Chapter 12
Orofacial Pain, Touch and Thermosensation, and Sensorimotor Functions
Barry J. Sessle
Faculty of Dentistry, University of Toronto
This chapter reviews the neural processes that are involved in the somatosensory and motor functions of the face and mouth. It focuses first on the neural basis of orofacial touch, temperature, and especially pain common in the orofacial region. In addition, attention is given to the neural mechanisms that underlie the numerous reflex and other sensorimotor functions expressed in the orofacial region, particularly those related to mastication (chewing), swallowing, and other associated neuromuscular functions.
The orofacial region is remarkable in its high level of sensory discriminability and sensitivity, reflecting in part its great innervation density and the large amount of brain tissue devoted to the representation of the oral cavity and surrounding facial areas. Furthermore, there are specialized receptor systems associated with the periodontal tissues that support the teeth and with the facial whiskers (vibrissae) that are especially well developed in several subprimates (e.g., rodents). These receptor systems provide an added dimension of sensory experience, and together with the tongue and lips are very important for an individual’s ability to explore the environment and control movement and other behaviors. Also noteworthy are that some of the most common pains occur in the orofacial region (e.g., toothaches, headaches, temporomandibular disorder (TMD) pain) and can cause considerable long-term suffering. Furthermore, some sensory functions are unique to this region (e.g., taste). The orofacial region also is remarkable in the vast array of sensorimotor behaviors that it manifests, ranging from relatively simple reflexes (e.g., the jaw-opening reflex) to the very complex sensorimotor activities that are associated with speech, mastication, and swallowing that provide for social communication and the ingestion of food and fluid vital for life. These simple or complex sensorimotor activities utilize sensory inputs into the brain from the receptors in the face and mouth to initiate or guide them, and involve the integrated neuronal activity of many parts of the brain.
Sensory functions
Touch
General characteristics
The ability to sense touch (i.e., tactile sensibility) is extremely well developed in the orofacial region of humans and other animals. In fact some species depend on parts of this region for exploring their environment and even for their very survival. For example, the facial whiskers and perioral tissues are crucial for rodents, especially in infancy, to sense their environment and find nutrients (e.g., from their mother’s nipples). In humans, tests to measure touch include tactile threshold, two-point discrimination, and stereognosis (a term referring to the ability to recognize the form of objects), and these tests have shown that some orofacial areas such as the lips and tongue tip have a tactile sensitivity that is better than any other part of the body.
Peripheral processes
As noted in Chapter 9, the orofacial tissues are densely innervated by primary afferent (i.e., sensory) nerve fibers, each of which terminates peripherally at sensory organs termed receptors. These receptors “sense” stimuli applied to the face and mouth as well as changes in the environment, and transduce this sensory information into electrochemical energy, which is then conducted as action potentials along the afferent fibers into the brainstem (i.e., “nerve conduction”). The receptors can be broadly categorized into two types: free nerve endings, and specialized or corpuscular receptors, of which several anatomically distinct examples exist. Many of the receptors primarily associated with myelinated, large-diameter, fast-conducting afferent nerve fibers (A-β afferents, some A-δ afferents) function as mechanoreceptors since they respond either transiently to (as velocity detectors) or throughout (as static-position detectors) a mechanical stimulus applied to a localized orofacial area that is termed the receptive field of the afferent. Figure 12.1 shows examples from recordings of afferents supplying periodontal tissues. Since many types of mechanoreceptors are exclusively activated by tactile stimuli and have an anatomically recognized receptor structure and many neurons in the central relay stations (see below) respond exclusively to tactile stimulation, these features support the concept of the specificity theory of sensation (Fig. 12.2). According to this theory, a specific set of receptors and afferent nerve fibers and neurons and relay stations in the central nervous system (CNS) respond exclusively to tactile stimuli, for example, and provide the higher levels of the brain (e.g., cerebral cortex) only with neural information related to touch and not, for example, to pain or temperature. As noted below, this theory has not held up well in accounting for pain.
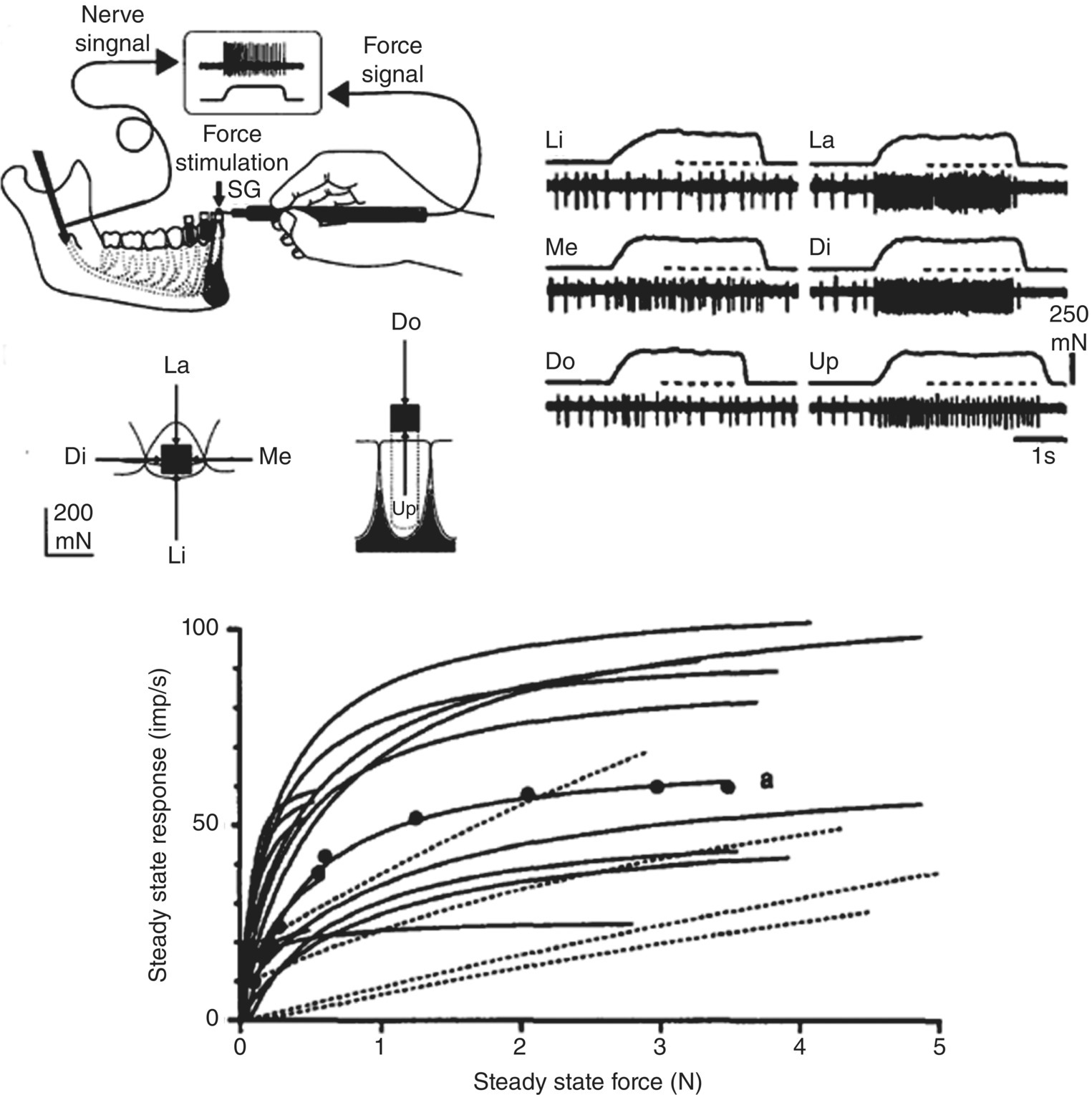
Figure 12.1 Response properties of periodontal mechanosensory afferents recorded in the inferior alveolar nerve in humans during application of forces applied to the central incisor in labial (La), lingual (Li), mesial (Me), distal (Di), downward (Do), and upward (Up) directions. Note the different responses of a single periodontal afferent supplying the tooth when the same magnitude of force is applied in the six different directions; the greatest slowly adapting discharge of the afferent is to the Di force. Also note from their stimulus-response functions that some periodontal afferents show a progressive increase in their response as the force is progressively increased (dotted lines) whereas others show a more rapid increase at low forces but then quickly saturate (adapt).
