for 10 seconds or more, despite persistent respiratory effort. Hypopnea occurs when airflow reduces, by 30% or more, in a partially obstructed airway. Each episode of reduced airflow is significant if it lasts for at least 10 seconds’ with an arterial oxygen desaturation of 4% or more; or if there is a 50% or greater reduction in airflow for at least 10 seconds associated with an arousal due to hypercapnia. The criterion of apneas and hypopneas of 10 seconds duration is based on two lost breaths during normal adult respiration rates. Five to 15 apnea and/or hypopnea events per hour is classified as mild OSA, more than 15 and up to 30 events per hour is moderately severe OSA, and more than 30 events per hour is classified as severe OSA (Guilleminault et al., 1978; American Academy of Sleep Medicine Task Force, 1999).
The criteria for defining pediatric OSA differ from those of adult OSA (Table 13.1). In children, OSA consists of shorter apnea and hypopnea periods associated with pediatric respiration rates. An apneic event lasts for the duration of two lost breaths in children, about 6 seconds. Hypopnea in children refers to a drop in airflow by at least 50% and associated with an arousal or an arousal with 3% desaturation of arterial oxygen. An AHI of 1–5 events per hour is indicates very mild OSA, 5–10 events per hour is mild OSA, 10–20 events per hour is moderately severe OSA, and greater than 20 events per hour is severe OSA in children (American Academy of Sleep Medicine, 2005).
Table 13.1 Comparisons of childhood and adult obstructive sleep apnea
Parameter | Child | Adult |
Peak age | 2–6 years | 30–60 years |
Gender ratio (female : male) | 1 : 1 | 1 : 2 |
Apnea–hypopnea index | ≥1 | ≥5 |
Estimated prevalence | ||
– In the general population | 1–3% | 2–4% |
– In habitual snorers | 9% | 10–40% |
– In morbidly obese | 37–55% | >50% |
BMI | Failure to thrive or ≥98th percentile | ≥28 Asian; ≥30 Caucasian |
Common cause | Adenotonsillar hypertrophy | Obesity |
Polysomnographic values of note are the AHI and the apnea index (AI) in grading the severity of OSA. Based on positive clinical findings, even if the AHI value is low, a diagnosis of OSA with an AHI of 1 is considered to be significant in children. Clinical information is as important as AHI and AI values in determining the clinical severity and morbidity of pediatric sleep-disordered breathing (Marcus et al., 1992; American Thoracic Society, 1999; Chan et al., 2004). Affected children demonstrate behavioral problems and learning problems, hyperactivity, inattention, aggression, and neurocognitive deficits with impact on memory, learning, and executive functions. Research suggests that neurocognitive deficits are partially reversible but there may be a residual learning deficit representing a ‘learning debt’ (Gozal and Pope, 2001; Larkin et al., 2005). Children with AHI ≥5 have raised levels of C-reactive protein (CRP), which is known to increase the risk of cardiovascular disease. Children with OSA may also have autonomic dysfunction with increased sympathetic activity (Gozal and O’Brien, 2004). There is also evidence of systemic inflammation in OSA patients (AM Li et al., 2008). The inflammatory mediator cysteinyl leukotriene (cys-LT) has been found in exhaled breath condensates of children and is related to the severity of SDB (Goldhart et al., 2006).
OSA is complex in children and cannot be excluded on the basis of history and physical examination (Gottlieb and Young, 2009). A diagnosis of OSA based on history and physical examination has a sensitivity of 63%, and a specificity of 60%. All the studies conducted on the predictive value of the history and the clinical signs and symptoms indicate that there is no foolproof method for confirming OSA except by attended overnight polysomnography (Hoffstein and Szalai, 1993).
If there is a high index of clinical suspicion, the patient should be referred to a doctor trained to manage sleep disorders or a sleep disorders team for examination of all possible causes and confirmation of the diagnosis. Full night (type 1) polysomnography is the gold standard test and is recommended for the diagnosis of OSA both in adults and in children (Kushida et al., 2005).