(From Trulsson and Johansson (1996) Progress in Neurobiology, 49, 267–284).
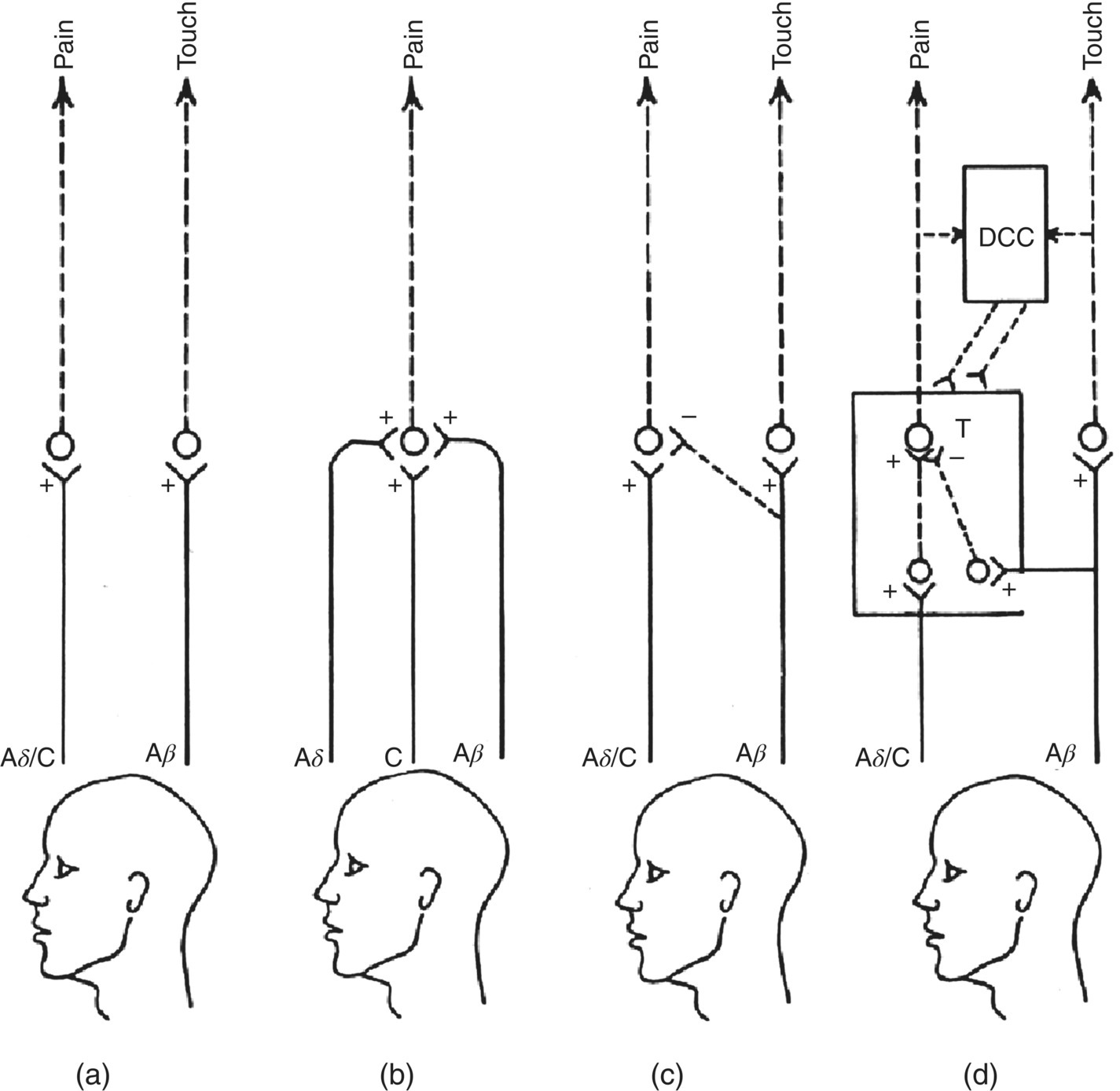
Figure 12.2 The main theories of pain. (a) Specificity theory emphasizes a separate and exclusive peripheral and central pathway for pain compared with, for example, touch. (b) Intensive or summation theory draws attention to the convergence of different afferent fiber types that are activated by different types of stimuli (e.g., noxious, tactile, warm, cool) and that excite (+) central neurons. Pain is coded by a distinctive pattern of activity of central neurons that is evoked by each of the four different stimulus modalities. (c) Sensory interaction theory emphasizes the ability of some afferent fiber inputs (e.g., mechanosensitive) to suppress (–) activity in central neurons relaying nociceptive information. (d) Gate control theory also stresses central interaction of inputs from different afferent fiber types. The interaction of inputs from large-diameter (e.g., A-β mechanosensitive) afferents versus small-diameter (e.g., A-δ and C nociceptive) afferents to central transmission (T) nociceptive neurons is emphasized. This theory also notes the capability of this “gating” mechanism to be modulated (+, –) by descending central controls (DCC).
(Modified from Sessle, 1981a.)
The mechanoreceptors in the facial skin, oral mucosa, periodontal tissues, and jaw joint (the temporomandibular joint, TMJ) thus provide the sensory inputs into the brain that reflect detailed information of the quality, location, intensity, duration, and rate of movement of an orofacial mechanical stimulus. Some analogous receptor mechanisms account for our ability to detect and discriminate the size of small objects placed between the teeth, their hardness and texture, and bite force. These receptors are located in the periodontal tissues around the root of each tooth, but also receptors in the TMJ and even in jaw muscles make an important contribution. Receptors in the TMJ and the jaw muscles also largely account for our conscious perception of jaw position (mandibular kinesthesia).