Respiration: Effect of Anatomy and Sleep
Effect of Anatomy on Respiration
The upper airway tract comprises three components: the nose, the pharynx, and the larynx. The nose, portal to the upper airway, is divided into two openings by the nasal septum; there is an alternative route should one nostril be blocked. The nasal airway is the preferred route for resting ventilation and infants are obligatory nasal breathers (Hall et al., 2000). The structures maintaining the patency of the anterior nares are the alar, procerus, compressor, and dilator naris muscles. The anterior nasal valves are responsible for much of the nasal resistance in patients with normal nasal apertures. The nose contributes to up to half of the total airway resistance in the normal awake patient (Ferris et al., 1964). The lateral nasal walls contain several protuberances, the nasal turbinates, which serve the following functions: creation of air turbulence to slow down airflow so as to prolong air contact with the highly vascularized nasal mucosa for temperature and humidity control; it contains nasal secretions with antimicrobial actions on inhaled air before it is channeled into the lungs. With increased ventilatory drives to meet physiological demands, the nasal apertures widen and nasal mucosa constricts to reduce airflow resistance. At a physiological threshold of ventilatory demand, the mouth opens for oral breathing to increase volume per breath, bypassing nasal resistance.
Inspired air passes through the nasal airway to the pharynx via the bilateral choanae. The pharynx traverses three regions: the nasopharynx which extends from the posterior nasal choanae to the hard palate; the oropharynx which comprises the retropalatal and retroglossal segments – the former extends from the level of the hard palate to the caudal margin of the soft palate and tip of the uvula, and the latter from the soft palate margins to the base of the epiglottis; the laryngopharynx, which is the segment from the base of the tongue to the larynx. Narrowing of the posterior nasal choanae occurs in patients with craniofacial abnormalities. Pharyngeal airway narrowing or collapse is common at the retropalatal and the retroglossal aspects of the pharynx, with a majority of patients having more than one site of obstruction (Hudgel, 1988; Horner et al., 1989; Launois et al., 1993; Morrison et al., 1993; Suto et al., 1993; Trudo et al., 1998; Ciscar et al., 2001).
Nasendoscopic studies describe the mechanics of pharyngeal airway narrowing as a function of sphincteric movement of the lateral pharyngeal walls together with backward movement of the anterior structures. Posterior pharyngeal wall movement is not implicated in airway narrowing (Weitzman et al., 1978).
The larynx connects the pharynx with the lower airway, the trachea. It is responsible for phonation as well as protection of the trachea. It is bounded superiorly by the epiglottis, inferiorly by the vocal chords, and laterally by the aryepiglottic folds. These structures work in synchrony to channel air into the anterior tract, the trachea, whereas food and liquids are directed into the posterior tract, the esophagus. The epiglottis acts as a valve to separate the trachea from the esophagus during deglutition and to maintain a patent airway during respiration. The posterior cricoarytenoid is the only abductor muscle that widens the glottic aperture during inspiration and expiration. The tongue and lingual tonsils, which stud the dorsum of the posterior third of the tongue, may hinder the function of the epiglottis if the lingual tissues are enlarged and encroach into the posterior airway space (Suzuki and Kirchner, 1969; Wyke, 1974).
Redundant mucosa in the arytenoid-aryepiglottic region has been associated with OSA. Vocal chord abnormalities are rarely implicated in symptomatic OSA, however, bilateral vocal chord paralysis has been reported to be associated with snoring. Rarely, congenital vallecula cysts occur between the base of the tongue and the epiglottis. When cysts are present in this region or in the larynx, they are sometimes associated with airway obstruction, stridor, pyoceles, or vocal chord paralysis (Griffith et al., 1993).
Many cephalometric studies have shown an association between certain craniofacial characteristics and OSA. These include hard and soft tissue features such as reduced mandibular body length (Miles et al., 1996), posteriorly positioned maxilla, and inferiorly positioned hyoid bone (deBerry-Borowiecki et al., 1988; Bacon et al., 1990; Pracharktam et al., 1994; Lowe et al., 1995). Other distinguishing craniofacial features of OSA patients are reduced cranial base length, mandibular or maxillomandibular retrusion, increased lower face height, an elongated soft palate, large tongue and a smaller upper airway compared with normal individuals (Horner et al., 1989; Ciscar et al., 2001).