Central pathways and processes
The major nerve carrying the primary afferent neural signals from the orofacial mechanoreceptors is the trigeminal nerve (the fifth cranial nerve). The low-threshold mechanosensory afferent nerve fibers in the trigeminal nerve pass via the trigeminal ganglion (where their primary afferent cell bodies are located) and the trigeminal sensory nerve root into the trigeminal brainstem sensory nuclear complex (Fig. 12.3). The neural signals are transferred (via synaptic transmission) to mechanosensitive neurons at all levels of the trigeminal brainstem sensory nuclear complex, which can be subdivided into a main sensory nucleus and a spinal tract nucleus; the latter is subdivided further into the subnuclei oralis, interpolaris, and caudalis. These second-order neurons conduct the signals onwards through their projections to higher sensorimotor centers but also many of the neurons project to local brainstem regions, including those responsible for activating muscles, and thereby serve as interneurons involved in reflexes or more complex sensorimotor behaviors (see “Sensorimotor Functions and Motor Control” below). Nonetheless, a major projection from trigeminal brainstem neurons is concerned with touch perception, and this pathway projects from the trigeminal spinal tract nucleus and especially the main sensory nucleus to the ventroposterior thalamus (termed the ventrobasal thalamus in subprimates). This projection is principally to the contralateral side of the brain, but an ipsilateral projection also exists (Fig. 12.3). In the thalamus the signals are relayed to third-order mechanosensitive neurons, many of which project to parts of the overlying cerebral cortex. One part is the somatosensory cortex, where the cerebral cortical neural processing begins that eventually leads to the perception of an orofacial touch stimulus. An extensive and disproportionate part of this somatosensory cortex is devoted to the representation of the face and mouth, reflecting the importance of sensory information from orofacial tissues compared to other body regions.
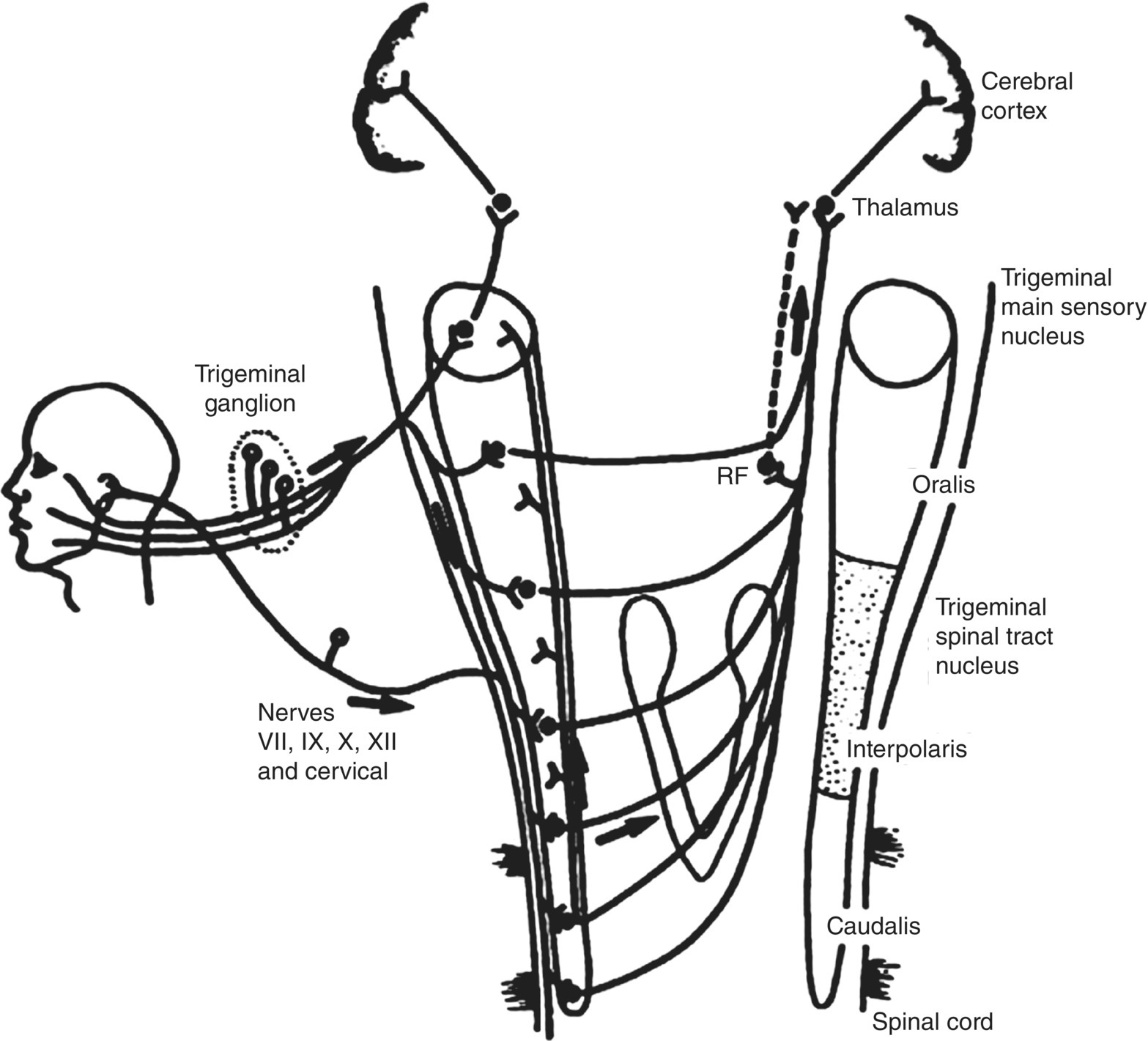
Figure 12.3 Major somatosensory pathways from the orofacial region. Trigeminal primary afferents have their cell bodies in the trigeminal ganglion and project to second-order neurons in the trigeminal brainstem sensory nuclear complex. These second-order neurons may project to neurons in higher levels of the brain (e.g., in the thalamus) or in brainstem regions such as cranial nerve motor nuclei or the reticular formation (RF). The sensory inputs also include orofacial afferents supplying the cornea and sinuses (e.g., maxillary sinus). Not shown in detail are the projections of some cervical nerve afferents and cranial nerve VII, IX, X, and XII afferents to the trigeminal brainstem complex and the projection of many cranial nerve V, VII, IX, and X afferents to the solitary tract nucleus.
(Modified from Sessle, 1981b.)
The second-, third-, and fourth-order neurons in this ascending pathway to the cerebral cortex show many functional properties comparable with those of the mechanoreceptive primary afferent fibers, with a localized mechanoreceptive field and coding of the intensity of the stimulus that results in their activation (e.g., see Fig. 12.4a). They also retain much of the “specificity” of the mechanoreceptors and their mechanosensory primary afferents, thus providing further support for the specificity theory of touch. Nonetheless, by means of the complex ultrastructure and regulatory processes that exist at each of the relay sites, considerable modification of the synaptic transmission of the tactile-related signals can occur, as a result of other incoming sensory signals and descending influences from higher brain centers exerted on touch-transmission neurons in the relay sites (Fig. 12.5). Such modulatory processes may explain, for example, how distraction or focusing one’s attention on a particular task at hand can depress our awareness of a touch stimulus, e.g., being unaware of all the tactile stimulation of the mechanoreceptors on our forearm and other parts of our body when we are writing a letter or examination or reading a book.