The oropharyngeal airway is usually narrowed (Shepard and Thawley, 1989; Schwab et al., 1993, 2003) in OSA. Reduced size of the posterior airway space and increased distance of the hyoid bone to the mandible are also associated with OSA. The two cephalometric parameters that are used as indicators of OSA are: posterior airway space of less than 11 mm and a hyoid to mandible distance of greater than 15.4 mm (Riley et al., 1983). Severe respiratory disturbance indices are associated with a posterior airway space of less than 5 mm at the base of the tongue and a distance of the hyoid bone to the lower border of the mandible distance of 24 mm or greater (Partinen et al., 1988). There is low probability of OSA if the airway cross-sectional area is greater than 110 mm2, but a high probability of severe OSA if the area measures less than 52 mm2. Most constrictions are located in the oropharynx (Lowe et al., 1986; Avrahami and Englender, 1995; Ogawa et al., 2007).
There are also differences in the soft tissue characteristics of OSA patients compared with those without OSA; besides a larger tongue, OSA patients have thicker and longer soft palates and parapharyngeal fat pads in the lateral pharyngeal walls (Schwab, 2003; Schwab et al., 2003).
Effects of Sleep on Respiration
The diaphragm is the main driver of ventilation during sleep. Pharyngeal airway patency is well maintained during wakefulness but during sleep, pharyngeal and genioglossal muscle activity is reduced, which predisposes to sleep-dependent pharyngeal airway collapse (McNicholas, 2002; Jordan and White, 2008). Sleep adversely affects tonic and phasic skeletal muscle functions. There is evidence suggesting that in OSA, the intrinsic upper airway muscle function and central neural regulation of the upper airway dilator muscles and genioglossus may be impaired. Recurrent apnea during sleep induces intermittent hypoxia that alters respiratory muscle contraction, reducing muscle endurance with diminished pharyngeal electromyographic (EMG) responses to physiological changes (Bradford et al., 2005).
Sleep depresses muscular contractions, which allows the negative pressure of inspiration to pull the tongue into the pharyngeal airway (Remmers et al., 1978). The mechanical properties of the upper airway, that maintain tissue stiffness and thus airway patency, are reliant on intrinsic physiological properties. In OSA patients, there is reduced effectiveness of upper airway dilator contraction and reduced genioglossal reflex. The genioglossal negative pressure reflex declines with age, which may explain age-related increase in OSA. Current evidence suggests a biomechanical basis for OSA in patients who have an anatomical and sleep-positional predisposition to pharyngeal collapse (White et al., 1985; Sériès, 2002; Malhotra et al., 2004; Dempsey et al., 2010).
Mechanical Influences on Respiration
A number of mechanical influences impact the airway diameter causing it to be open, narrowed, or closed. These factors can be either static or dynamic. Static factors are surface adhesive forces, neck and jaw posture, tracheal tug, gravity, and sleep position. Dynamic factors comprise upstream resistance within the nasal airway and pharynx: the Bernoulli effect and dynamic compliance (Schwab et al., 2005).
Several studies have demonstrated a smaller upper airway width in OSA patients compared with patients without OSA. The reduction in size of the upper airway has been attributed to the enlargement of the surrounding soft tissues: lateral pharyngeal walls, parapharyngeal fat, tongue, and soft palate. Reduced airway diameter affects airway resistance the most, as described by Poiseuille’s law: R = 8nl/pr4; the resistance (R) and the length of the tube (l) are inversely related to its radius (r) and pressure (p). By halving the size of the tube, resistance increases 16 times (Shepard and Thawley, 1989; Schwab et al., 1993; Schwab, 2003; Schwab et al., 2003).
Nasal obstruction has been implicated as a cause of OSA because nasal resistance and mouth breathing increase upper airway collapsibility. Nasal resistance, measured by posterior rhinomanometry, is reported to be higher in individuals with OSA. Nasal resistance increases upper airway pressure during inspiration, placing the pharyngeal walls at a greater risk of collapse (Isono et al., 2004). In infants, during sleep, the genioglossal activity increases when an infant switches from oral to nasal breathing. There is evidence correlating increased genioglossal EMG activity with changes in airway resistance and carbon dioxide levels (Roberts et al., 1986).
Mouth-breathing may also play a role in pharyngeal airway obstruction by changed dynamics in the airway; mouth-opening destabilizes airway patency. When the tongue is lowered, it moves away from the soft palate. Once the soft palate is no longer in contact with the tongue, it becomes free moving and obstructs the pharyngeal airway when it falls backwards. Surface adhesion forces of mucosal surfaces may also be contributory in maintaining airway patency (Issa and Sullivan, 1984; Roberts et al., 1985).
OSA in Children
The clinical morbidties associated with obstructed upper airways were first reported in medical publications more than a century ago. In 1889, Ambroise Guye described ‘the impairment of cerebral functions by disorders of the nose’. The obstructive effect of enlarged adenoid masses, particularly during sleep, was observed to produce ‘aprosexia’ – the inability to fix the attention on any abstract subject. With removal of the adenoid mass from the nasopharynx, headaches ceased and learning difficulties were prevented (Guye, 1889).
This observation was confirmed soon after by William Hill (1889) who was ‘much struck by the fact that operations on children, undertaken for the relief of deafness associated with adenoid growths, enlarged tonsils, and hypertrophic catarrhal conditions of the nose, have frequently resulted in such an immediate improvement in the mental acuteness of the patients as was altogether incommensurate with the often slight immediate improvement in the sense of hearing.’ He advised that the ‘stupid looking lazy child who frequently suffers from headache at school, breathes through his mouth instead of his nose, snores and is restless at night, and wakes up with a dry mouth in the morning, is well worthy of the solicitous attention of the medical officer’.
Despite Hill and Guye’s strikingly similar observations, the controversies over the causality of OSA and what is systematic evidence-based management of children with nasal congestion, adenotonsillar hypertrophy and OSA rage on till today (Bixler et al., 2009). Although OSA in children is largely attributed to adenotonsillar hypertrophy, there is no conclusive evidence to demonstrate a causal relationship between enlarged tonsils and/or adenoids in children with SDB. Nevertheless, magnetic resonance imaging (MRI) has revealed the region of greatest pharyngeal airway narrowing as the area where the adenoid and tonsillar masses overlap (Arens et al., 2003).
Diagnosis
Proper diagnosis forms the basis for effective treatment. All children should be screened for snoring as part of well-child visits. Habitual snoring, if present, should prompt a more detailed history for other symptoms: unusual sleeping postures, restless sleep, labored sleep-breathing, observed apneas, hyperactivity, attention deficit or behavioral problems. Hyperactivity is more likely to be present in the child with OSA rather than excessive daytime sleepiness; the latter occurs more often in adults with OSA (Leach et al., 1992; Carroll, 1996). Craniofacial anomalies and hyotonia in children are positively correlated with OSA and craniofacial morphology attributed to OSA. Down syndrome children, who have hyotonia and obesity, are more likely to develop OSA (approximately 59–79%; Dyken et al., 2003; Ng et al., 2006). Treated cleft lip and palate patients have similar craniofacial, craniocervical, and pharyngeal morphology with OSA patients; narrowing of the oropharyngeal airway at the tip of the velum and a greater distance between the hyoid and the mandibular plane (Oosterkamp et al., 2007).
There is a positive correlation between OSA and obese children without adenotonsillar hypertrophy (Verhulst et al., 2008). In children with adenotonsillar hypertrophy, the greatest narrowing of the airway is seen at the retropalatal region where the soft palate, adenoid, and tonsils overlap (Isono et al., 1998; Fregosi et al., 2003). Significant cephalometric nasopharyngeal airway measurements are as follows:
- Percentage of nasopharynx occupied by the adenoid mass compared to percentage airway norm for a 6-year-old is approximately 51% (Handelman and Osborne, 1976; Schulof, 1978).