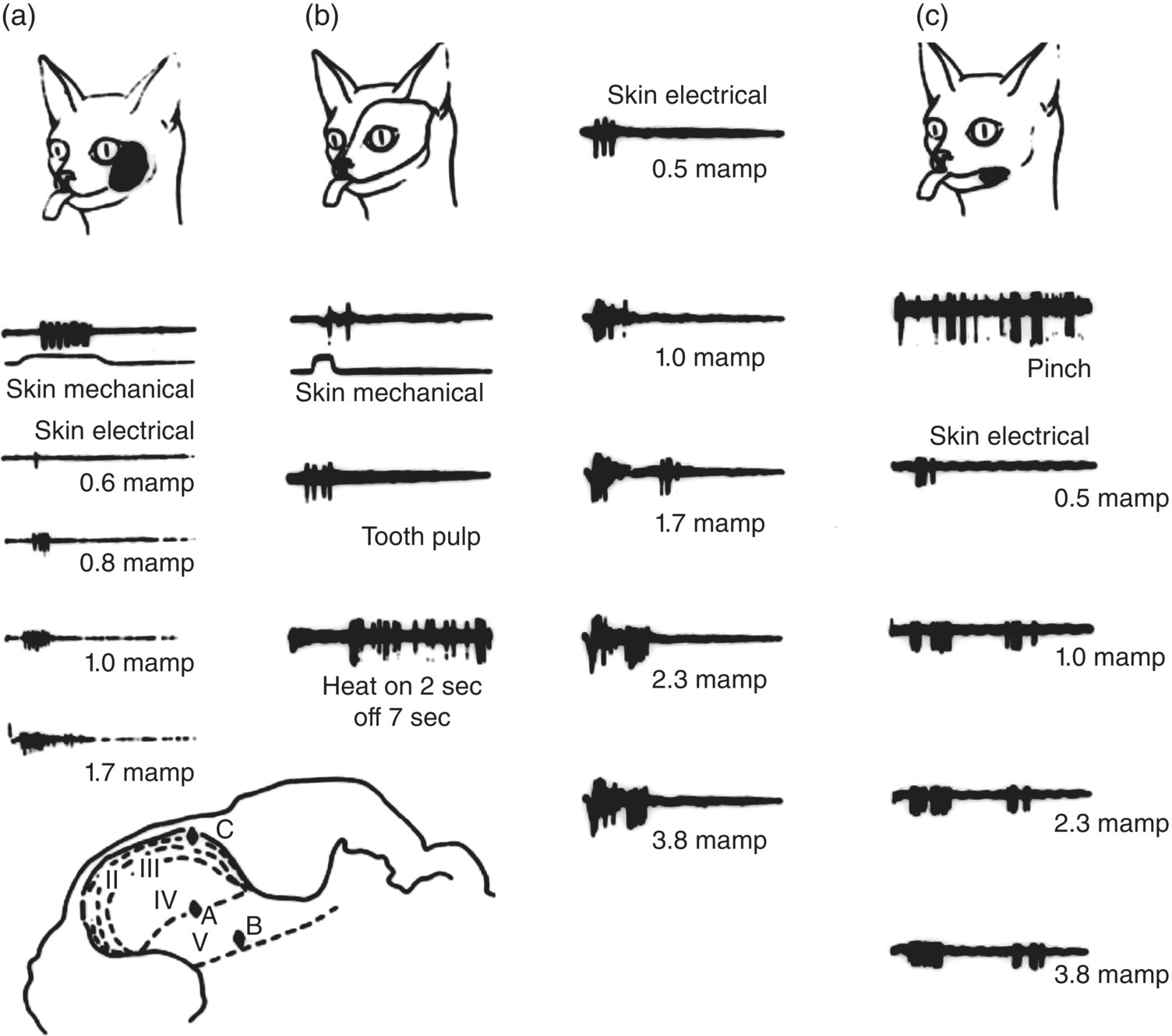
Figure 12.4 Examples of the three major classes of neurons in the trigeminal subnucleus caudalis. Data were derived from electrophysiological recordings in the subnucleus caudalis of a cat. Records in (a) were obtained from a single low-threshold mechanosensitive neuron that could only be activated by light mechanical (tactile) stimulation of the area of facial skin outlined (i.e., the receptive field of the neuron). Note its progressively increasing discharge to mechanical stimulation of the receptive field to increasing intensity levels of electric stimulation of the receptive field; even at high intensities, only a single burst of impulses can be produced. This neuron in (a) was located in layer IV of the subnucleus caudalis, as illustrated below in the cross-section of the medulla. The wide-dynamic-range neuron shown in (b) could be activated by tactile stimulation of its receptive field (outlined) as well as by electric stimulation of the canine tooth pulp, pinch (not shown), and noxious levels of radiant heat applied to the receptive field. Note that with increasing intensity levels of electric stimulation of the receptive field, late as well as early bursts of impulses could be evoked; the late discharge probably reflects inputs from nociceptive afferent fibers (e.g., A-δ and C), and the early burst reflects inputs from faster-conducting mechanosensory afferent fibers (e.g., A-β). This neuron was located in layer V of the subnucleus caudalis. The nociceptive-specific neuron shown in (c) was located in layer I of the subnucleus caudalis and could only be excited by noxious stimulation (e.g., pinch) of the receptive field. The first burst of impulses produced by high-intensity electric stimulation probably reflects inputs from small myelinated (A-δ) nociceptive afferent fibers, and the later burst reflects inputs from the slower-conducting unmyelinated (C) nociceptive afferent fibers. Time duration of records: (a), 50 msec; (b), 100 msec (except heat record: 10 sec); (c), 200 msec (except pinch record: 10 sec). Record to pinch in (c) is at twice the amplification for skin electrical records.
(Modified from Sessle, 1981a.)