- Reduced distance from the posterior nasal spine (PNS) to the nearest adenoid mass measured along PNS-basion; normal distance in a 6-year-old is approximately 15–21 mm (Linder-Aronson, 1970; Schulof, 1978).
- Reduced distance from PNS to the nearest adenoid mass measured along a line through PNS perpendicular to sella-basion; normal measurement in a 6-year-old is approximately 15–16 mm (Schulof, 1978; Linder-Aronson and Leighton, 1983).
- Reduced distance to the nearest adenoid mass from a point on the pterygoid vertical 5 mm above PNS; the normal value is approximately 7 mm (Ricketts, 1954; Schulof, 1978).
Screening for OSA using questionnaires in children with adenotonsillar hypertrophy has been suggested, but it has not been proven to detect the presence or the absence of OSA (Constantin et al., 2010). Adenotonsillar hypertrophy is a common finding in children. The Scammon growth curves demonstrate accelerated growth of the lymphoid tissue from birth, reaching its maximum at 11–13 years. The total body lymphoid tissue of a 13-year-old will have attained a mass of almost 200% greater than that of an adult. Lymphoid tissue growth declines and tails off gradually between 14 and 20 years of age. Craniofacial skeletal growth follows a different timing and growth trajectory; the cranial vault and the upper face follow the neural pattern of growth which peaks between 8 and 12 years of age, with growth ceasing between 12 to 16 years of age. Growth of the lower face and mandible follows the general adolescent growth spurt, peaking between 14 to 18 years, and slows down or stops after 20 years of age (Scammon, 1930).
Selective screening of special groups of children, those with craniofacial syndromes and complex craniofacial synostosis, is effective in those with complex craniosynostosis and have fusion of at least two cranial sutures. In a study of children with Apert, Crouzon, Muenke, Pfeiffer and Saethre–Chotzen craniosynostotic syndromes, the critical clinical question that identified children without OSA was ‘Has the child difficulty with breathing during sleep?’ A negative response excluded clinically significant OSA (high negative predictive value of 91%, sensitivity of 64%). Snoring is a common but less specific symptom as 77% of the children with craniofacial syndromes snored due to narrow noses and midface hypoplasia. Children with craniofacial syndromes or complex craniosynostoses had a 40% chance of developing OSA from midface retrusion (Bannink et al., 2010).
Some studies recommend against the use of history to distinguish the primary snorer from the child with OSA (Carroll et al., 1995; Lamm et al., 1999). Screening pediatric patients for OSA using a combination of history taking, sleep-related symptoms questionnaire and clinical assessment misses the detection of OSA of 1 in 5 children. An unusual sleep posture, as in sitting up to sleep, has been shown to be a significant predictor of a child with OSA. However, overnight polysomnography is still the definitive diagnostic tool for confirming the presence of OSA (Chay et al., 2000).
Certain groups of children should be targeted for OSA screening. The risks of OSA are higher in children with craniofacial anomalies, Down syndrome, cleft lip and palate after pharyngoplasty, morbid obesity, and atopic disease (Guilleminault et al., 1981; Brouillette et al., 1982; Mallory et al., 1989; Marcus et al., 1991; Silvestri et al., 1993; Chng et al., 2004). The index of suspicion for OSA is further increased in children who fail to thrive or are obese and have cardiovascular symptoms. Obesity in a child is defined as having a BMI that is above the 95th percentile in their age and gender cohort. Obesity is a primary independent factor impacting on the respiratory disturbance index (RDI) in children. Children with BMI ≥180 percent of ideal body weight are deemed to be morbidly obese, and between 37–55% of such children have OSA (Brooks et al., 1998; Chay et al., 2000; Mei et al., 2002).
As part of the well-child program, all children should be screened for sleep-breathing problems. Children at risk must be referred for a thorough evaluation and confirmation of OSA by a sleep-trained pediatrician (American Academy of Pediatrics, 2002).
Orthodontic Management
In 1902, Dr Pierre Robin fabricated an ‘apparatus, the monobloc’, the first oral appliance to be used in airway management. He prescribed the monobloc appliance for use in children who had ‘hypotrophy’ of the mandible from as young as 20 months. The treatment was aimed at re-establishing the normal spatial relationship of the maxilla and mandible. Dr Robin also observed that ‘the great majority of children with hypoplasia of the mandible breathed through the mouth’. Most of them, at 6–7 years of age had adenoids, and ‘though the adenoids were removed once or several times they continued to breathe through the mouth and the adenoid facies persisted’.
Dr Robin explained that ‘why a child who has had adenoids removed is still unable to breathe through the nose, since he still suffers from glossoptosis’. He concluded that ‘adenoidism without adenoids’ is a morbid entity requiring general care by a physician and placement of a monobloc in the mouth even after the removal of adenoids. Interdisciplinary management was recognized even then, as necessary, for the treatment of patients with breathing disorders. By using the monobloc to posture the mandible forward, normal mastication is achieved through the ‘perfect equilibration’ of the temporo-maxillodental articulation’ and children with ‘adenoidism’ are able to breathe through the nose (Robin, 1934). The monobloc was later replaced by the activator, which used in the treatment of Class II Division 1 malocclusion. Modified forms have since been used in treatment of patients with OSA (Cozza et al., 2004).
Treatment Involving the Maxilla
Rapid maxillary expansion (RME) or slow maxillary expansion (SME) with maxillary protraction increases the transverse width of the maxilla and enlarges the palatal and retropalatal area. This improves the ratio of airway space relative to tonsil size. Enlargement of the dental arches, transversely and anteroposteriorly increases the intraoral space for accommodating the sublingual tissues and tongue by removing the constraints of narrow maxillary and mandibular arches (Viva et al., 1992; Defabjanis, 2003). RME or SME also enlarges the nasal cavities, and purports to reduce nasal resistance thereby promoting nasal breathing. Villa et al. (2007), reported that the majority of children (78.5%) treated with RME had significantly reduced AHI, with 85.7% of the children switching from oral to nasal breathing.
The findings on outcomes of maxillary expansion with regard to reduced nasal resistance and change to nasal respiratory mode are equivocal. Increased width of the nasal floor is associated with reduced nasal resistance in the short term but was not sustained predictably over the long term. Increased nasal airflow resistance should not be the primary indication for maxillary expansion (Berretin-Felix et al., 2006; Ceroni et al., 2006; Enoki et al., 2006; Neeley et al., 2007). Rhinomanometric studies do not support RME with predictable reduction of nasal resistance and improvement of nasal breathing (Hartgerink et al., 1987). Age and gender may be confounding factors affecting the variability of nasal resistance and nasal respiration; more data is needed to distinguish normal nasal from abnormal nasal functions (Vig, 1998).
Treatment of OSA by Stimulating Maxillary and Mandibular Growth
Correction of anteroposterior maxillomandibular deficiencies in children may improve OSA. Forward repositioning of the mandible with functional appliances are shown to enlarge the upper airway, improving respiratory function after 6 months and reducing AHI in more than 60% of patients (Villa et al., 2002). Results of studies on the effects of maxillary protraction on airway dimensions in children with Class III malocclusion are contradictory. In one study, significant favorable skeletal changes in the maxilla and mandible did not result in short-term or long-term changes in sagittal airway dimensions of the nasopharynx and oropharynx (Baccetti et al., 2010). One other study reported a favorable increase in airway dimensions with maxillary protraction. Maxillary protraction increased upper airway caliber with significant increases in anteroposterior width as well as nasopharyngeal airway area (Oktay and Ulukaya, 2008). The increased airway size was significantly stable after 4 years. The oropharyngeal airway area, however, did not remarkably increase immediately after active treatment but it increased significantly during a 4-year follow-up period (Kaygisiz et al., 2009). The study suggested that the increase in the oropharyngeal airway after active treatment may be an effect of growth.