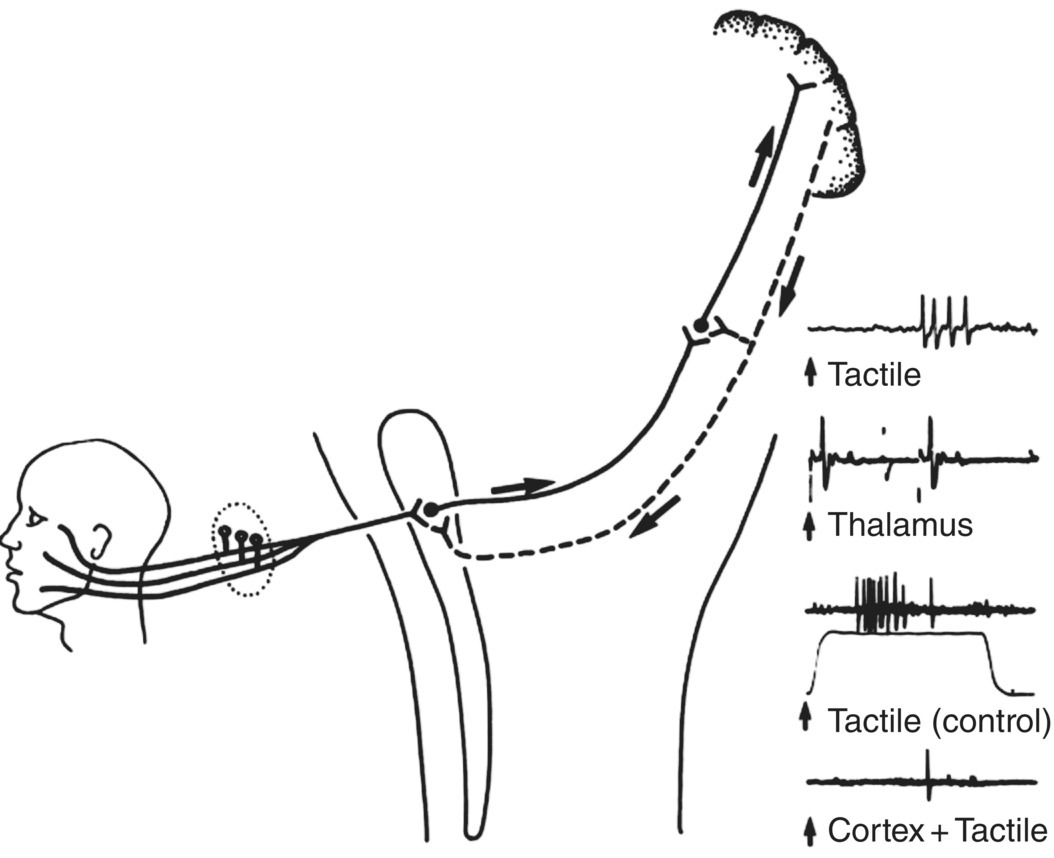
Figure 12.5 Influences from somatosensory cerebral cortex on sensory transmission in the trigeminal system. Tactile information from the face and mouth is relayed to the cerebral cortex via the trigeminal brainstem nuclei and thalamus. Many cortical neurons receiving and processing this information project directly down to the thalamus and brainstem and may modulate sensory transmission in the brainstem and thalamic neurons. Records on the right show such an example of a neuron recorded in the trigeminal brainstem sensory nuclear complex of a cat. The neuron could be activated by mechanical (tactile) stimulation of a localized receptive field on the face. It projected directly to the contralateral ventrobasal thalamus since it could be antidromically excited (viz., backfired) by an electric stimulus delivered to the thalamus, as shown. Note that if the facial tactile stimulus (the electronic analog of which is shown below the third trace) was delivered around the same time as an electric stimulus to the somatosensory cerebral cortex, the neuron’s responses to a series of tactile stimuli were markedly depressed. This is evidence of a rapidly conducting feedback loop from the cortex that may depress or limit trigeminal sensory transmission.
(Modified from Sessle, 1981b.)
Thermosensation
General features
The ability to sense the temperature of an object or substance is particularly well developed in the orofacial region. Thermal changes of less than 1 °C can be readily detected and discriminated, although temperature detection and discrimination can vary depending on the area of thermal stimulation, the magnitude and rate of the thermal changes, whether or not the area has undergone previous thermal changes, and the adapting temperature of the skin or oral mucosa being stimulated.
Peripheral processes
The receptors for temperature change are called thermoreceptors, which are activated by a small thermal change in either a cooling (cold receptors) or warming (warm receptors) direction; the former are more common than the latter. They are associated with some of the small-diameter, slow-conducting afferent fibers that are either myelinated (e.g., A-δ afferents) or unmyelinated (C-fiber afferents) and that provide the brain with accurate information on the location, magnitude, and rate of the temperature change.
Central pathways and processes
The main relay site in the brainstem of the afferent signals carried in the orofacial thermoreceptive primary afferent fibers is the trigeminal subnucleus caudalis. Some caudalis neurons are exclusively activated by thermal stimulation of localized parts of the face and mouth, and this thermal-related information is relayed from these caudalis neurons to the contralateral thalamus and then to the somatosensory cerebral cortex. The properties of peripheral and central neural elements underlying our thermal sensibility fit well with the specificity theory.
Taste
Only three aspects of taste are briefly mentioned here since taste is comprehensively covered in Chapter 10. First, like pain (see below), taste has cognitive, affective, and motivational dimensions as well as a sensory-discriminative dimension. For example, some tastes are pleasurable and we are motivated to seek them out, whereas other tastes have the opposite effect. In fact, humans have innate as well as acquired taste preferences, and the food industry is well aware of our inborn preference for sweetness (our “sweet tooth”). Second, taste sensibility is now known not to be confined to specific areas of the tongue; extralingual (e.g., palatal) taste buds may also make an important contribution to our taste. Third, several factors may modify taste, e.g., decreased saliva, poor oral hygiene, wearing of dentures, local anesthetics, plant extracts, other sensory experiences (such as smell). In addition, genetic, metabolic, and endocrine factors and the age of the individual may also influence taste sensibility.
Pain
General characteristics
Pain can cause great human suffering and represents a major economic burden on society through healthcare costs, time lost from work, etc. Moreover, in the case of orofacial pain conditions, they are very common (e.g., toothaches, headaches, TMD) and are often chronic and disabling.
Pain is now conceptualized as a multidimensional experience that includes a sensory-discriminative component that allows us to discriminate the quality, location, intensity, and duration of a noxious stimulus (i.e., a tissue-damaging stimulus). However, pain also encompasses cognitive, motivational, and affective (emotional) dimensions that can vary from individual to individual and can modify a person’s response to the stimulus. Thus, the pain a person experiences can depend not only on the magnitude of the noxious stimulus but also on factors such as the meaning to the person of the situation in which the pain occurs, the person’s emotional state and motivation to rid themselves of the pain, and even racial and cultural background and gender. A clinical consequence of this multifactorial nature of pain is that it can complicate diagnosis and treatment of pain for the clinician. It also makes the experimental study of pain exceedingly difficult.
Concepts of pain
The neural basis of pain is still only partly understood, but considerable advances have been made in the last few years. These insights into pain and especially its mechanisms have to a large extent come from studies of animals. The classic concept for explaining pain and the other somatic sensations is the specificity theory (Fig. 12.2). While it seems to explain other sensations such as touch (see above), specificity theory has been shown to have a number of limitations in trying to explain pain on the basis of a specific peripheral and central system. Consequently other theories have been proposed to account for the complexity and multidimensionality of pain, including the gate control theory of pain, which has attracted the most interest and research in the past 50 years (Fig. 12.2). Although it has its own limitations, it nonetheless provides a good conceptual framework for considering the multifactorial nature of pain. A major tenet of the gate control theory is its emphasis on the sensory interaction that occurs within the brain between the tactile-related neural signals carried into the brain by the large-diameter, low-threshold primary afferent fibers (principally A-β afferents, see above), and those signals conveyed by the small-diameter, high-threshold afferent fibers (some A-δ and C fiber afferents). The peripheral terminals of many of the latter fibers have a high threshold and are activated only by noxious stimuli. If, as a result of this interaction, the activity in these nociceptive afferent fibers prevails, central “pain-transmission” neurons are excited (the “gate” opens) and bring into action the central processes related to the perception of and reactions to noxious stimulation. The theory also emphasizes descending central neural controls (i.e., emanating from higher brain centers) related to the cognitive, affective, and motivational dimensions of pain that through their influences on the central transmission neurons can modulate the gate for pain.