A randomized controlled study on non-obese 4 to 10-year-old children with OSA (AHI ≥1) reported a normalization of AHI in 50% of patients who used customized oral appliances to advance the mandible for 6 months. The use of oral appliances was associated with reduced tonsillar hypertrophy in about 67% of patients compared with 14% of children in the control group. About 74% of the patients tolerated the appliance treatment well (Villa et al., 2002) (Figure 13.2).
Figure 13.2 (a) Lateral cephalogram showing the significant parameters in a child with mild obstructive sleep apnea. (b) Twin-block appliance to expand the maxilla and correct the retrognathia.
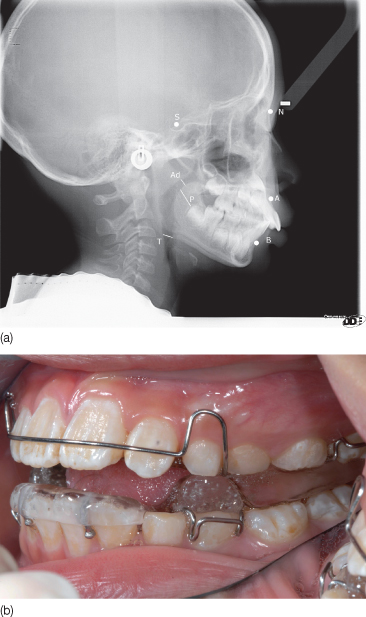
Long-term studies are needed to find out whether dentofacial orthopedic therapy in OSA children is curative in the long term. A review of the effects of oral and functional dentofacial orthopedic appliances indicated that they are an effective nonsurgical alternative in the management of childhood OSA in the short term. However, there is insufficient data to confirm if orthodontic and dentofacial orthopedic treatments are effective in long-term management of OSA (Carvalho et al., 2007).
Children whose OSA does not improve after adenotonsillectomy may have other underlying structural problems: narrow pharyngeal airway, hypoplastic maxilla and/or retrognathic mandible. OSA is also compounded by the coexistence of obesity, neuromuscular disorders, and craniofacial and dentofacial anomalies (Shintani et al., 1998; Chay et al., 2000; Chan et al., 2004).
OSA in Adults
Many OSA patients remain undiagnosed because sleep assessments are not included in medical history taking. A thorough medical history and communication with the patient’s primary physician are important to establish the patient’s general medical condition. OSA is not just confined to obstructive sleep-related breathing; it is also associated with medical comorbidities such as hypertension, congestive heart failure, cardiac arrhythmia, ischemic heart disease, pulmonary hypertension, and metabolic syndrome.
Diagnosis
A thorough history and examination are important tools for identifying adult patients who require further medical evaluation. It is worth while asking the bed-partner whether the patient snores habitually or stops breathing during sleep. Hypersomnolence or excessive daytime sleepiness (EDS) is another common symptom among adults with OSA. EDS screening in adults is easily accomplished with the Epworth Sleepiness Scale (ESS), an eight-item questionnaire. The ESS is a patient-reported assessment of daytime sleepiness and drowsiness in monotonous soporific situations or during drivi/>
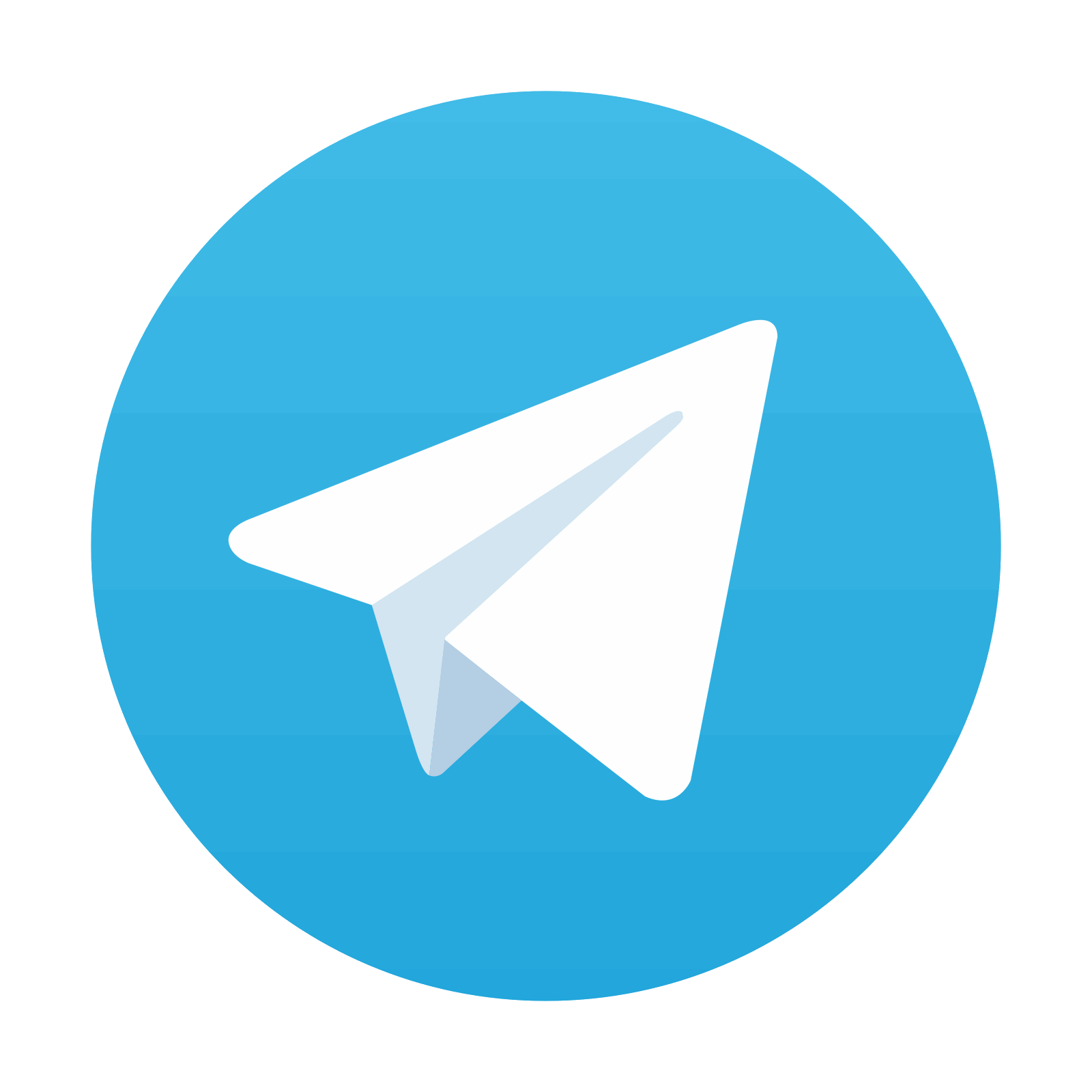
Stay updated, free dental videos. Join our Telegram channel

VIDEdental - Online dental courses
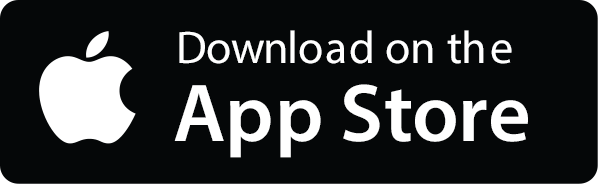
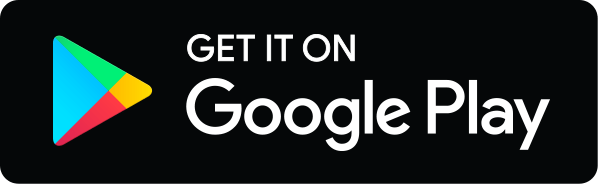