Peripheral processes
Some nociceptive primary afferent fibers are specifically sensitive to noxious stimuli, whereas others respond to innocuous stimuli as well. The nociceptive afferents are small in diameter and slow conducting (A-δ and C fiber afferents), and they terminate in the peripheral tissues as free nerve endings, termed nociceptors. Injury or inflammation of orofacial tissues not only activates some of the numerous nociceptive afferent endings in the tissues, for example, tooth pulp afferents (Fig. 12.6), but may also induce an increased excitability of these endings and adjacent nociceptive endings. This peripheral sensitization is important in the increased pain sensitivity that can be detected clinically at a peripheral injury or inflammation site (e.g., as in arthritis, myositis, and pulpitis). The increased pain sensitivity may be reflected as an exaggerated perceptual response to noxious stimuli (hyperalgesia) or as a pain response to a stimulus (e.g., tactile) that is normally innocuous (allodynia), and the involvement of adjacent afferent endings beyond the initial injury site is a peripheral process contributing to the spread of pain in these tissues (Table 12.1). Several chemical mediators, including some normally associated with actions within the central nervous system (CNS) (e.g., excitatory amino acids such as glutamate; opioids), are involved in activating the nociceptive afferent endings or in producing or modifying peripheral sensitization by interacting with ion channels or membrane receptors on the nociceptive afferent endings (Fig. 12.7). Some of these ion channels and membrane receptors are activated by noxious mechanical and thermal stimuli (e.g., the TRP channels described in Chapter 9). In addition, non-neural processes, such as satellite glial cells in the trigeminal ganglion and immune cells in peripheral tissues such as the tooth pulp, may modulate the nociceptive afferent excitability in these tissues. Also noteworthy is the documentation of sex differences in some of these peripheral processes that may account at least in part for the sex differences in the prevalence of a number of orofacial pain states. Moreover, some drugs that are commonly used to relieve orofacial pain (e.g., aspirin) may exert their analgesic action by interfering with some of these peripheral mechanisms.
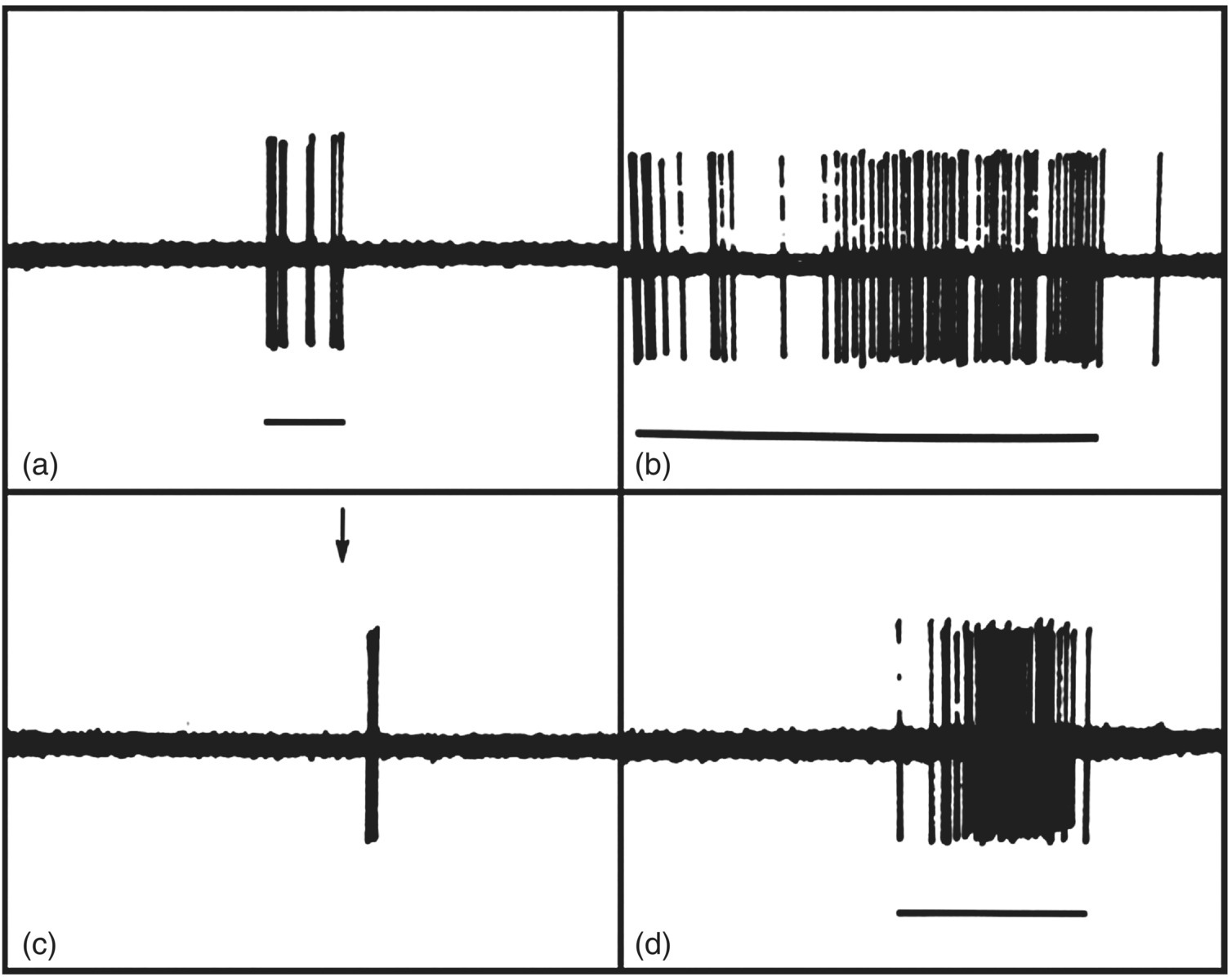
Figure 12.6 Responses of tooth pulp afferents recorded in the inferior alveolar nerve of a dog. Note that stimuli that cause pain when applied to human teeth could excite the pulp afferent(s), as shown by its response to (a) mechanical probing of dentin of the tooth supplied by the afferent as well as to (b) an air blast, (c) hypertonic calcium chloride (arrow), and (d) drilling applied to the dentin. Time calibration: 1 sec.
(From Narhi et al. (1982) Archives of Oral Biology, 27, 1053–1058).
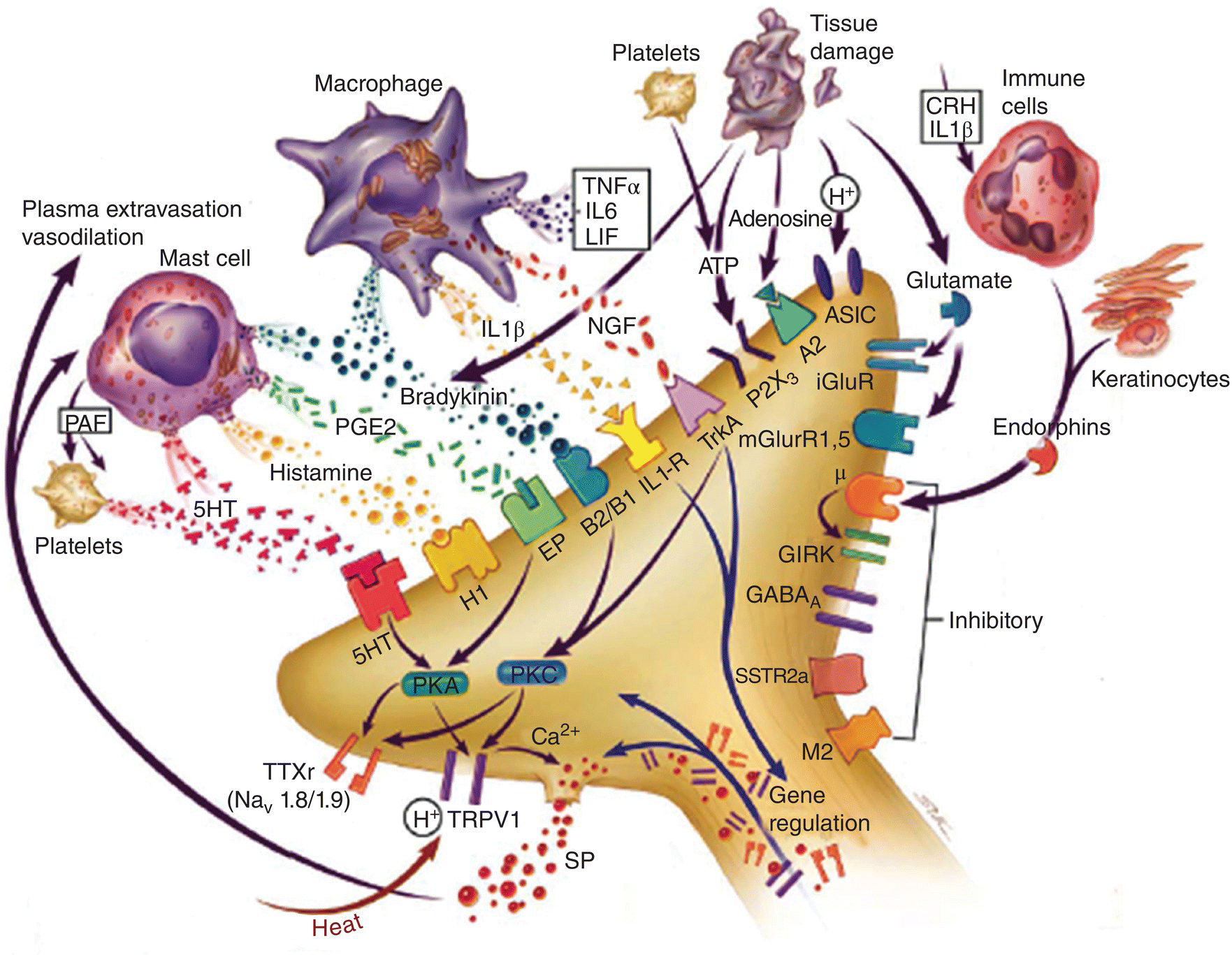
Figure 12.7 Mediators in orofacial tissues involved in peripheral sensitization following inflammation. Inflammation leads to numerous chemicals being released from macrophages, mast cells, immune cells, and injured cells that act on ion channels or membrane receptors on peripheral nociceptive afferent nerve endings and thereby may alter the sensitivity of the endings. Some of these mediators can increase the excitability of the nociceptive afferent endings (i.e., peripheral sensitization); others may exert inhibitory effects. Several of these mediators are shown. ASIC, acid-sensing ion channel; CRH, corticotrophin-releasing hormone; GIRK, G protein coupled inward rectifying potassium channel; 5-HT, serotonin; iGluR, ionotropic glutamate receptor; IL-1β, interleukin-1-beta; IL-6, interleukin-6; LIF, leukemia inhibitory factor; μ, mu-opioid receptor; M2, muscarinic receptor; mGluR, metabotropic glutamate receptor; NGF, nerve growth factor; PAF, platelet-activating factor; PGE2, prostaglandin E2; PKA, protein kinase A; PKC, protein kinase C; SSTR2A, somatostatin receptor 2A; TNF-α, tumor necrosis factor alpha; TrkA, tyrosine kinase receptor A; TRPV1, transient receptor potential vanilloid 1; TTXr, tetrodotoxin-resistant sodium channel.
(From Meyer et al., 2006.)
Table 12.1 Relationship of parameters of peripheral sensitization of a nociceptive afferent to sensory perceptual correlates of pain.
Peripheral Sensitization | Sensory Perception |
Decreased activation threshold | Allodynia |
Increased responsiveness to noxious stimuli | Hyperalgesia |
Involvement of adjacent nociceptive afferent endings | Pain spread |
Central pathways and processes
The nociceptive primary afferent fibers supplying the face and mouth project via the trigeminal ganglion to the trigeminal brainstem sensory nuclear complex, especially to the subnucleus caudalis (see Fig. 12.3). Here they release chemical mediators that are synthesized in the primary afferent trigeminal ganglion cell bodies and transported to their peripheral (in orofacial tissues) and central (in the brainstem) endings; these include glutamate and the neuropeptide substance P, which activate the neurons by acting, respectively, on glutamatergic and neurokinin receptors on the neurons. Many caudalis neurons receive the signals from orofacial nociceptive primary afferents and, thus, can respond to noxious stimulation of the face and mouth (including the teeth), TMJ, or jaw and tongue muscles. There are two types of these neurons: nociceptive-specific neurons that respond exclusively to noxious stimuli, and wide-dynamic-range neurons that respond to innocuous as well as noxious stimuli (e.g., Fig. 12.4b, 12.4c). These two types of neurons relay nociceptive information to neurons in the contralateral thalamus, from where it is relayed from analogous nociceptive-specific and wide-dynamic-range neurons to the overlying cerebral cortex or other thalamic regions. While parts of the thalamus or cortex are involved in the various components of pain behavior (perception, motivation, etc.), the precise function of each region is still not fully understood. Also unclear is the function of the rostral components of the trigeminal brainstem complex. While they are especially concerned with the relay of tactile information (see above), they also may play a role in orofacial pain mechanisms. For example, afferent fibers from the tooth pulp, generally assumed to represent a nociceptive input, synapse with neurons present not only in the subnucleus caudalis but also in the more rostral components of the complex.
Control of pain
The procedures available for the control of pain are numerous (Table 12.2), ranging from pharmacologic approaches such as local and general anesthetics and analgesic drugs (e.g., aspirin, morphine) to therapeutic procedures such as acupuncture, transcutaneous electric stimulation (TENS), cognitive behavioral therapy, hypnosis, and psychiatric counseling; neurosurgical procedures are also are available for severe pain conditions unrelieved by the other approaches. These various procedures are aimed at blocking pain signals either in peripheral tissues (e.g., aspirin), before nerve impulses enter the brain (e.g., local anesthetics), or within the brain (e.g., general anesthetics and many analgesic drugs).
Table 12.2 Various methods used for management of orofacial pain
Pharmacological methods | Psychological methods | Physical methods |
Local anesthetics Nonopioid analgesics
Steroids |
Information Education Counseling Biofeedback Stress Management Relaxation Hypnosis Cognitive-behavioral therapy Psychotherapy |
Jaw exercises Stretch therapy Massage Heat/cold Ultrasound Soft laser Acupuncture TENS Oral splints |
As mentioned above, the trigeminal brainstem sensory nuclei have a complex structural organization by which their neurons can interact in complex ways with many other parts of the CNS. These interactions involve inhibitory processes that modulate nociceptive transmission and that are widespread in the brain. Some of these processes also are involved, as noted above, in modifying touch as well as reflex activity and more complex behavioral functions (see “Sensorimotor Functions and Motor Control”). Inhibitory modulation of nociceptive transmission in the trigeminal and spinal systems can occur through (1) sensory interaction and (2) descending central control mechanisms, as postulated by the gate theory of pain (see above). For example, the activity of trigeminal nociceptive neurons can be markedly suppressed by large fiber afferent inputs activated by tactile stimulation of orofacial tissues (i.e., sensory interaction); in some situations, even small-fiber nociceptive afferent stimulation may also suppress their activity. In addition, there are descending central controls, e.g., stimulation of brain sites such as the midbrain periaqueductal gray matter and the nucleus raphe magnus in the lower brainstem (Fig. 12.8) that descend in the CNS and produce inhibition of the nociceptive neurons and can have marked analgesic effects in humans and experimental animals.
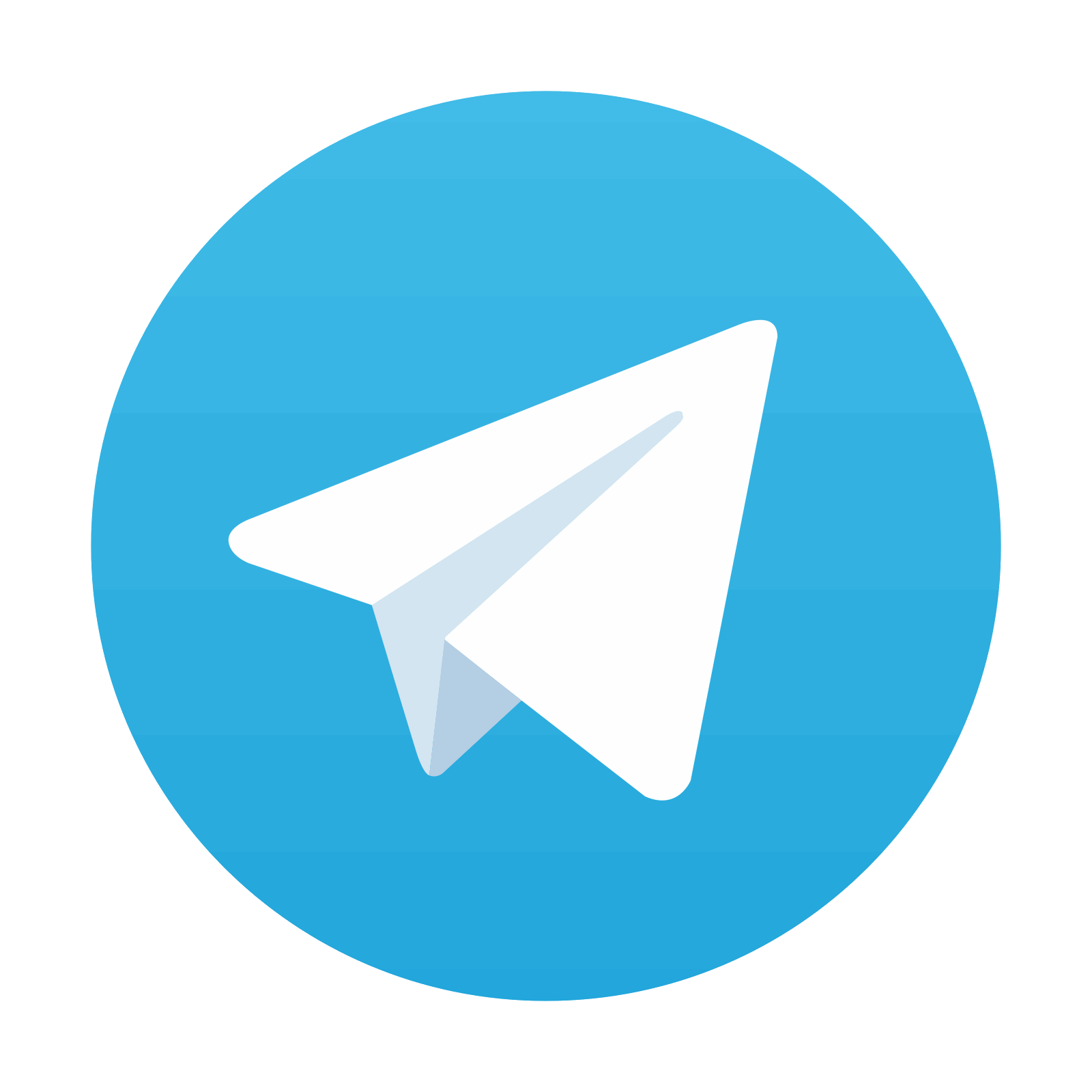
Stay updated, free dental videos. Join our Telegram channel

VIDEdental - Online dental courses
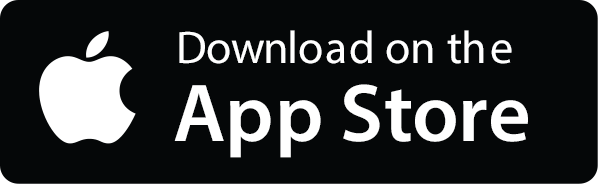
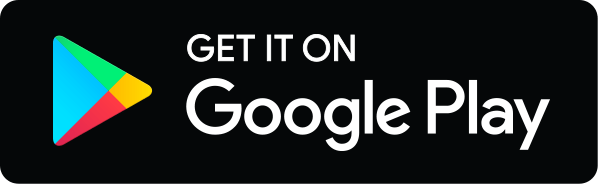